Exploring Gravity: Foundations and Current Research
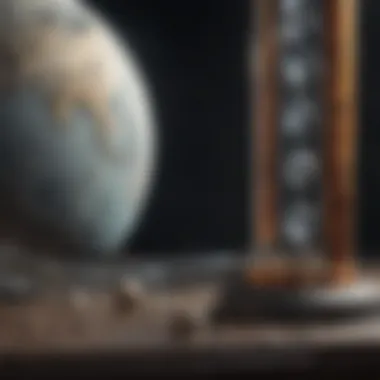
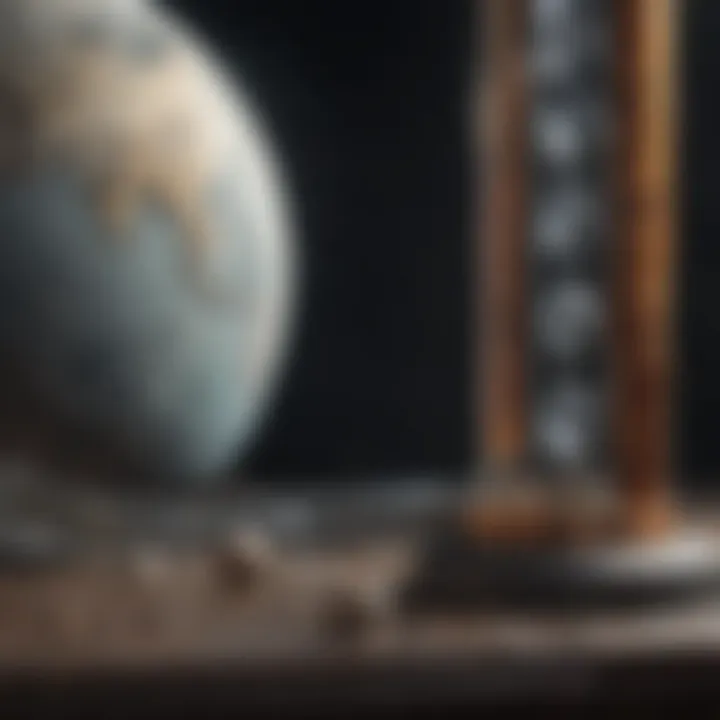
Intro
Gravity is a concept that transcends simple understanding; it is a cornerstone of physics and astronomy. Although often taken for granted, gravity is the force that governs the motion of planets, stars, galaxies, and even light. Its influence is profound, shaping not only the physical world but also the very fabric of our theories of the universe.
The development of gravitational theory has evolved significantly since ancient times. Early civilizations viewed gravity through a mystical lens, attributing movement to divine forces. It was not until Sir Isaac Newton in the 17th century that gravity was articulated as a universal force, described mathematically in his law of universal gravitation. This marked a pivotal moment in scientific thought.
In contemporary research, gravity continues to be a topic of awe and investigation. Advances in technology allow scientists to probe deeper into its nature and effects, fueling ongoing debates and discoveries about the universe. From black holes to gravitational waves, modern physics unravels the complexities of this fundamental force.
Understanding gravity is essential for students, researchers, educators, and professionals across various fields. This article will explore key concepts surrounding gravity, its implications, and the latest research trends, aiming to provide a comprehensive understanding of this vital topic.
Prelude to Gravity
Gravity is a fundamental force that influences everything in our universe. Understanding gravity is crucial for fields such as physics, astronomy, and even engineering. This section lays the groundwork for the entire article. It aims to clarify why gravity is more than just a force that keeps us grounded.
The role of gravity extends far beyond keeping objects from floating into space. It governs the motion of celestial bodies, affects the rate of time dilation, and plays a vital part in the structure of the universe itself. By grasping the concepts surrounding gravity, readers can appreciate its intricate connections to various scientific disciplines and its impact on daily life.
Historical Perspectives
The exploration of gravity dates back thousands of years. Ancient Greeks began to ponder its nature, though they could not provide a clear understanding of it. In the sixteenth century, Nicolaus Copernicus proposed the heliocentric model, suggesting that the Earth revolves around the Sun. This challenge to established beliefs laid the groundwork for future scientific inquiry.
Isaac Newton’s announcement of the Law of Universal Gravitation in the seventeenth century marked a significant milestone. Newton articulated that every mass attracts every other mass in the universe. His observations and calculations provided a framework that could be applied to everything, from falling apples to orbiting planets. Without Newton’s contributions, the discussion on gravity would likely be in its infancy.
Throughout the nineteenth and twentieth centuries, science continued to evolve. Albert Einstein’s theory of General Relativity transformed our understanding by presenting gravity not just as a force but as a curvature of spacetime caused by mass. This evolution in thought highlights the dynamic nature of scientific understanding and the continual reassessment of established principles.
Definition and Significance
Gravity can be defined as the force by which a planet or other celestial body attracts objects toward its center. While the concept may seem straightforward, its significance is far-reaching.
- Impacts on daily life: Gravity keeps everything anchored to the Earth. It enables our existence, guiding how we move and interact with our environment.
- Celestial phenomena: Gravity shapes the orbits of planets, the formation of galaxies, and even the movement of light. Black holes, for instance, reveal the extreme impacts of gravitational forces.
- Technological advancements: Understanding gravity leads to applications in technology. Satellite systems, for example, must account for gravitational pulls when calculating trajectories.
The study of gravity is therefore essential not only for pure scientific curiosity but also for practical applications that enhance our understanding of the universe. As new research emerges, it becomes increasingly evident that the pursuit of knowledge about gravity will yield new insights into both the origin of our universe and the dynamics of everything within it.
Newton’s Law of Universal Gravitation
Newton's Law of Universal Gravitation is a cornerstone in the study of classical physics. This law states that every particle of matter in the universe attracts every other particle with a force that is directly proportional to the product of their masses and inversely proportional to the square of the distance between them. Formulated by Isaac Newton in the late 17th century, it fundamentally transformed how we understand the nature of forces and motion. The impacts of this law continue to resonate through various fields of science.
The significance of Newton's Law goes beyond academic interest. It provides a framework that explains a range of phenomena, from the falling of an apple to the earth, to the orbit of planets around the sun. This law initiated a shift in scientific thought from a geocentric to a heliocentric perspective. It made it possible to calculate the gravitational force between objects, contributing to the development of modern physics.
Formulation of the Law
Newton's formulation introduced the equation:
F = G \fracm_1 m_2r^2
Here, F represents the gravitational force between two bodies, m1 and m2 are the masses of the bodies, r is the distance between the centers of the two masses, and G is the gravitational constant, approximately equal to 6.674 × 10⁻¹¹ N(m/kg)². This equation elegantly encapsulates the relationship between mass, distance, and gravitational force. It is not only simple yet profound, allowing for calculations in various contexts, from terrestrial to astronomical.
The precision of this formulation has made possible advances in fields such as astronomy, engineering, and even technology development. For instance, satellite launches rely on these calculations to ensure successful orbits around Earth. The law also laid the groundwork for the later development of fields like celestial mechanics.
Impacts on Classical Physics
The implications of Newton's Law of Universal Gravitation extend deeply into classical physics. First, it challenges previous notions held by ancient scholars. Before Newton, gravity was largely a subject of philosophical contemplation without rigorous mathematical grounding.
- Practical Applications: The law allows engineers to design structures that consider gravitational forces, influencing everything from bridges to skyscrapers.
- Astronomy: It guided astronomers in predicting planetary positions and understanding celestial mechanics. Calculations of orbits were made possible, benefiting navigation and space exploration.
- Physics Education: It serves as a critical part of the educational curriculum, aiding in the understanding of forces and movement.
"The law not only united terrestrial and celestial mechanics, it fundamentally reshaped understanding of the universe."
"The law not only united terrestrial and celestial mechanics, it fundamentally reshaped understanding of the universe."
In essence, Newton's Law has cemented its place as a vital topic in scientific literature. It is a key concept that bridges historical developments and modern implications of gravity, showing the perpetual relevance of Newton's insights in scientific exploration.
Einstein and General Relativity
Einstein's theory of general relativity revolutionized the understanding of gravity. Its implications extend beyond physics, influencing various fields including cosmology and even philosophy. Understanding this topic is crucial for recognizing how gravity functions not just as a force but as a curvature of spacetime dictated by mass. This approach transformed the classical mechanics narrative established by Newton.
Fundamental Concepts
General relativity introduces several fundamental concepts that challenge traditional notions of gravity. First, Einstein proposed that gravity is not a force exerted at a distance but a result of curved spacetime caused by mass.
Key ideas include:
- Spacetime: The merging of three-dimensional space and time into a four-dimensional continuum.
- Curvature of spacetime: Massive objects like planets and stars warp the fabric of spacetime, causing other nearby objects to follow curved paths.
- Geodesics: The paths taken by objects moving under the influence of gravity without any other forces acting upon them. In curved spacetime, these paths are not straight lines but curves.
This idea of spacetime curvature replaces the concept of gravitational force with a geometrical perspective. In simple terms, objects follow the contours of this curvature, leading to the experience of gravity. The famous equation, (E=mc^2), supports this by illustrating the relationship between energy, mass, and the geometrical structure of spacetime.
Predictions and Experimental Verifications
General relativity has led to numerous predictions that have been confirmed through various experiments and observations. Some notable predictions include:
- Bending of Light: Light rays passing near massive bodies, like the sun, bend due to spacetime curvature. This was famously observed during a solar eclipse in 1919, providing empirical support for Einstein's theories.
- Time Dilation: An object's gravitational field influences time. Clocks in stronger gravitational fields tick slower than those in weaker fields. This phenomenon is critical for technologies, such as the Global Positioning System (GPS), where satellite timekeeping must account for general relativity.
- Gravitational Redshift: The wavelength of light escaping a gravitational field is stretched, shifting towards the red end of the spectrum. This effect has been confirmed through numerous experiments, demonstrating the profound impacts on light as it interacts with gravity.
These predictions not only reinforce Einstein's theories but also continue to inspire ongoing research. As scientists explore deeper realms of space and time, general relativity remains a cornerstone of modern physics.
"It is not the force that makes an object fall, but the curvature of space around it."
"It is not the force that makes an object fall, but the curvature of space around it."
Understanding these concepts is vital for anyone studying gravity or even engaging in discussions about the universe at large. Through these insights, we grasp the essential nature of gravity, influencing everything from the formation of galaxies to the path of light across the cosmos.
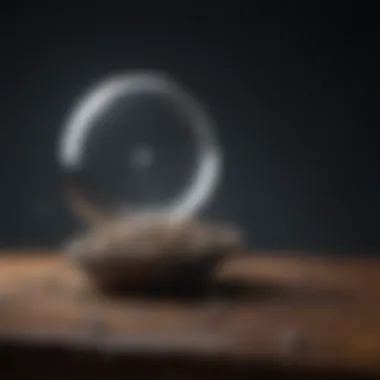
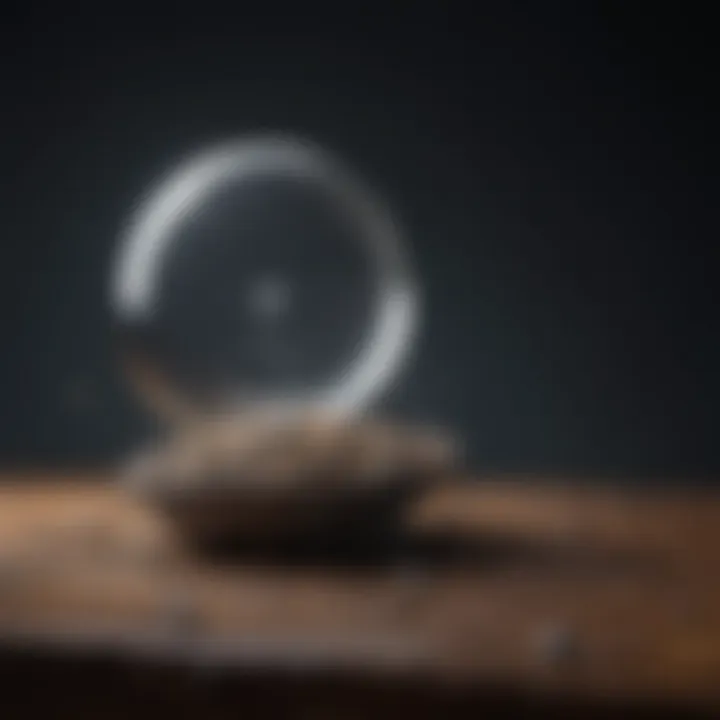
Gravity in Modern Physics
Gravity, a fundamental force, plays an essential role in our understanding of the universe. In modern physics, its implications extend beyond classical mechanics into realms governed by the principles of quantum mechanics and cosmology. Addressing gravity's significance requires examining its intricate connection with other fundamental forces and how it influences the fabric of spacetime.
The necessity of integrating gravity into modern physics is highlighted by the ongoing quest to unify the various forces of nature. This integration has spurred research into theories that reconcile general relativity with quantum field theory, two pillars of contemporary physics. The challenges of these reconciliations pose intriguing questions, pushing scientists to explore complex ideas and refine existing theories.
Quantum Gravity Theories
Quantum gravity theories attempt to bridge the gap between general relativity and quantum mechanics. The essence of these theories lies in the application of quantum principles to gravitational interactions. There are several notable approaches, such as loop quantum gravity and causal dynamical triangulation. Each presents a distinct method of addressing the challenges associated with gravity at microscopic levels.
For instance, loop quantum gravity proposes that spacetime is composed of discrete units called "loops." This contrasts with the classical view of spacetime as a smooth continuum. Researchers are currently conducting experiments to observe effects predicted by these theories, which could lead to groundbreaking insights. More research is neeeded to confirm these theories’ potential and their implications for our comprehension of gravity.
String Theory and Gravity
String theory further extends the conversation, suggesting that fundamental particles are not point-like but rather one-dimensional strings. This theory unifies gravity with the other three fundamental forces: electromagnetism, weak nuclear force, and strong nuclear force. The beauty of string theory lies in its ability to project higher dimensions, allowing for a richer understanding of gravitational interactions.
Within string theory, the graviton, a hypothetical particle, serves as the quantum representation of gravity. This theoretical framework allows physicists to study gravitons as potential carriers of gravitational force, providing new pathways in theoretical research. Currently, it garners significant attention, particularly in the search for a viable theory of quantum gravity. The implications of string theory not only challenge traditional views but also reshape how we envision the universe’s structure.
The synthesis of gravitational theories in modern physics showcases its complexities and the relentless pursuit for understanding. These explorations hold the promise of unlocking new dimensions of knowledge, paving the way for future breakthroughs.
Gravitational Waves
Gravitational waves represent a profound aspect of our understanding of gravity, connecting theoretical physics to observable phenomena in the universe. These waves are produced by certain movements of massive objects, such as colliding black holes or neutron stars. They carry information about these cataclysmic events and allow us to study aspects of the universe that were previously inaccessible.
Understanding gravitational waves enhances our grasp of fundamental physics and challenges the boundaries of our current theories. The detection of gravitational waves opens new avenues for exploration in cosmology and astrophysics. Scientists can now observe the universe in a novel way, beyond traditional electromagnetic observations, thus providing a more comprehensive view of cosmic events.
Discovery and Significance
The first direct observation of gravitational waves occurred on September 14, 2015, by the Advanced LIGO detectors. This landmark detection confirmed a prediction made by Albert Einstein nearly a century earlier in his general relativity theory. The event, resulting from the merger of two black holes, was the culmination of decades of research, investment, and technological advancements.
The significance of this discovery extends beyond mere validation of Einstein's theory. It presents an entirely new method of observing the universe. Gravitational waves do not interact with matter in the same way that light does, allowing scientists to detect events that are invisible to traditional telescopes. The ability to listen to the universe has led to insights into the lifecycle of stars, the formation of black holes, and the dynamics of neutron stars.
"Gravitational waves provide a new tool for exploring the universe, revealing phenomena we could only theorize about before."
"Gravitational waves provide a new tool for exploring the universe, revealing phenomena we could only theorize about before."
This new observational capability enriches our understanding of the fundamental processes governing the cosmos.
Implications for Astrophysics
The implications of gravitational wave research for astrophysics are immense. By analyzing the data received from gravitational wave events, researchers can glean information about the mass, spin, and distance of the objects involved. This information can lead to more accurate models of stellar evolution and the dynamics of galaxies.
Moreover, gravitational waves have the potential to uncover the existence of populations of black holes or neutron stars that may not emit light. The study of gravitational waves can also provide evidence supporting or challenging existing theories about dark matter and dark energy.
The interdisciplinary nature of this field encourages collaboration among physicists, astronomers, and engineers. Experiments like LIGO and Virgo are already working on future upgrades that will increase sensitivity, allowing for the detection of weaker signals, thereby expanding our observational reach.
The Role of Gravity in Cosmology
Gravity plays a pivotal role in shaping our understanding of the cosmos. It is not just a force that draws objects together; it is the architect of the universe's structure. The gravitational force is responsible for the formation of galaxies, stars, and planetary systems. Understanding this force provides insight into the dynamics and evolution of the universe. The study of gravity in cosmology is essential for explaining not only how celestial bodies interact but also how the universe itself behaves on a large scale.
One must consider the importance of gravity's effects on cosmological models. The influence of gravity is evident in various phenomena, from the orbits of planets to the expansion of the universe. Gravitational interactions dictate the distribution and motion of matter in space. As a result, studying gravity helps us understand the underlying processes that govern the formation and fate of cosmic structures.
Gravity and the Structure of the Universe
The structure of the universe is largely defined by gravity. This force causes matter to cluster together, forming galaxies and galaxy clusters. Over vast timescales, gravity organizes the matter in the universe into a web-like structure called the cosmic web. This web consists of filaments of dark matter and galaxies interconnected by gravity.
Key points about gravity's role in the structure of the universe include:
- Formation of Structures: Initially, small fluctuations in the density of matter led to gravitational attraction, resulting in the creation of stars and galaxies.
- Galactic Dynamics: The behavior of stars within galaxies is governed by gravity. This interaction leads to the rotation curves that provide clues about the presence of dark matter.
- Cosmological Evolution: As the universe expands, gravity affects the rate of expansion and the fate of cosmic structures. Understanding how gravity influences this evolution is an ongoing area of research.
Dark Matter and Gravitational Effects
Dark matter is a crucial component in the study of gravity in cosmology. Although invisible and undetectable through conventional means, it accounts for a significant portion of the universe's total mass. Its presence is inferred from the gravitational effects it exerts on visible matter.
The significance of dark matter can be summarized as follows:
- Gravitational Lensing: Dark matter’s mass bends light, allowing astronomers to observe its effects even though it cannot be seen directly. This phenomenon, known as gravitational lensing, creates optical distortions around massive objects.
- Structure Formation: Dark matter provides the gravitational backbone that holds galaxies and clusters together. The distribution of dark matter influences the formation of visible structures in the universe.
- Competing Theories: Understanding dark matter is critical as it raises fundamental questions regarding the nature of gravity. Theories such as Modified Newtonian Dynamics (MOND) have emerged, attempting to explain the observed gravitational effects without invoking dark matter.
"Gravity is not merely a question of weight; it is essential to understanding how the universe organizes itself, driving both formation and evolution of cosmic structures."
"Gravity is not merely a question of weight; it is essential to understanding how the universe organizes itself, driving both formation and evolution of cosmic structures."
In summary, gravity is the cornerstone of cosmology. It shapes the structure of the universe, influences the dynamics of celestial bodies, and provides the framework for understanding phenomena like dark matter. Ongoing research continues to opene possibilities, shedding light on the intricate relationship between gravity and the universe.
Gravitational Theories Across Disciplines
Gravitational theories span various disciplines, influencing not only physics but also philosophy and theology. Understanding gravity in these contexts allows a deeper appreciation of its implications beyond mere scientific inquiry. Each discipline approaches gravity from unique angles, enriching the overall discourse about its nature and significance.
Major benefit of exploring gravitational theories across disciplines is that it encourages interdisciplinary collaboration. Physicists’ quantitative analysis of gravitational forces can intersect with philosophical treatments of existence and knowledge. Moreover, theological interpretations may challenge and deepen the conversation about creation and the universe. These interactions can propel new insights that might be overlooked when viewed through a single-lens.
Philosophical Considerations
Philosophical inquiry into gravity often encompasses questions about existence, causation, and the nature of reality. For many centuries, thinkers have pondered what gravity reveals about the fundamental structure of the universe. One significant aspect is the debate about whether gravity is an intrinsic property of matter or a relational phenomenon. Piloting this line of thought encourages discussions regarding substance and essence in the broader context of metaphysics.
Additionally, gravity raises questions about determinism versus free will. If gravity governs motion, to what extent can one argue that human actions are truly autonomous? These philosophical dilemmas invite students, researchers, and educators alike to critically analyze the implications of gravitational forces—both in terms of physical laws and human experience.
"Gravity not only influences celestial bodies but also instigates profound philosophical questions about existence and knowledge."
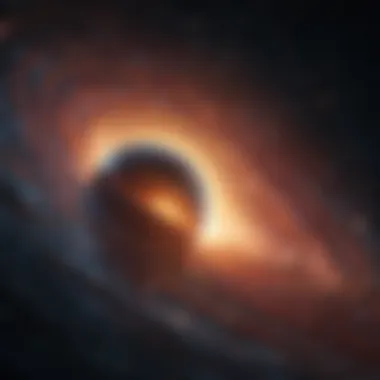
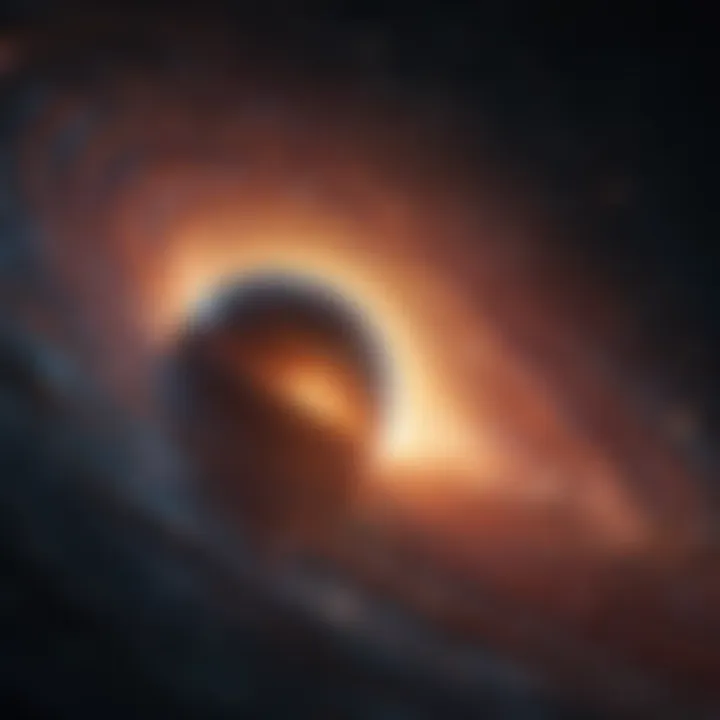
"Gravity not only influences celestial bodies but also instigates profound philosophical questions about existence and knowledge."
One notable philosophical perspective comes from Immanuel Kant, who proposed that space and time are not merely external realities but frameworks through which humans comprehend phenomena. In this view, gravity's influence on spatial relationships reveals more than the mechanics of objects; it can elucidate our cognitive approach to understanding reality itself.
Theological Implications
The relationship between gravity and theology can be complex yet illuminating. Various religious doctrines perceive gravity through the lens of creation and divine order. For instance, many traditions view the laws of physics, including gravity, as manifestations of a creator's design. This view advocates for a universe that is structured and coherent, where gravity serves as a foundational force ensuring stability and continuity.
The theological implications of gravity also extend to discussions about the nature of the universe as a created entity. If gravity governs the heavens and the earth, how does that influence beliefs about the nature of divine providence? This interplay invites theologians to contemplate how they interpret sacred texts in light of scientific advancements.
Moreover, gravity contributes to existential questions such as humanity's place in the cosmos. The role it plays in the formation and sustainability of planets and stars suggests a universe finely tuned for life. This perspective opens pathways for dialogue between scientific findings and spiritual beliefs—a necessary conversation in a world increasingly informed by empirical data.
In summary, engagement with gravitational theories across disciplines fosters a broader understanding of both the scientific and intangible aspects of existence. The philosophical and theological dimensions enrich the study of gravity, making it not only a subject of scientific inquiry but also a profound exploration of human thought.
Gravity's Influence on Technology
Understanding gravity plays a critical role in modern technology. This influence manifests in various sectors, impacting everything from navigation systems to the design of buildings. By comprehending gravitational principles, innovators and engineers can create more effective and efficient solutions. The integration of gravity-based technologies has revolutionized many fields. It has also led to improved safety and performance in numerous applications.
Satellite Navigation Systems
Satellite navigation systems, notably the Global Positioning System (GPS), rely heavily on gravitational principles. These systems use satellites in orbit around Earth to determine precise locations on the planet’s surface. Gravity influences the orbits of these satellites, making it essential to account for it when calculating positions. The effects of Earth's gravitational pull must be considered to achieve the accuracy necessary for navigation.
To ensure accuracy, calculations factor in gravitational time dilation, a concept derived from Einstein's theory of relativity. This time dilation occurs because clocks on satellites run at a different rate compared to those on the ground due to their altitude and speed in Earth's gravity. Without adjustments for this effect, GPS measurements could be off by several kilometers.
Gravity in Structural Engineering
Gravity influences every aspect of structural engineering. Essential elements like building design, material choice, and safety assessments depend on gravitational considerations. Engineers must account for gravitational forces to ensure stability and structural integrity.
The design process includes calculations for static loads—forces acting on a structure when it is at rest. For instance, buildings must be capable of supporting their weight along with additional loads, such as furniture and occupant activities. Failure to regard gravity in these calculations can result in catastrophic failures.
In civil engineering, gravitational forces also dictate the design of bridges and dams. Engineers use specific models to visualize how structures will hold up under gravitational stress.
Key Considerations in Structural Engineering
Key Considerations in Structural Engineering
- Load distribution: Ensures that gravitational forces are effectively managed throughout the structure.
- Material properties: Different materials respond differently to gravitational stress, affecting durability.
- Safety factors: Engineers include additional safety margins beyond expected loads to account for potential unforeseen stresses.
In summary, gravity is not merely an abstract scientific principle but a fundamental aspect of technology that influences our daily lives. By understanding its effects, researchers and engineers can innovate and enhance technological solutions, ensuring systems are both effective and safe.
Impact of Gravity on Biological Systems
The influence of gravity extends beyond the realm of physics and astronomy. It plays a crucial role in shaping biological systems. Understanding gravity’s impact is vital, especially as we explore how living organisms adapt to gravitational forces. In this section, we will examine two key aspects: the effects of gravity on human physiology and the gravitational adaptations in organisms.
Effects on Human Physiology
Gravity has a significant influence on human physiology. It affects various bodily functions and structures. For instance, living in a constant gravitational field shapes our skeletal structure. The human body is designed to function optimally under the force of gravity, which helps keep bones dense and strong. When experiencing microgravity, such as in space, humans undergo distinct physiological changes.
- Bone Density Loss: Astronauts often face decreased bone density due to reduced mechanical loading.
- Muscle Atrophy: Muscles weaken and shrink in microgravity, which can lead to significant health challenges.
- Fluid Redistribution: Gravity causes bodily fluids to shift, impacting cardiovascular function and potentially leading to issues like space motion sickness.
These effects demonstrate that gravity is not merely a force but a fundamental aspect deeply integrated into the health and physiology of humans.
Gravitational Adaptations in Organisms
Various organisms exhibit adaptations that help them thrive in different gravitational environments. These adaptations are essential for survival and overall functioning. For example, certain plants have developed flexible structures to counterbalance the force of gravity.
- Root Systems: Plants orient their roots downward, anchoring them in the soil and allowing them to absorb water and nutrients effectively.
- Growth Patterns: Many species develop specific growth patterns to utilize gravitational pull, which can be seen in how they orient leaves or stems.
- Marine Life: Aquatic organisms, such as jellyfish and sea cucumbers, have adapted to minimize the effects of gravity. They possess buoyant properties that allow them to navigate without expending excessive energy.
In summary, gravity significantly influences biological systems, driving adaptations necessary for survival. By studying these effects, researchers can gain insights into health, development, and the potential for life in varying gravitational contexts.
"Understanding how gravity shapes biological systems opens new avenues for research, especially in fields like medicine and evolutionary biology."
"Understanding how gravity shapes biological systems opens new avenues for research, especially in fields like medicine and evolutionary biology."
This examination of gravity's impact on biological systems highlights its significance not just in scientific studies but also in practical applications across disciplines such as medicine and engineering.
Experimental Approaches to Understanding Gravity
Understanding gravity necessitates rigorous experimental methods that can validate or challenge existing theories. Experimental approaches are critical because they provide the empirical evidence required to substantiate theoretical frameworks and inform future research. Without experimental grounding, our understanding of gravity would remain speculative at best.
Research on gravity is unique as it spans numerous disciplines, from physics to astronomy. The insights gained from these experiments can enhance our grasp of the fundamental nature of gravity itself. This is particularly important in light of ongoing debates surrounding gravitational theories. It invites researchers to investigate not only traditional methods but also to embrace innovative, cutting-edge techniques.
Ground-based Experiments
Ground-based experiments have historically played a pivotal role in advancing our understanding of gravity. These experiments utilize instruments located on Earth to test gravitational theories and behaviors. For instance, the Kater's pendulum is one of the classic experiments that accurately measured the acceleration due to gravity by employing a simple pendulum setup. The results helped refine our understanding of gravitational acceleration ( 9.81 m/s² on Earth).
Moreover, recent advancements in technology allow for more precise measurements. Modern gravimeters, for example, can detect minute changes in gravitational force caused by shifts in mass distribution underground, such as tectonic movements or seasonal water reservoirs. This data is crucial in fields like geology and climatology, revealing how gravity serves as a useful tool for interpreting changes within the Earth.
Other innovative ground-based experiments include using laser interferometry to test the equivalence principle. Such setups enable researchers to detect extremely small changes in distances between two points, thus observing gravitational effects with high sensitivity. These methods underscore the importance of ground-based experimentation for testing between various theories within gravitational studies.
Space Mission Contributions
Space missions have significantly enhanced our experimental capabilities related to gravity. By placing instruments beyond Earth's atmosphere, researchers can mitigate many interference factors when studying gravitational forces. One of the remarkable achievements in this context is the Gravity Recovery and Climate Experiment (GRACE) missions. Launching in pairs, these satellites measure changes in Earth's gravity field over time, providing crucial insights into mass distribution changes related to climate change.
Another important mission is the European Space Agency's LISA Pathfinder. It aimed to test technologies necessary for the future of gravitational wave observatories in space. The project successfully demonstrated the feasibility of maintaining the required sensitivity and stability of measuring gravitational waves outside the Earth's atmosphere.
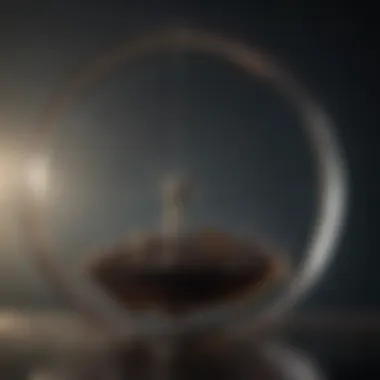
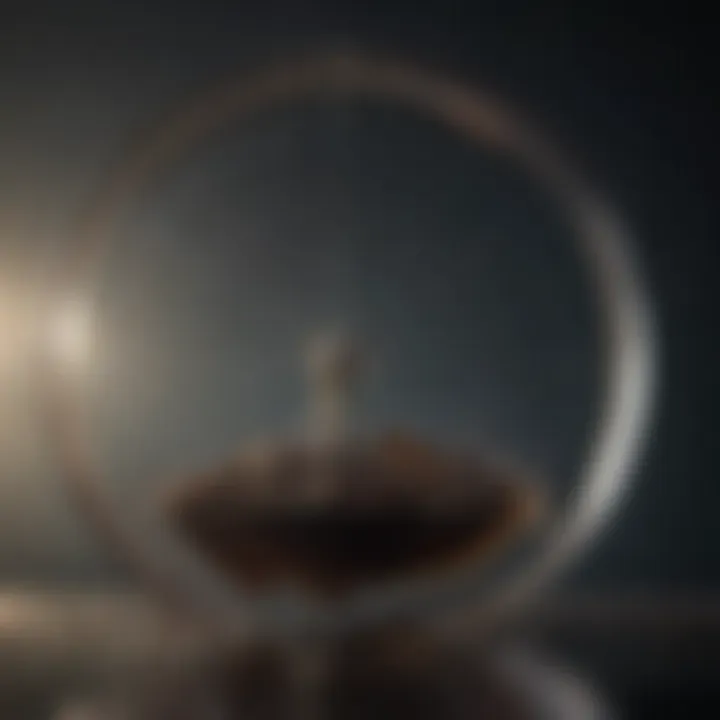
The findings from these space missions are invaluable for our understanding. By collecting data outside the constraints of our planet, researchers can observe cosmic phenomena that impact gravity, such as black holes and cosmic expansion. This sets a precedent for future studies exploring gravitational theories and their implications on a universal scale.
"Experimental approaches are vital in filtering through the theoretical noise, allowing us to advance our understanding of gravity beyond speculation."
"Experimental approaches are vital in filtering through the theoretical noise, allowing us to advance our understanding of gravity beyond speculation."
In summary, both ground-based experiments and space missions provide complementary insight into gravity research. They build a comprehensive framework through which we can investigate and refine our existing gravitational theories. As technology progresses, we can expect even more innovative approaches to emerge, leading science further down the path of understanding this fundamental force.
Current Theories Challenging Existing Paradigms
The investigation of gravity has historically focused on established frameworks, notably those proposed by Isaac Newton and Albert Einstein. However, as our understanding of the universe deepens, it has become clear that these classical models may not fully encapsulate all gravitational phenomena observed. This section highlights the importance of current theories that challenge existing paradigms, particularly in light of new astronomical data and experimental findings.
The development of alternative theories is vital for several reasons:
- Addressing Anomalies: There are persistent anomalies in gravitational observations that cannot be explained by traditional models. For instance, the rotation curves of galaxies display unexpected behavior that suggests the presence of unseen factors affecting gravity.
- Progression of Science: Science thrives on questioning established ideas. By exploring these new theories, researchers can foster advancements in both theoretical frameworks and practical applications.
- Interdisciplinary Approaches: Exploring alternative models encourages collaboration between various fields, such as cosmology, particle physics, and even philosophy, enhancing our overall understanding of gravity.
Modified Newtonian Dynamics (MOND)
Modified Newtonian Dynamics, or MOND, presents an interesting approach to the issue of galaxy rotation curves. It deviates from Newton's law of universal gravitation, proposing that at low accelerations, the familiar laws of motion do not apply as they do at higher accelerations. Instead, MOND posits that the gravitational force behaves differently under certain conditions. It offers potential explanations without invoking dark matter, a concept that remains elusive and debated by physicists.
The implications of MOND extend beyond astronomy; they challenge fundamental principles of physics. By modifying Newton's laws, proponents argue that MOND can explain observed phenomena while reducing the need for dark matter. Critics raise concerns about its predictive power and compatibility with other aspects of physics. The ongoing discourse surrounding MOND emphasizes the need for empirical validation and theoretical soundness in gravitational research.
Emerging Gravitational Theories
Several new gravitational theories are currently making waves in the scientific community. These theories aim to provide frameworks that can better account for observations that challenge traditional gravity concepts. They include:
- Tensor-Vector-Scalar Gravity (TeVeS): This theoretical model combines various gravitational influences to account for cosmological observations while remaining consistent with observed dynamics.
- Loop Quantum Gravity: This approach merges quantum mechanics with general relativity, attempting to describe gravity at the quantum level. It seeks to provide insights into the fabric of spacetime and the nature of gravity at microscopic scales.
- Emergent Gravity: This theory suggests that gravity is not a fundamental force, but rather emerges from the entropic behavior of microscopic degrees of freedom. This perspective shifts the understanding of gravity from a fundamental interaction to a macroscopic phenomenon.
Each of these theories is in various stages of development and scrutiny, but they all share a common goal: to explain the complexities of gravity in a universe that increasingly defies simple explanations. As research continues, the insights gained may not only reshape our understanding of gravity but also influence a broader range of scientific disciplines.
Future Research Directions in Gravity Studies
Future research in gravity studies is crucial for expanding our understanding of fundamental physics. This area of investigation not only addresses lingering questions but also has the potential to unlock new frontiers in both theoretical and experimental physics. As scientists pursue deeper insights into gravity, a multitude of elements and benefits arises, leading to significant implications for the scientific community and beyond.
Identifying New Phenomena
One of the main focuses in gravity studies revolves around identifying new phenomena that challenge existing theories. For instance, current research aims to observe gravitational interactions at different scales, particularly at quantum levels, where gravity's effects may behave differently from predictions. This involves meticulous experimentation and a fresh outlook on how gravity operates in various contexts. An example includes the search for dark matter interactions or studying anomalies in gravitational waves that could suggest new physics.
Exploring these anomalies could reshape our understanding of gravity and its role in the universe.
Exploring these anomalies could reshape our understanding of gravity and its role in the universe.
Scholars are also intrigued by the possibility of discovering exotic objects, such as primordial black holes, that might have formed in the early universe. These objects, if proven to exist, could act as a bridge between our understanding of gravity and the foundational aspects of cosmology. Identifying such phenomena warrants innovative approaches in both observational techniques and theoretical frameworks.
Technological Advancements in Research
The field of gravity studies benefits immensely from technological advancements. Improved technologies enhance our capability to measure gravitational effects with precision. For example, the use of laser interferometers in gravitational wave detection has opened the door to new experimental paradigms. Tools like LIGO and Virgo rely on advanced optics and control systems to detect disturbances caused by passing gravitational waves, demonstrating how technology can illuminate previously unseen aspects of gravity.
Additionally, ongoing developments in satellite technology contribute to better measurements of gravitational fields. The European Space Agency's GOCE (Gravity Field and Steady-State Ocean Circulation Explorer) mission revolutionized the way we observe Earth’s gravitational field, providing high-resolution maps that are critical for both scientific and practical applications.
In essence, increasing collaboration among different fields, such as astrophysics, quantum mechanics, and engineering, can yield richer insights into gravity's nature. It reinforces the idea that interdisciplinary approaches are vital for charting future directions in gravity studies.
Gravity in Popular Culture
The portrayal of gravity in popular culture holds significant relevance in today's discourse, providing insights into not only how we understand this fundamental force but also how it shapes our collective imagination. Popular media, including film, literature, and games, often takes liberties with the complexities of gravity while simultaneously influencing public interest and knowledge about this essential component of the universe.
This section discusses both the representation of gravity in media and its influence on the public perception of science. Through these insights, we can appreciate the cultural impact of scientific concepts and their intersections with everyday life.
Representation in Media
In various forms of media, gravity is frequently depicted as a dramatic element that can enhance narratives or provoke emotional responses. Films like Interstellar and Gravity explore themes of space travel and the intricacies of gravitational forces. These representations often feature visually stunning depictions of black holes, wormholes, and the curvature of spacetime. Such portrayals serve both educational and entertainment purposes. They not only captivate audiences but also provoke questions about the real nature of gravity and its implications in our universe.
It's noteworthy that while these representations are based on scientific principles, they usually simplify or exaggerate certain aspects for dramatic effect. Gravity focuses on the tension and isolation experienced by astronauts, allowing viewers to engage with the concept on a human level when, in reality, the nuances are far more complex. This balance between scientific truth and artistic license invites viewers to think critically about the realities of gravitational phenomena.
Influence on Public Perception of Science
The engagement of popular culture with scientific themes plays a pivotal role in shaping public perception. When gravity is depicted in accessible ways, it often sparks curiosity, admiration, and sometimes skepticism. This inquisitiveness can lead to increased interest in physics and astronomy among students and the general audience.
Many individuals derive their knowledge from films, documentaries, and even social media platforms like Reddit or Facebook. As a result, misconceptions sometimes arise. For example, the romanticized and simplified portrayal of phenomena can lead the audience to misunderstand critical elements of physics. However, it can also foster a desire for deeper understanding and further exploration of scientific concepts.
"Science is not only a disciple of reason but, also, one of romance and passion." – Stephen Hawking
"Science is not only a disciple of reason but, also, one of romance and passion." – Stephen Hawking
Finale
The topic of gravity is a cornerstone of our understanding of the universe. In this article, we explored how gravity influences not just physical science but also touches on areas like technology, biology, and even culture. The significance of understanding gravity cannot be understated. As we delve into its complexities, we uncover insights that illuminate not only the workings of celestial bodies but also the fundamental forces guiding our everyday existence.
Summary of Key Points
Key takeaways from our exploration include:
- Historical Context: Gravity has been understood differently throughout history, evolving from Newton's law to Einstein's theory of general relativity.
- Modern Applications: Gravity plays a pivotal role in technology, especially in the fields of satellite navigation and structural engineering.
- Biological Impact: The effects of gravity on human physiology manifest in various ways, from muscle atrophy in space to the adaptations of organisms in different gravity conditions.
- Future Research Directions: Emerging interest in quantum gravity theories and potential new phenomena could reshape our understanding of the universe.
By consolidating these points, we can appreciate the multifaceted nature of gravity.
Implications for Future Studies
Looking ahead, the implications for future studies on gravity are vast. Continued research holds the potential to uncover new physics that could redefine existing paradigms. As researchers develop technologies that provide more accurate measurements of gravitational waves or explore unconventional theories like Modified Newtonian Dynamics (MOND), we may soon be equipped to answer lingering questions about the cosmos.
In this context, interdisciplinary collaboration will be vital. Combining insights from physics, biology, and engineering can lead to novel approaches in understanding how gravity operates across scales. Furthermore, accessible communication about these developments will cultivate public interest and support for scientific endeavors.
As we continue to refine our understanding of gravity, we open doors to remarkable breakthroughs that not only expand our knowledge but also enhance our technological capabilities.