Exploring DNA: Techniques and Applications in Genetics
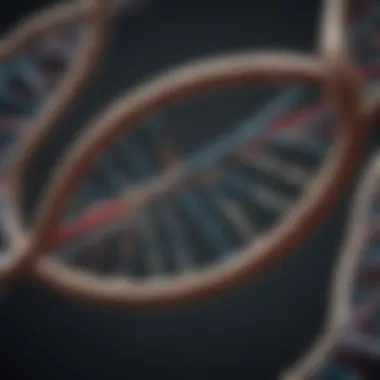
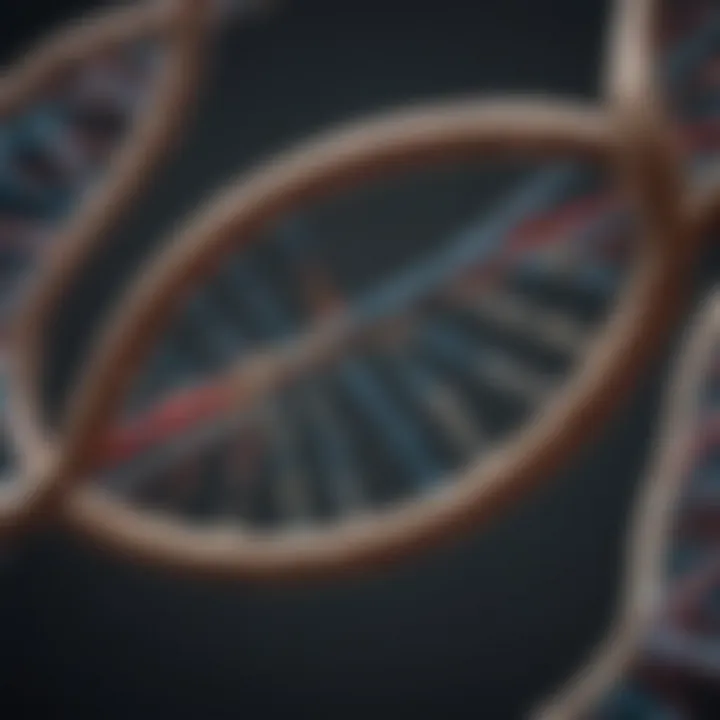
Intro
DNA, or deoxyribonucleic acid, serves as the building block of life, carrying the genetic blueprint required for the growth, development, functioning, and reproduction of all living organisms. As we embark on this journey through the intricate realms of DNA, we will explore its structure and function, revealing the underlying principles that govern its every role in genetics and biotechnology.
Understanding what DNA is—its double helix structure composed of nucleotides, which are further organized into genes—sets the stage for grasping its significance. Not merely a microscopic strand, DNA's impact resonates through various fields, from medicine that harnesses its power for treating genetic disorders to the forensic science that relies on DNA evidence to solve crimes.
With such breadth of influence, it's imperative to uncover how scientists study this vital molecule. There are numerous techniques designed to locate, analyze, and manipulate DNA. These include methods like PCR (Polymerase Chain Reaction) and sequencing technologies that have revolutionized the landscape of genetic research.
Moreover, the societal implications of DNA research are profound. As we navigate newer frontiers of genetic testing, privacy concerns arise alongside the ethical considerations of gene editing. This article aims to present a clear, structured overview of these complex issues, providing valuable insights for students, educators, and professionals alike.
Understanding DNA
The study of DNA is foundational to genetics and biotechnology. Understanding DNA illuminates the processes that dictate how traits are passed down through generations and how these traits can be analyzed, manipulated, and even utilized in a variety of fields. By comprehensively grasping the structure and function of DNA, readers can appreciate its central role in living organisms.
The Molecular Structure of DNA
Components of DNA
The basic building blocks of DNA are nucleotides, which consist of three essential components: a phosphate group, a sugar molecule (deoxyribose), and a nitrogenous base. Each of these elements plays a crucial role in the overall integrity and function of DNA. The sugar and phosphate form the backbone of the DNA strand, while the nitrogenous bases—adenine, thymine, cytosine, and guanine—interact in pairs (A-T and C-G) to create the genetic code.
One significant characteristic of these components is their stability. The deoxyribose sugar provides a backbone that can withstand various environmental factors that could otherwise damage genetic material. This stability is beneficial when we consider the longevity of DNA, which can remain intact for thousands of years in some cases. However, while the structure lends itself to resilience, it can also pose challenges in DNA analysis, especially when isolating ancient samples.
The Double Helix Model
The double helix model of DNA, first described by James Watson and Francis Crick, reveals how the strands of DNA coil around each other, resembling a twisted ladder. This structure not only looks appealing under a microscope but also serves a functional purpose. The arrangement allows for compact storage of genetic information and provides a mechanism for replication and repair.
The unique feature of the double helix model is its anti-parallel strands, which means that the two strands run in opposite directions. This is a pivotal aspect as it facilitates the proper pairing of nucleotides during DNA replication. Its aesthetic shape, while scientifically important, also allows significant implications for understanding structural biology and genetic engineering, although it can complicate the processes involved in transcription and translation by requiring specific enzymes to navigate the structure.
Nucleotide Sequences
Nucleotide sequences are the specific arrangements of nitrogenous bases along the DNA strand. These sequences encode the information necessary for building proteins and determining organism traits. The arrangement of these sequences is like a language, one that scientists are constantly learning to decode.
The primary characteristic that stands out in nucleotide sequences is their variability. Variations in these sequences, whether due to random mutations or inherited alterations, underpin the diversity of life. This variability is invaluable when investigating genetic linkage and association studies, as it provides insight into disease susceptibility and evolutionary relationships. Yet, the decoding of these sequences can become a convoluted affair, especially when dealing with large genomes where the sheer amount of data can overwhelm even the most seasoned researchers.
Functionality of DNA
Gene Expression
Gene expression involves the transformation of genetic information contained in DNA into functional products, mostly proteins. This process encompasses two phases: transcription, where DNA is copied into mRNA, and translation, where that mRNA is utilized to form proteins. The efficiency of this process determines how traits are realized and expressed in organisms.
A key point here is the regulation of gene expression. Different cells express different genes; for example, a liver cell will express a different set of genes compared to a muscle cell. This adaptability is crucial for maintaining cellular functions and responding to environmental changes. However, interruptions in gene expression mechanisms can lead to diseases, including cancer. This multifaceted role of gene expression makes it a popular area of study for those investigating molecular biology and genetics.
Replication Processes
DNA replication is a vital process that ensures genetic information is copied accurately before cell division. This process involves a host of enzymes—such as DNA polymerase—that sequentially unwind the DNA strands and synthesize new complementary strands from the existing template.
An important characteristic of this process is its high fidelity. The enzymes not only match the appropriate nucleotides to the template strands but also have mechanisms to check and correct errors. This high accuracy is beneficial in preserving genetic stability but can also imply that mutations are exceedingly rare. However, these rare occurrences are still significant, as they contribute to genetic diversity within populations, enabling adaptation and evolutionary changes.
Mutations and Variability
Mutations refer to changes in the DNA sequence that can arise spontaneously or from environmental factors. These can be point mutations, insertions, deletions, or large-scale chromosomal rearrangements. The variability brought about by mutations is fundamental for natural selection but can also lead to various genetic disorders.
One particularly compelling aspect of mutations is their dual nature as both beneficial and detrimental. While they can give rise to new traits that enhance survival or reproduction, they can also precipitate genetic diseases. This dichotomy makes studying mutations crucial, as understanding their sources, mechanisms, and impacts is necessary for medical and ecological research. Moreover, the challenge lies not just in identifying mutations but also deciphering their potential effects on organismal biology.
Without an understanding of DNA, we cannot navigate the complexities of life itself. One might say that DNA is life's instruction manual, and decoding it paves the way for advancements in a myriad of disciplines.
Without an understanding of DNA, we cannot navigate the complexities of life itself. One might say that DNA is life's instruction manual, and decoding it paves the way for advancements in a myriad of disciplines.
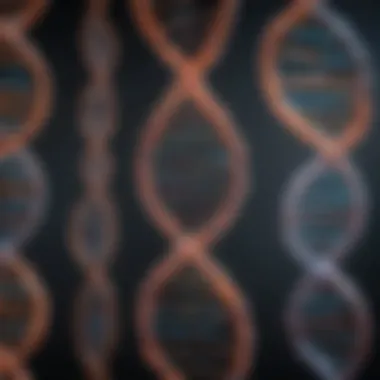
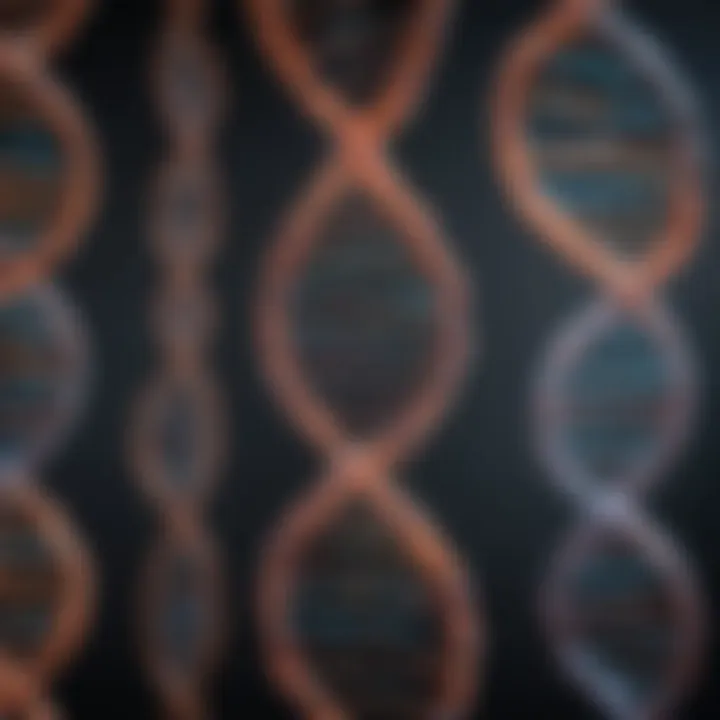
Techniques to Isolate DNA
Understanding the various techniques to isolate DNA is crucial in the field of genetics and biotechnology. These methods enable researchers and forensic scientists to extract genetic material from a variety of sources, which is foundational for analysis in multiple applications. Each technique comes with its own benefits and considerations, which makes it essential to choose the right approach based on the specific objectives and the type of sample being studied.
Histological Techniques
Histological techniques play a vital role in analyzing tissues and cellular structures where DNA is contained. Techniques under this umbrella allow for careful preparation and manipulation of biological samples, ensuring that the integrity of the DNA is maintained while preparing for analysis.
Sample Preparation
Sample preparation involves the meticulous process of preparing biological specimens for examination. This initial step is integral to ensure accurate and reliable results down the line. A key characteristic of sample preparation is that it is adaptable, allowing scientists to work with various tissues, whether plant or animal. This versatility is one reason it's a popular choice.
The unique feature of sample preparation is the use of a method called homogenization. This process breaks down tissues into a uniform mixture, which is beneficial as it enhances the extraction efficiency of DNA. However, it can also come with disadvantages such as the risk of introducing contaminants that might affect the purity of the DNA. Therefore, careful methodology is paramount to avoid such pitfalls in any ensuing analysis.
Staining Methods
Staining methods are pivotal for visualizing DNA and its associated structures within cells. These methods enhance the contrast of biological samples, thus allowing for easier identification of where DNA is present. The key characteristic of staining methods is their ability to provide clear visual insight into the cellular organization, making them incredibly valuable in histological studies.
A unique aspect of staining methods is the use of fluorescent dyes, which can illuminate specific segments of DNA when exposed to particular wavelengths of light. This can be exceptionally advantageous for pinpointing particular regions within chromosomes. However, one should also consider that overstaining or the wrong application can lead to confusion in interpretation, emphasizing the need for a careful balance in the technique used.
Molecular Biology Methods
Molecular biology methods are broad-reaching and can provide powerful results in DNA isolation. Each of these techniques targets specific aspects of DNA for retrieval, making them essential tools in modern biological research.
Polymerase Chain Reaction (PCR)
The Polymerase Chain Reaction, commonly known as PCR, is a powerful technique for amplifying small segments of DNA. What makes PCR particularly famous is its ability to produce millions of copies of a specific DNA sequence in just a few hours. This quality is what has made it a cornerstone technique in many genetic studies and applications.
Now, the unique feature of PCR lies in its temperature cycling process, where repeated heating and cooling allow for denaturing, annealing, and elongation. This makes it incredibly efficient and effective; however, it can be sensitive to contamination, which may potentially give rise to false positives. Thus, strict protocol adherence is essential.
Gel Electrophoresis
Gel electrophoresis is another pivotal method that serves to separate DNA fragments based on size. This technique is particularly beneficial in visualizing the results from PCR and other amplification methods, ensuring that the DNA fragments are of the expected size range.
A key aspect of gel electrophoresis is its straightforwardness; DNA fragments can be viewed after separation, which allows for immediate analysis. Additionally, the ability to use various gel types—such as agarose or polyacrylamide—adds to its versatility in accommodating different fragment sizes. However, it has limitations; for example, it cannot offer precise quantification of DNA, which could be a downside in certain applications.
Restriction Enzyme Digestion
Restriction enzyme digestion is a critical method used to cut DNA at specific sequences. This technique allows for customized manipulation of DNA, making it therefore indispensable for cloning and assembling genetically modified organisms. Its unique characteristic is its precision; restriction enzymes can target very specific sites in the DNA sequence, which can be a powerful tool in genetic engineering.
However, potential disadvantages stem from the fact that one is limited to the available restriction sites, which can constrain the types of manipulations possible. Moreover, the need for careful enzyme selection and handling showcases the complexity involved in executing this technique successfully.
Next Generation Sequencing
Next generation sequencing (NGS) has revolutionized the way DNA is analyzed, enabling rapid sequencing at unprecedented scales. The ability to sequence an entire genome in a fraction of the time and cost compared to traditional methods makes NGS a significant development in genetic research.
Principles of Sequencing
The principles of sequencing involve various methodologies that allow for the reading of nucleotide sequences in DNA. This is paramount for understanding genetic variability and structure. One key characteristic that sets NGS apart is its parallel processing ability, which allows thousands of DNA strands to be sequenced at once; this massively boosts throughput.
A unique aspect of NGS is its ability to provide high-quality data quickly, which is essential for both clinical diagnostics and research purposes. However, while it offers extensive detail, the interpretation of sequence data can be complex and requires sophisticated bioinformatic tools, which could be a hurdle for some researchers.
Applications in Genomics
When applied to genomics, next generation sequencing opens doors to a multitude of applications, from identifying genetic disorders to personalized medicine approaches. The key characteristic of its application in genomics is the detailed insights it provides into genetic variations among individuals.
The unique feature of using NGS in genomics is its scalability; researchers can choose to focus on specific regions of interest or conduct whole-genome sequencing. However, the expense associated with high-quality sequencing can be a limitation, especially for smaller labs or projects with constrained budgets. Nevertheless, the potential for groundbreaking discoveries emphasizes its importance in the field.
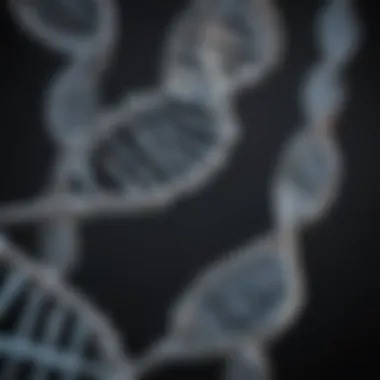
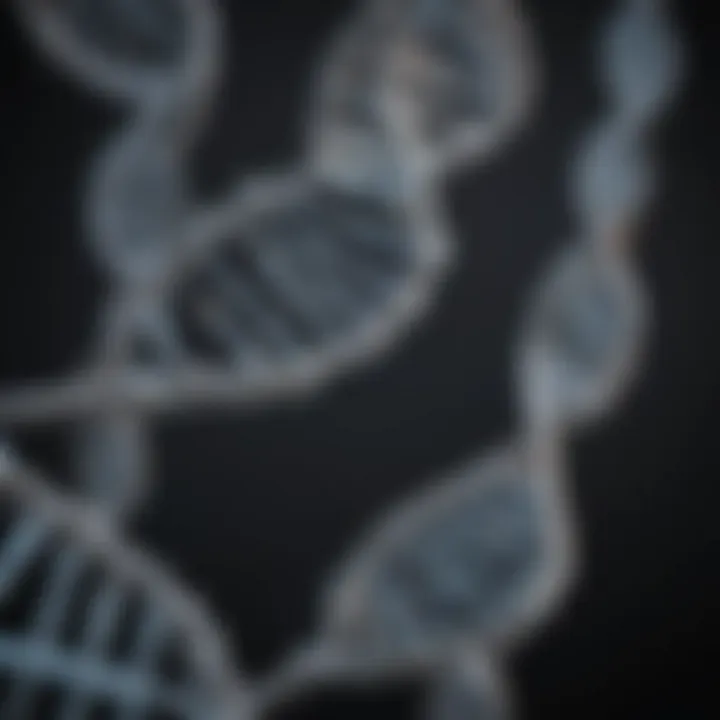
Applications of DNA Analysis
The analysis of DNA has far-reaching implications across various fields. Its importance in modern science cannot be overstated, as it often serves as the backbone for advancements in forensics, medicine, and historical research. With the power to provide insights into identity, health, and ancestry, DNA analysis helps unlock numerous mysteries.
Forensics and Criminal Justice
DNA Fingerprinting Techniques
DNA fingerprinting, or DNA profiling, is a method used to uniquely identify individuals based on their DNA. It hinges on the analysis of specific regions of DNA that vary among individuals. This technique is invaluable to law enforcement, providing a means to connect suspects to crime scenes through biological evidence. The key characteristic of DNA fingerprinting is its precision in individual identification. For example, in a murder case where blood is found at the scene but no clear suspect, forensic scientists can analyze the blood's DNA and match it with potential suspects. This powerful capability makes DNA fingerprinting a prominent choice for criminal investigations.
One unique feature of DNA fingerprinting is its ability to distinguish between identical twins, who share nearly the same genetic coding. This strengthens its credibility and application in courts, where DNA evidence can sway the jury's decision. However, the technique does have its drawbacks, including the possibility of contamination or misinterpretation of results, which can lead to wrongful convictions. Despite these disadvantages, the benefits in providing accurate, scientific evidence of identity are considerably significant, proving its worth in forensic science.
The Role of DNA in Cold Cases
Cold cases, often regarded as unsolved mysteries, can benefit greatly from advancements in DNA technology. The ability to revisit old evidence with modern methods can lead to breakthroughs that were not feasible at the time of the original investigation. Cold cases that may have faded from public attention can be reignited when DNA analysis brings new hope for justice.
A vital aspect of employing DNA in cold cases is the tracking of both male and female lineages through Y-chromosome and mitochondrial DNA analysis. This dual approach can provide two pathways to identify potential suspects or victims. Notable cases, such as the identification of historical remains or exoneration of wrongfully convicted individuals, highlight how transformative DNA can be in these scenarios. However, it's also crucial to note that re-examining evidence usually demands resources and time, which can be constrained in law enforcement. Still, these efforts underscore the profound role of DNA analysis in seeking closure for victims and families.
Medical Diagnostics
Genetic Testing
In the field of medicine, genetic testing has emerged as an essential tool for understanding diseases and conditions at a molecular level. It involves analyzing an individual’s DNA to detect genetic disorders or predispositions. The primary purpose of such testing is to inform patients about their health risks and treatment options based on their genetic makeup. Genetic tests can identify everything from single-gene disorders to complex polygenic conditions.
One key characteristic of genetic testing is its ability to guide personalized medicine, allowing for tailored treatments that suit individual genetic profiles. For instance, individuals with a known mutation linked to breast cancer, such as BRCA1 or BRCA2, can make informed decisions about preventive measures. However, the unique feature of genetic testing lies in its dual role: it aids in early detection and helps assess familial risk. Despite it being a beneficial tool, there are ethical considerations surrounding the privacy of genetic information and potential discrimination.
Targeted Therapies
Targeted therapies represent a significant advancement in cancer treatment, using genetic information to direct the therapeutic approach towards specific genetic markers of a tumor. This strategy enhances the efficacy of treatment while reducing the side effects traditionally associated with chemotherapy. The crux of targeted therapies is to selectively attack cancer cells based on their unique genetic profiles, rather than harming healthy cells.
Its key characteristic is that it offers a more precise treatment modality, benefitting patients with better outcomes compared to conventional therapies. For example, in cases of lung cancer, identifying mutations in the EGFR gene can lead to tailored treatments with medications designed specifically to counteract those mutations. Nevertheless, while targeted therapies show promise, they are not universally applicable to all patients or cancers, leaving some with limited prospects.
Historical and Ancestry Research
Understanding Human Migration
DNA analysis has vastly aided in unraveling the complexities of human migration patterns throughout history. By examining genetic variations across different populations, researchers can piece together how and when humans dispersed across the globe. This is key for anthropologists and historians alike, offering context to historical narratives.
The primary insight gained from understanding human migration through DNA is that it highlights shared ancestry and diversity within human populations. Each migration pattern tells a story about adaptation, survival, and cultural exchange. However, the unique aspect of such studies often lies in the interpretation of ancient DNA, which can be challenging due to environmental degradation. Despite these challenges, the knowledge gained remains crucial for comprehending human evolution.
Genetic Ancestry Testing
Genetic ancestry testing has grown in popularity, offering individuals insights into their family history and ethnic backgrounds. This form of DNA analysis can connect people to their heritage and illuminate historical migrations that may be unknown to them. By examining specific markers in an individual's DNA, companies can provide a breakdown of their ancestral origins, linking them to various regions or populations.
One major characteristic of genetic ancestry testing is its accessibility; many services are available at a relatively low cost compared to extensive genealogical research. However, it is vital to understand that the results may not always reflect an individual's complete heritage, which is often shaped by complex historical events. Since not everyone has provided DNA samples to these databases, the conclusions drawn can be limited. Still, the value of genetic ancestry testing lies in its ability to connect individuals with their roots, fostering a broader understanding of one’s lineage.
Ethical Considerations
Discussing DNA often leads down a path that intertwines science with ethics. As the tools for manipulating and analyzing DNA continue to evolve, several ethical considerations come to the fore. It's critical to navigate these waters carefully, as they harbor implications not only for individual privacy but also for broader societal norms.
Privacy Issues in DNA Collection
DNA collection raises significant privacy concerns, particularly in a world that's increasingly data-driven. The ability to trace one's ancestry or solve crimes can bring about a host of ethical dilemmas.
Informed Consent
Informed consent is a cornerstone of ethical practices in DNA research. This concept hinges on the idea that individuals have the right to understand how their genetic information will be used and what implications this might hold for them. An important characteristic of informed consent is its requirement for clear communication; people need to know exactly what they're agreeing to. This approach fosters trust between researchers and participants. Informed consent is popular not just because it is a legal necessity, but because it respects the autonomy of individuals.
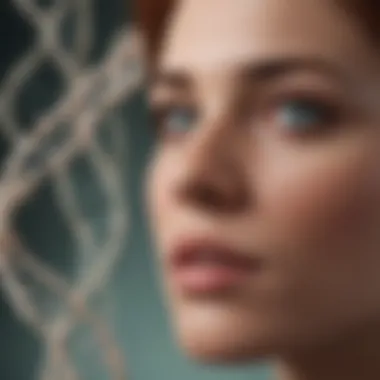
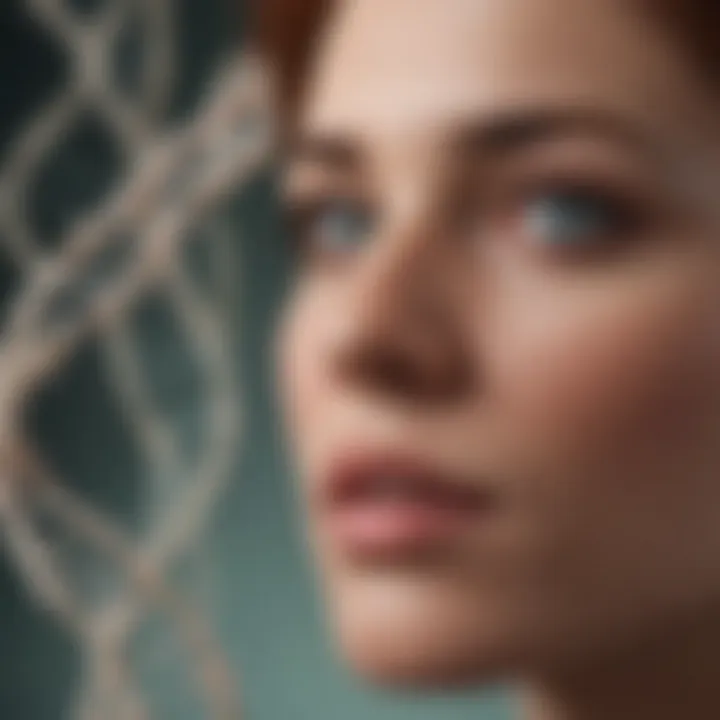
However, there are unique challenges associated with it. Sometimes, people may not fully grasp the technical jargon or the long-term implications of sharing their genetic data. It's an advantage that researchers can build more ethically responsible models, but a disadvantage lies in the potential misunderstandings that may arise from complicated explanations.
Data Security
Data security plays a crucial role in protecting the information gathered during DNA collection. At its core, data security is about ensuring that sensitive genetic information is safeguarded from unauthorized access. The key characteristic of data security is its multifaceted approach, involving encryption, secure storage, and strict access controls. This makes it a beneficial topic for considering ethical implications as researchers tackle the challenge of maintaining confidentiality.
A distinctive feature of data security in DNA research is its ever-evolving nature, adapting to new threats and vulnerabilities. While it provides peace of mind, challenges arise, such as the risk of data breaches. If data security fails, the consequences can be dire—individual identities could be exposed, leading to discrimination or stigmatization.
Implications of Genetic Editing
Genetic editing, particularly through advanced methods like CRISPR, has opened doors previously thought closed. It represents incredible potential but also brings with it a series of ethical quandaries that demand attention.
CRISPR Technology
CRISPR technology stands out in discussions about genetic editing. Its precision and versatility make it a game-changer in the field. The unique feature of CRISPR lies in its ability to target specific sequences in DNA, enabling precise modifications. This capability is what has made CRISPR a popular choice among researchers looking to advance medicine and agriculture.
However, the technology's power raises questions. While it allows for the correction of genetic disorders, it also opens the door to potential misuse—designer babies, for instance. Thus, while it offers advantages, the ethical balance must always be considered.
Potential for Ethical Dilemmas
The potential for ethical dilemmas in genetic editing remains a significant concern. The practice carries with it key issues related to the consequences of altering genetic information. One potential dilemma is that the power to edit genes could lead to discrimination based on genetic makeup. This makes it a crucial topic in this article, as society grapples with what it means to wield such power.
A notable characteristic of these dilemmas is their complex nature; choices made today could resonate for generations to come. While genetic editing may offer life-saving treatments, it also poses profound ethical questions, such as who gets to decide which traits are preferred.
In summary, as we venture further into the realm of DNA analysis and manipulation, these ethical considerations will play an increasingly pivotal role. Addressing them head-on is not only vital for responsible research but also for fostering public trust in scientific advancements.
Future Directions in DNA Research
As we peer into the future, the realm of DNA research is buzzing with possibilities that could reshape medicine and biotechnology. The exploration of DNA is ever-evolving, and understanding where it’s headed can provide insights that are crucial for students, researchers, educators, and professionals alike. An emphasis on gene therapy advancements and the integration of artificial intelligence signifies a transformative approach that holds great promise. These directions are not just about technical innovation; they have real implications for health care, disease management, and beyond.
Advancements in Gene Therapy
Current Research Trends
Current research trends in gene therapy show a lively intersection of genetics and therapeutic innovation. Scientists are honing methods to correct genetic disorders at their root by delivering therapeutic genes to patients. One key characteristic of these trends is personalized medicine, tailored therapies that take a patient's unique genetic makeup into account. This personalized approach is particularly exciting, as it allows for targeted treatments with potentially fewer side effects.
Specific features of current research often include viral vectors that facilitate the safe transfer of genes into cells. While this method has shown promising advantages, such as high efficiency and specificity, it does pose challenges like immune responses or vector-related complications. Therefore, careful evaluation is crucial as researchers advance in this pivotal area.
Case Studies of Success
Looking at case studies of success sheds light on practical applications of these advancements. One highlighted success story is Luxturna, a treatment for a rare genetic eye disorder that restores vision effectively. This case stands out because it showcases how a single treatment can bring life-changing benefits.
Moreover, the sustained effects of treatment have sparked hopes for curing other genetic disorders. However, success doesn't come without its caveats; the high costs and limited accessibility of such treatments raise questions about equity and distribution in healthcare. Thus, while celebrating accomplishments, it's also critical to discuss challenges and potential solutions in making treatments more accessible.
Integrating AI and DNA Research
Predictive Models in Genetics
The integration of AI in DNA research represents a transformative frontier. With predictive models in genetics, researchers can uncover patterns from vast datasets, allowing for more accurate predictions of genetic diseases before they manifest. A notable characteristic of this approach is its ability to process an immense number of variables efficiently. This option proves beneficial because it can lead to early interventions and tailored healthcare strategies, ultimately improving patient care.
One unique feature of predictive modeling involves machine learning algorithms that are utilized to identify genetic markers associated with specific diseases. While promising, the limitations include the potential misinterpretation of data or overfitting models, which can lead to inaccuracies.
Data Analysis and Interpretation
Equally important is the aspect of data analysis and interpretation, which complements predictive models by providing the necessary context for understanding genetic information. Through advanced analytic techniques, researchers can derive significant meaning from complex data sets, leading to clearer insights. This capability is crucial for deciphering genetic variants and their implications.
Another distinct feature is the interdisciplinary collaboration that enhances these analyses. By combining knowledge from genetics, statistics, and computer science, better analysis outcomes emerge. Nevertheless, challenges remain surrounding data privacy and the need for robust infrastructure to manage the enormous volume of genetic information being generated. Such considerations will be critical as the field advances.
"The future of DNA research rests not just in the lab, but in the crossroads of technology, ethics, and society's needs."
"The future of DNA research rests not just in the lab, but in the crossroads of technology, ethics, and society's needs."
In summary, exploring future directions in DNA research signifies not only a technological leap but also an ethical call to action. Advancements in gene therapy and the integration of AI into DNA research hold incredible potential, but they also come with nuances and implications that must be navigated thoughtfully. A concerted effort towards equitable access to these innovations is key for realizing the full benefits of advancements in DNA research.