Einstein's Theory: Foundations and Implications
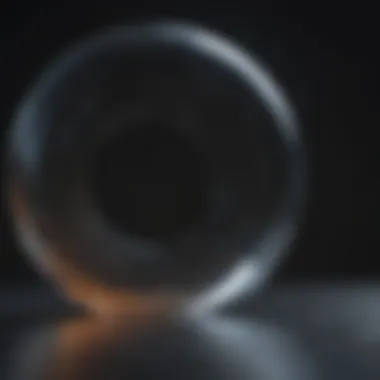
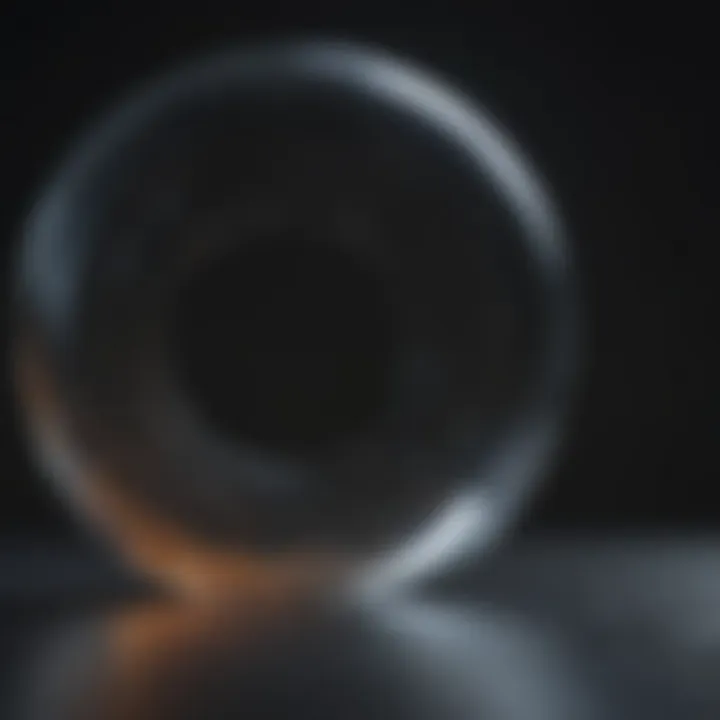
Intro
Albert Einstein stands as a towering figure in the landscape of modern physics, shaping our understanding of space, time, and the fabric of the universe itself. His theories of relativity—both special and general—have redefined the bedrock of scientific inquiry, challenging preconceived notions and opening vast realms of exploration. This piece aims to break down these intricate theories and examine their foundations, implications, and significance in today’s scientific context.
In Special Relativity, Einstein introduced a new paradigm where the laws of physics remain constant for all observers, regardless of their relative motion. This theory fundamentally shifts how we perceive time and space, suggesting they are not fixed entities but rather interwoven constructs that can stretch and compress under various conditions.
The General Theory of Relativity builds upon this foundation, presenting gravity not as a mere force but as a curvature of spacetime caused by mass. This concept has invigorated fields such as cosmology and astrophysics, leading to revolutionary insights about phenomena like black holes and the universe's expansion.
Beyond the mathematical equations and experimental validations, the philosophical implications of these theories force us to grapple with profound questions about reality. With the continuous relevance of Einstein's ideas, particularly in correlations with quantum mechanics, this narrative seeks to unfold the legacy and ongoing influence of his work within modern science.
By dissecting each element of Einstein's theories, we gain not just insight into scientific principles but also a deeper appreciation for the very nature of the universe. Join us as we examine the key concepts central to his theories, explore current research trends, and ultimately reflect on how Einstein's insights have shaped our understanding today.
Overview of Einstein's Contribution to Physics
Einstein's contribution to physics marks a significant turning point in our understanding of the universe. His theories, particularly the special and general theories of relativity, transformed long-held notions about space, time, and gravity. The importance of this topic lies not just in the groundbreaking scientific principles that Einstein introduced but also in the profound implications these theories have on both contemporary science and everyday life.
A Prelude to Modern Physics
Before Einstein emerged onto the scientific scene, physics was predominantly dominated by classical mechanics, largely shaped by Newtonian principles. Scientists were operating under the assumption that time and space were absolute entities. However, Einstein's revolutionary ideas peeled back the layers of these assumptions. His special theory of relativity, published in 1905, tackled the interconnectedness of space and time, suggesting that they are relative and interconnected. This concept challenged the views of his predecessors and laid the framework for modern physics.
Essentially, Einstein was not just another theorist; he was a harbinger for a new way of thinking. The implications of his theories reached far beyond academic circles. People started to reconsider their interpretation of the physical world and the very mechanics of reality itself. As a result, Einstein's work spurred a new era of exploration and inquiry in science, prompting physicists to look at the universe with fresh eyes.
Historical Context
To fully appreciate Einstein's contributions, understanding the historical context of his work is crucial. At the beginning of the twentieth century, the scientific community was rife with debates about the nature of light, electromagnetism, and thermodynamics. The classical theories were being questioned, sparking curiosity and frustration among physicists.
In the realms of electromagnetic theory, physicists like James Clerk Maxwell had already shown how light is an electromagnetic wave. Yet, the existing models couldn't satisfactorily explain the behavior of light in moving frames of reference—most notably in relation to the speed of light. This is where Einstein stepped in, offering simple yet profound postulates that would forever change the landscape of physics.
Einstein not only picked up the existing threads of knowledge; he masterfully wove them together to create a new tapestry of understanding. His reflections on simultaneity, motion, and the speed of light were revolutionary. They allowed scientists to break free from the limitations imposed by classical thinking.
"The important thing is not to stop questioning. Curiosity has its own reason for existence." - Albert Einstein
"The important thing is not to stop questioning. Curiosity has its own reason for existence." - Albert Einstein
Thus, Einstein's insights came at a pivotal moment in scientific history, serving as the launching pad for quantum mechanics, cosmology, and beyond.
The Special Theory of Relativity
The Special Theory of Relativity represents a cornerstone of modern physics, fundamentally reshaping our perception of time and space. Proposed by Albert Einstein in 1905, it introduced revolutionary concepts that challenge traditional views held since Newton's time. This theory is unique in its forward-thinking approach, capturing attention not only for its groundbreaking principles but also for its far-reaching implications in both theoretical and practical realms. With its core tenets, it lays the groundwork for understanding the universe in ways that still resonate in present-day science and technology.
Fundamental Postulates
Einstein's Special Theory of Relativity is underpinned by two crucial postulates. The first asserts that the laws of physics are the same for all observers, regardless of their relative motion. In simpler terms, whether you're sitting on your porch or zooming down the highway, the rules governing everything from gravity to electromagnetism do not change. The second postulate contends that the speed of light in a vacuum is constant, irrespective of the motion of light sources or observers. This means that no matter how fast you're traveling, the speed of light remains unchanged, acting as a cosmic speed limit that even the fastest spaceship cannot surpass.
These foundational ideas are not merely theoretical assertions; they provoke significant questions about existence itself. They prompt us to think, "What does it mean to observe reality if different observers experience it differently?"
Implications of Time Dilation
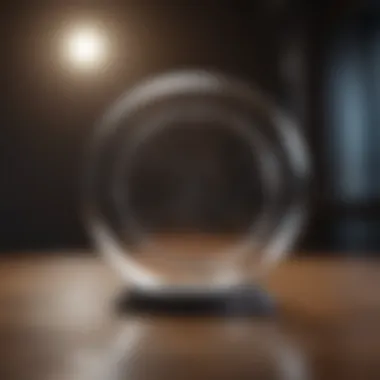
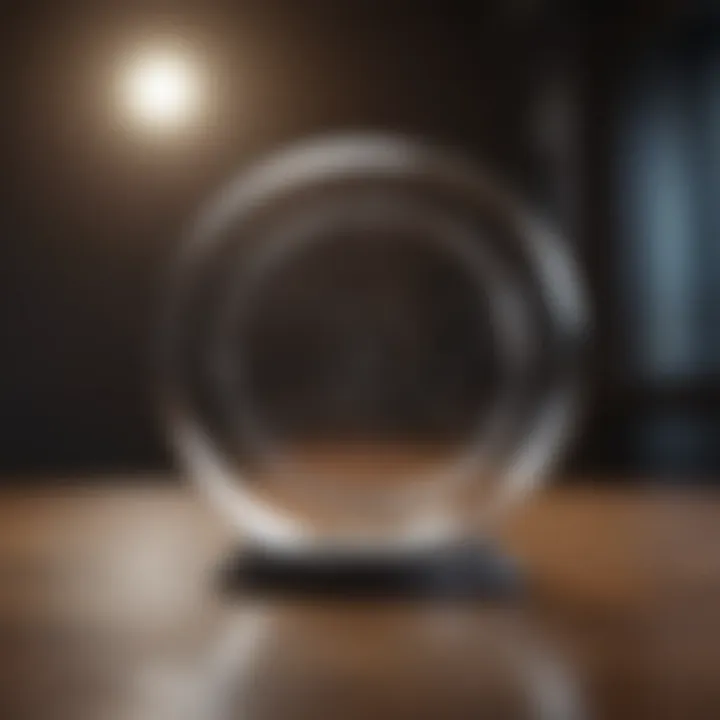
One of the more profound consequences arising from the theory is time dilation, suggesting that time itself slows down for objects in motion relative to a stationary observer. This isn’t just abstract mumbo jumbo; time dilation has real-world consequences. For instance, think of astronauts aboard the International Space Station. They experience time slightly slower than people on Earth's surface due to the high velocities at which they travel. The faster an object goes, the more pronounced the effect.
Imagine two identical twins, where one ventures into space at near light speed while the other stays on Earth. Upon the traveler’s return, they would find that they aged less than their sibling. This phenomenon blurs the lines between past and future, emphasizing the relativity of experience in ways that were previously inconceivable.
Length Contraction Explained
Just like time can stretch, so too can space bend. This aspect, often called length contraction, indicates that objects moving at speeds close to the speed of light will appear shorter in the direction of their motion compared to stationary observers. Picture a train hurtling down a track—if it were moving at a significant fraction of the speed of light, an observer standing still would measure it to be shorter than what the train passengers would perceive.
This contraction only becomes notable in extreme velocities, unlike anything we encounter in our day-to-day lives. It’s one of those peculiar phenomena that make you question reality itself—after all, how can something be perceived differently by two separate observers?
The Equivalence of Mass and Energy
One of the milestones of the Special Theory of Relativity is encapsulated in the famous equation: E=mc². This formula conveys the equivalency of mass and energy, suggesting that mass can be converted into energy and vice versa. What does this mean practically? For starters, it implies that a small amount of mass could yield a substantial release of energy, an idea that lies at the heart of nuclear reactions.
Moreover, this profound connection between matter and energy has paved the way for advancements in diverse fields, from power generation to cosmology. It illustrates how everything in the universe is interconnected—no piece of matter exists in isolation; rather, each is a reflection of energy in motion.
Experimental Confirmations
The theoretical claims of the Special Theory of Relativity have not only survived rigorous scrutiny but have been validated through numerous experiments. From particle accelerator observations, where particles exhibit both time dilation and mass-energy equivalence, to the precise clocks used in GPS satellites, the evidence supporting Einstein’s theory is irrefutable.
In one notable experiment, scientists have managed to measure the expected time dilation effects on fast-moving particles, finding results that match Einstein’s predictions almost perfectly. This is why, when we navigate using GPS, we must factor in these relativistic effects, ensuring our technology operates accurately in the modern age.
Einstein's theories were not mere speculation; they have been tested and confirmed across various scientific landscapes, showcasing their importance in understanding our universe.
Einstein's theories were not mere speculation; they have been tested and confirmed across various scientific landscapes, showcasing their importance in understanding our universe.
In summary, the Special Theory of Relativity initiates an enthralling journey into the nature of reality, challenging perceptions and demonstrating the intricate dance between space, time, mass, and energy. With this foundation set, it propels scholars and enthusiasts alike to explore deeper layers of the cosmos and its underlying laws.
The General Theory of Relativity
The General Theory of Relativity, published by Albert Einstein in 1915, represents a monumental leap in our understanding of the universe. At its core, this theory extends the principles outlined in the Special Theory of Relativity, introducing the concept that gravity is not merely a force but rather a curvature of spacetime. This perspective has profound implications for physics, challenging long-held beliefs and paving the way for numerous advancements in both theoretical and experimental domains.
Not only does it redefine gravity, but it also invites a reevaluation of our understanding of phenomena like time and space itself. The theory emphasizes the dynamic nature of these elements, evoking a view of the universe that is ever in flux and inherently interconnected.
Concept of Gravitational Curvature
The concept of gravitational curvature is vital in understanding Einstein’s approach to gravity. Unlike Newton's view, which treated gravity as a force acting at a distance, Einstein suggested that mass causes the fabric of spacetime to curve. Picture a heavy object like a bowling ball placed on a trampoline; the surface sags around it, demonstrating how the presence of mass alters the spatial area around it.
When smaller objects, such as marbles, roll nearby, they follow paths determined by this curvature, not because they are directly pulled, but because they are following the contours created by the bowling ball’s weight. Thus, planets and stars move in curved paths dictated by the warping of spacetime.
"Mass and energy determine the geometry of spacetime, and conversely, the geometry of spacetime tells mass how to move."
"Mass and energy determine the geometry of spacetime, and conversely, the geometry of spacetime tells mass how to move."
Mathematical Framework and Tools
The mathematical foundation for General Relativity is steeped in differential geometry, a branch of mathematics that deals with curves and surfaces. Einstein formulated his theory using the Einstein Field Equations, a set of ten interrelated differential equations. These equations are pivotal as they describe how matter and energy in the universe influence the curvature of spacetime.
One of the primary tools involved is the metric tensor, which encapsulates information about the distances and angles within spacetime.
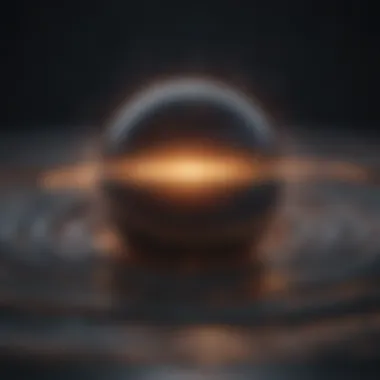
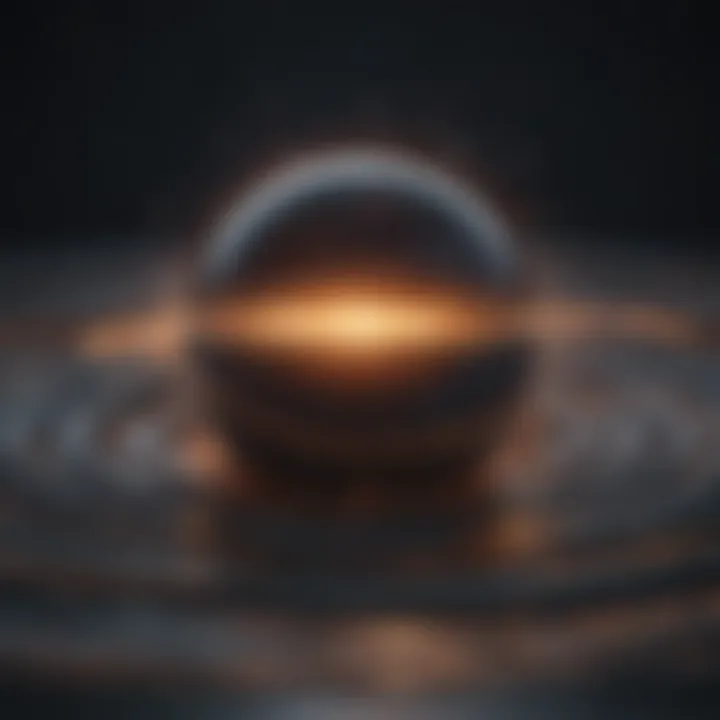
- Key elements of the mathematical framework include:
- The Ricci curvature tensor, which helps measure the degree of curvature of spacetime.
- The stress-energy tensor, which relates energy density and momentum to curvature.
This intricate mathematical structure enables predictions about the behavior of gravitational fields, laying the groundwork for future explorations in astrophysics and cosmology.
Black Holes and Singularities
One of the most fascinating predictions of the General Theory of Relativity pertains to black holes—regions in spacetime where gravity is so fierce that nothing, not even light, can escape.
Einstein's equations imply the presence of singularities, points in spacetime where the curvature becomes infinite, and the classical laws of physics cease to apply. These phenomena challenge our understanding and raise tantalizing questions about the nature of the universe. The existence of black holes was later confirmed through astronomical observation, leading to monumental breakthroughs in the field of astrophysics.
Cosmological Implications
The ramifications of General Relativity extend to cosmology, fundamentally altering our understanding of the universe's structure and evolution. It posits that the universe is not static; rather, it is expanding, a notion further substantiated by Edwin Hubble’s observations of distant galaxies.
Moreover, concepts such as the Big Bang and the subsequent cosmic evolution are rooted in the principles of General Relativity, providing a framework for explaining the large-scale structure of the cosmos. Ideas like dark energy and dark matter have emerged to describe phenomena that cannot be understood solely through traditional physics, establishing Einstein's work as a cornerstone of modern cosmology.
Testing General Relativity
The validity of the General Theory of Relativity has been rigorously tested over the years through various experiments and observations. One notable instance is the observation of light bending around massive objects—this phenomenon, called gravitational lensing, corroborates Einstein’s predictions.
Other tests include measuring the rate at which time changes in strong gravitational fields, known as gravitational time dilation, and observing the effects of gravity on the orbit of planets. Each successful test has reinforced its status as a cornerstone of theoretical physics.
- Some significant experiments include:
- The 1919 solar eclipse observations by Arthur Eddington.
- The precise measurements of the orbit of Mercury.
Through these varied channels, General Relativity continues to be not just a theoretical framework but an essential part of contemporary physics, influencing everything from satellite technology to our understanding of the universe's ultimate fate.
Philosophical Considerations
The exploration of Einstein's theories opens a dialogue not just within the realm of physics, but extends into the philosophical dimensions of existence and knowledge. This section examines how Einstein's groundbreaking ideas compel us to reconsider our understanding of reality, perception, and the nature of the universe. Einstein's contributions push us to question both our scientific frameworks and philosophical assumptions, bridging the chasm between empirical evidence and abstract thought.
Reality and Perception in Physics
One of the significant philosophical implications arising from Einstein's work is the relationship between reality and perception. Traditionally, physics emerged from the notion that there exists an objective reality, independent of human observation. However, Einstein introduced a level of subjectivity through his relativity theories.
Consider this: time and space, according to Einstein, are not absolutes but can be shaped by velocity and gravity. This means that observers in different frames of reference might experience time and space differently. For example, if you’re aboard a fast-moving spaceship, time passes differently for you than for someone standing still on Earth.
This leads to a fundamental question: if our perceptions can alter our experience of reality, how do we define what is "real"? As Einstein once suggested,
"Reality is merely an illusion, albeit a very persistent one."
"Reality is merely an illusion, albeit a very persistent one."
In this light, perception doesn’t just influence our understanding but becomes a vital component in our grasp of the physical world. Through the lens of relativity, we see that scientific inquiry must also consider subjective experiences, challenging the idea of a fixed reality and making way for a more nuanced view of existence.
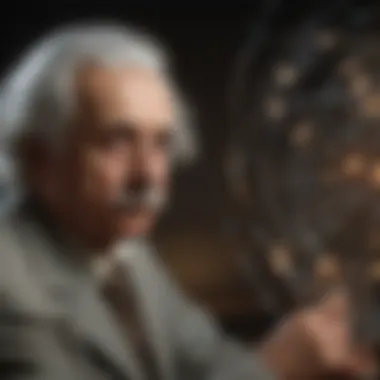
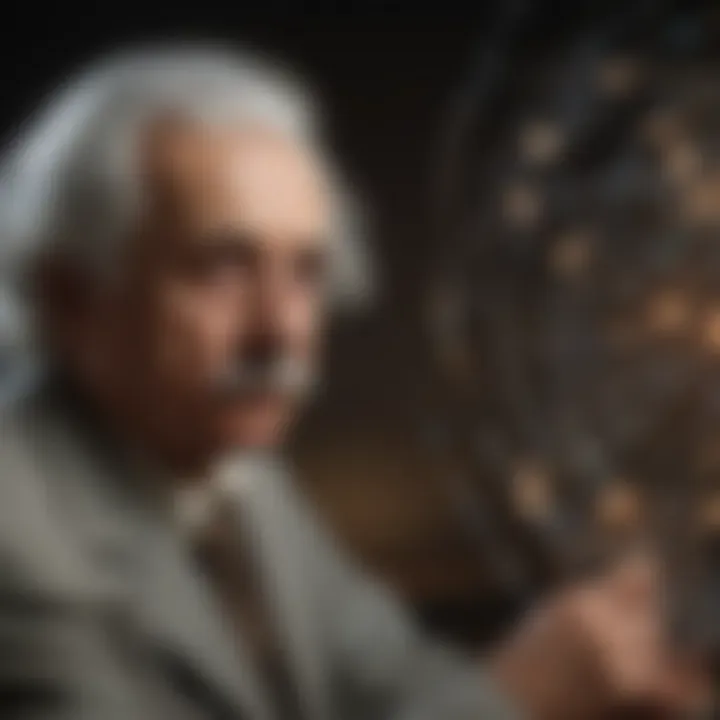
Determinism Versus Probabilism
Another philosophical consideration that arises from Einstein's body of work involves the tension between determinism and probabilism in understanding the universe. Determinism suggests that every event is predetermined by preceding causes, implying a set path through which events unfold. This was an idea that Einstein grappled with, especially in the context of quantum mechanics.
On the other hand, probabilism introduces chance and randomness into physical laws. The famous Einstein quote, "God does not play dice with the universe," reflects his discomfort with the notion of an unpredictable universe governed by probabilities. He preferred a deterministic approach, where things followed clear and predictable laws.
However, the rise of quantum theory, particularly as championed by physicists like Niels Bohr, showed that at subatomic levels, behavior often defies deterministic laws. Particles can exist in multiple states until observed, challenging the predictability Einstein held dear.
This philosophical conflict raises profound questions about freedom of choice and the nature of causality. Are we mere puppets tangled in strings of cause and effect, or do we have a role in shaping outcomes? The dialogue between determinism and probabilism remains crucial not only for physics but for our understanding of existence itself.
Einstein's theories thus foster ongoing contemplation around these philosophical notions, emphasizing that science is as much about questions as it is about answers. Understanding how we perceive reality and the degree to which it may or may not be predetermined continues to shape not only academic discourse but everyday thought.
Einstein's Legacy in Contemporary Science
Albert Einstein's theories have transformed the landscape of modern science. His ideas laid the groundwork for countless advancements, particularly in astrophysics and quantum mechanics. The relevance of his work is felt deeply in these fields; it shifts the paradigms of how we understand the universe. It’s not just the discoveries themselves that matter, but the methodologies and principles Einstein employed that continue to inspire generations of scientists.
Influence on Modern Astrophysics
Einstein's impact on astrophysics is monumental. The general theory of relativity revolutionized our understanding of gravity and space-time, providing a new framework that explained various cosmic phenomena. Concepts such as black holes and gravitational waves, which were once mere speculation, now stand as pillars in the field of astrophysics.
- Gravitational Waves: The detection of gravitational waves by LIGO in 2015 validated Einstein's predictions made over a century earlier. These ripples in space-time, caused by massive events like merging black holes, opened up a new avenue for exploring the cosmos.
- Black Holes: The notion that time and space can warp around a massive object has led to the understanding of black holes as regions of space where gravity is so strong that nothing can escape. They are now pivotal to astrophysical research, pushing the boundaries of what we know about the universe's structure and evolution.
- Cosmology: Einstein also contributed to the birth of modern cosmology with his introduction of the cosmological constant—meant to allow a static universe in his original formulation. This constant has evolved into the concept of dark energy, a crucial component in understanding the accelerating expansion of the universe today.
Interplay with Quantum Mechanics
The relationship between Einstein’s work and quantum mechanics is complex and, in some ways, paradoxical. While Einstein himself had reservations about the probabilistic nature of quantum theory, his contributions critically influenced the field.
- Photoelectric Effect: Einstein's explanation of the photoelectric effect provided pivotal evidence for quantum theory, showing that light can behave both as a wave and a particle. This duality is a cornerstone of quantum physics, influencing how particles are studied today.
- Wave-Particle Duality: Through his work, Einstein urged physicists to rethink the nature of light and particles, leading to significant discussions that shaped quantum mechanics.
- Uncertainty and Determinism: Einstein famously disagreed with the idea that quantum mechanics was inherently probabilistic, famously stating, "God does not play dice." This philosophical debate continues to spur discussions among scientists about the nature of reality and determinism in the quantum realm.
Einstein's legacy, then, is one of profound influence and innovation. His insights not only catalyzed extensive research and development in contemporary science but also laid the philosophical groundwork for ongoing inquiries into our universe's most fundamental questions.
Culmination: The Enduring Impact of Einstein's Theories
In understanding the vast landscape of physics, it's indispensable to recognize that Einstein's contributions serve as a cornerstone. His theories don't just fill pages in a textbook; they resonate through every corner of modern scientific thought and practice. The intertwining of his ideas with both theoretical and experimental physics sets the stage for a dialogue that pervades contemporary research.
Einstein’s theories reflect a paradigm shift in how the universe is perceived, going beyond mere equations and principles into realms that challenge our very understanding of reality. They urge us to think critically and reshuffle long-held beliefs. The implications of such work extend into multiple domains including cosmology, quantum mechanics, and even philosophy, leaving a legacy that continues to inspire and provoke inquiry.
Reflections on Scientific Progress
When we gaze back at the scientific progress sparked by Einstein, it’s hard not to appreciate the boldness of his ideas. His work cut across familiar territories and introduced concepts that at first seemed almost nonsensical, yet proved to be fundamentally true. His inclination to question conventional wisdom paved a path not just for his successors, but for an entire generation of scientists willing to explore the unknown.
In the domain of astrophysics for instance, his predictions about gravitational waves and black holes are but two examples of how a single individual's thought can ripple out to alter entire fields. The 2015 detection of gravitational waves by LIGO stands testament to this—an event that many would argue was the ultimate validation of Einstein's insights about the fabric of spacetime.
Educators and researchers alike continue to draw from Einstein's ethos, emphasizing critical thinking and the courage to challenge established norms. His legacy is manifest not only in academic achievements but in the broader cultural conversation surrounding scientific inquiry.
Future Directions in Physics
Even as we stand on the solid ground laid out by Einstein, questions continue to loom large regarding the universe's intricate workings. The intertwining of relativity, quantum mechanics, and cosmological phenomena paints a complex picture that beckons further investigation. New theories are emerging at the edges of scientific scholarship, and at the heart lies the question of unifying gravity with quantum mechanics.
Extraterrestrial exploration, dark matter investigations, and the search for a Theory of Everything all are future directions that imply an overarching quest for deeper understanding. Considerations about how the theories of relativity mesh with quantum field theories will shape the path forward for physicists aiming for a comprehensive depiction of physical reality.
"The pursuit of knowledge is an endless journey; we stand on the shoulders of giants, looking forward to new horizons."
"The pursuit of knowledge is an endless journey; we stand on the shoulders of giants, looking forward to new horizons."
In summation, the impact of Einstein’s theories will continue to endure and evolve. They challenge each new generation of scientists to push boundaries, seek truths, and uncover the mysteries that lay beyond our present understanding. Whether through experiments in particle physics or explorations of the cosmos, the intellectual legacy of Einstein remains a guiding star—ever-present and illuminating the way forward.