Exploring Polymeric Materials: Types and Applications
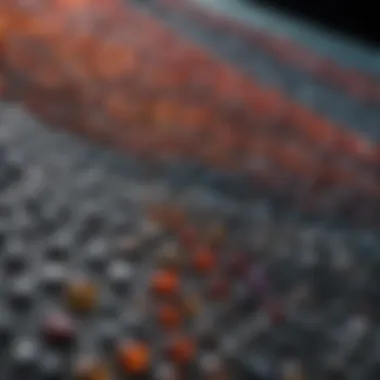
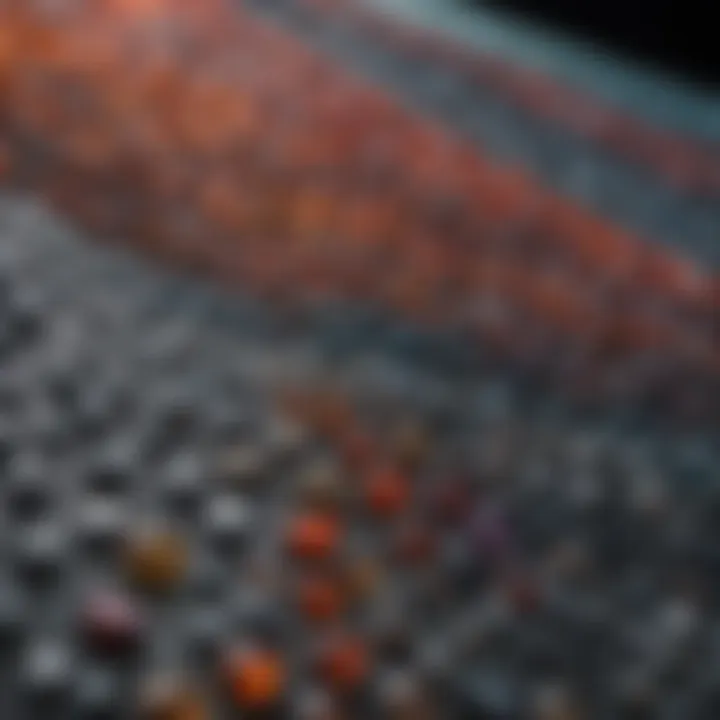
Intro
In recent years, the importance of polymeric materials has gained significant attention across multiple sectors. These materials play a crucial role in technology and our daily lives. To understand their impact better, one must explore their definitions, types, and practical applications. With advancements in technology, polymers are becoming increasingly versatile, leading to innovative solutions in various domains.
Key Concepts
Definition of the Main Idea
Polymeric materials are large molecules composed of repeating structural units known as monomers. The properties of polymers can greatly vary based on their composition and arrangement. They are found in both natural forms, such as cellulose, and synthetic forms, like polyethylene. This versatility allows them to be used in many applications, from everyday products to industrial solutions.
Overview of Scientific Principles
The behavior of polymeric materials is guided by several scientific principles. These include:
- Molecular Weight: Higher molecular weights typically lead to greater strength and durability.
- Cross-linking: This process influences the elasticity and thermal properties of the polymer.
- Glass Transition Temperature (Tg): Tg defines the temperature range where a polymer transitions from a brittle state to a more flexible one.
Understanding these principles is essential for professionals working with polymers, as they determine material performance in practical applications.
Current Research Trends
Recent Studies and Findings
Recent research has highlighted the potential of biopolymers as sustainable alternatives to traditional fossil fuel-based polymers. Scientists investigate materials like polylactic acid, which can be produced from renewable resources.
Another significant area of interest is the development of smart polymers that respond to environmental stimuli. These materials can change shape, color, or other properties based on temperature or pH changes.
Significant Breakthroughs in the Field
Several breakthroughs have emerged in polymer science, particularly in nanotechnology. Examples include:
- Creation of nanocomposites that enhance strength and thermal stability.
- Use of 3D printing technology for rapid prototyping and manufacturing of polymer-based products.
These advancements signify a shift towards more efficient and innovative applications of polymeric materials that can greatly influence future designs and usage.
"Polymers are not just materials, they are a gateway to innovation and sustainability in industry."
"Polymers are not just materials, they are a gateway to innovation and sustainability in industry."
Preamble to Polymeric Materials
The study of polymeric materials is significant for both academic and practical reasons. This section aims to introduce the fundamental aspects of polymers, which serve as the backbone of many modern technologies. By exploring polymeric materials, one can gain insight into their diverse forms and functionalities. The relevance of these materials across multiple industriesโranging from packaging to healthcareโcannot be overstated. Notably, polymeric materials are tailored for specific applications, thus altering how products are designed and manufactured.
Defining Polymeric Materials
Polymeric materials are large molecules known as polymers, which are formed by the repetition of smaller units called monomers. These materials can be classified based on their properties, processing methods, and applications. A few key characteristics include their ability to deform under stress and recover when the stress is removed, thus making them suitable for a variety of applications.
Polymers can be natural or synthetic. Natural polymers, such as proteins and cellulose, exist abundantly in nature. In contrast, synthetic polymers like polyethylene and polystyrene have been engineered to exhibit particular characteristics that benefit various industries. The classification is crucial for understanding the specific applications and processing techniques pertinent to each type.
Historical Context
The timeline of polymeric materials reflects their evolution alongside human innovation. The journey began in the 19th century, with the discovery of natural rubber and subsequently the first synthetic polymer: Bakelite, invented by Leo Baekeland in 1907. This marked a turning point in materials science, showcasing the potential to create entirely new products.
Since then, advancements have accelerated, particularly in the mid-20th century when World War II spotlighted the necessity for new materials in military applications. Rapid commercialization followed, with polymers seeping into everyday products such as containers, clothing, and medical devices. The contemporary landscape of polymeric materials is characterized by a continual push toward sustainability and performance optimization, shaping how we interact with the environment and technology.
Understanding the historical context helps appreciate the technological strides we now take for granted. The impact of polymeric materials on society is profound, altering our approach to manufacturing, product design, and environmental considerations.
Types of Polymeric Materials
The examination of types of polymeric materials is critical in understanding their diverse properties and applications. The classification of polymers allows us to appreciate the distinct attributes that make them suitable for various industries. From manufacturing to healthcare, each type offers specific benefits and challenges. This exploration also facilitates advancements in technology, promoting sustainable practices and innovation.
Thermoplastics
Thermoplastics are a crucial category of polymeric materials. These polymers can be melted and reshaped multiple times, making them highly versatile in production processes. The ease of processing thermoplastics contributes significantly to their popularity in the manufacturing sector. They are widely utilized in items ranging from common household products to intricate engineering applications.
Examples of Thermoplastics
The most notable examples of thermoplastics include polyethylene, polypropylene, and polystyrene. Each of these polymers displays unique characteristics. For instance, polyethylene is noted for its excellent chemical resistance and flexibility, which makes it a common choice for packaging materials. This characteristic facilitates its use in various applications, providing effective solutions in multiple industries.
Another example, polypropylene, is appreciated for its higher melting point and stiffness. This property is beneficial in automotive and consumer product applications, as it offers durability while remaining lightweight. The unique feature of thermoplastics is their ability to be recycled. This advantage speaks to the growing emphasis on environmental responsibility in production processes.
Thermal Properties of Thermoplastics
The thermal properties of thermoplastics are pivotal for their applications. These materials can withstand varying temperatures, influencing how they are used in different conditions. For example, polycarbonate displays high impact resistance and a considerable heat distortion temperature. This ensures its application in settings requiring high durability, such as safety glasses and automotive parts.
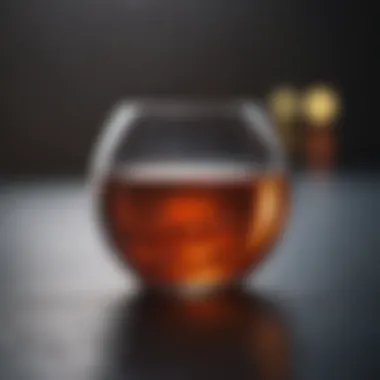
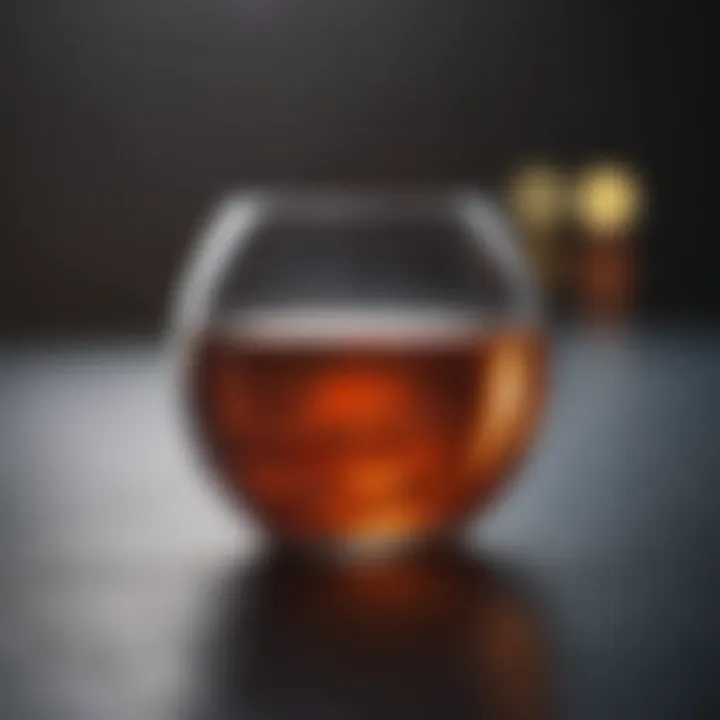
Thermal stability is a key characteristic of thermoplastics, allowing them to maintain structural integrity under heat. Nonetheless, while many thermoplastics can endure heat, some may deform when exposed to extreme temperatures. Thus, understanding their thermal properties is essential for appropriate material selection.
Thermosets
Thermosetting plastics exhibit a distinctive set of characteristics that render them suitable for specific applications. Once they are set into a shape, they cannot be remelted or reshaped, which provides excellent dimensional stability. The curing process involved in their production gives thermosets their durability and rigidity, making them popular in manufacturing processes that require lasting performance.
Characteristics of Thermosetting Plastics
Thermosetting plastics, such as epoxy and phenolic, are known for their high strength and thermal resistance. These characteristics are advantageous for applications involving structural components, electronics, and adhesives. Their ability to withstand heat and pressure enhances their usability in environments that demand resilience.
A unique feature of thermosets is their low shrinkage during curing, which results in precise dimensions for complex parts. However, the non-recyclability of thermosetting plastics poses challenges in waste management and sustainability efforts.
Common Applications
Common applications of thermosetting plastics include automotive parts, electrical insulations, and household appliances. Their durability and resistance to chemicals and heat make them ideal choices in these areas. The unique aspect of these applications is their long-term reliability, which ensures that products perform as expected over time. However, their inability to be reshaped can result in challenges when design modifications are needed.
Elastomers
Elastomers represent another essential category of polymeric materials. Their unique elasticity makes them particularly valuable for many applications. These polymers can stretch significantly and return to their original shape, which is critical for products requiring flexibility and resilience.
Properties of Elastomers
The properties of elastomers include high tensile strength and excellent elongation. This makes them suited for applications such as seals, gaskets, and tires. Their ability to withstand varying temperatures and mechanical stresses adds to their appeal. A critical characteristic of elastomers is their resistance to fatigue and wear, ensuring longevity in demanding environments. However, their susceptibility to degradation by certain chemicals can limit their usage in specific industrial sectors.
Uses in Various Industries
Elastomers find extensive usage across various industries, including automotive, healthcare, and consumer goods. In the automotive sector, they are used in tires and suspension components due to their excellent mechanical properties. In healthcare, elastomers are crucial in the production of medical devices and seals that require sterility and flexibility. Their ability to adapt to different applications underscores their importance in modern industry. While elastomers offer many benefits, their environmental impact raises concerns as advancements in sustainable alternatives are needed.
Key Examples of Polymeric Materials
Understanding specific examples of polymeric materials provides insight into their practical applications and essential roles in various sectors. The significance lies not only in their widespread use but also in their unique properties that make them suitable for certain tasks. Each category holds distinct benefits and considerations that influence their selection in industrial and consumer contexts.
Polyethylene
Properties and Characteristics
Polyethylene is one of the most commonly utilized polymeric materials. It is distinguished by its high flexibility, excellent chemical resistance, and durability. These properties contribute to its popularity across many industries. Notably, polyethylene can vary in density, yielding low-density polyethylene (LDPE) and high-density polyethylene (HDPE), each serving different purposes. This versatility allows manufacturers to choose the right type based on specific performance requirements. The ability to withstand moisture makes it suitable for packaging and containment applications.
Applications in Packaging
The role of polyethylene in packaging is paramount. Its lightweight nature and resistance to moisture are key advantages. These characteristics ensure that products remain safe and intact during transit. Polyethylene films are commonly used for food products, providing a barrier that helps to extend shelf life. However, its environmental impact, owing to non-biodegradability, raises concerns. Despite this, it remains a popular choice due to its performance benefits and cost-effectiveness.
Polypropylene
Industrial Applications
Polypropylene is recognized for its strength and rigidity. This polymer's resilience makes it suitable for various industrial applications, such as automotive components and packaging materials. Its resistance to chemical stress due to its non-polar structure enhances its utility in manufacturing processes. The lightweight nature paired with high tensile strength contributes to its widespread acceptance in industries looking for effective material solutions. However, the trade-off is its limited thermal stability.
Role in Consumer Goods
In the realm of consumer goods, polypropylene plays a vital role. This polymer is often found in products like containers and household items due to its affordability and durability. It holds an edge over other materials due to its ability to be molded into complex shapes, which is advantageous for manufacturers seeking design flexibility. Nonetheless, the recycling challenges associated with polypropylene can hinder its sustainability appeal.
Polystyrene
Uses in Construction
Polystyrene is valuable in the construction industry, particularly in insulation. Its lightweight characteristics and excellent thermal insulation properties make it an attractive option for enhancing energy efficiency. It can be produced in rigid forms, which are used in building panels, making it a durable choice for construction applications. A potential downside is that it can be vulnerable to certain solvents, which may limit its usage in specific environments.
Impact on Electronics
The impact of polystyrene on electronics is considerable. As an insulating material, it is used extensively in electronic components. Its low density and effective insulation properties contribute to creating lightweight and functional products. However, concerns regarding the flammability of polystyrene have led manufacturers to seek alternatives in some electronic applications. Despite this, its cost-effectiveness still makes it a viable choice.
Polyvinyl Chloride (PVC)
Versatility in Construction
PVC stands out for its versatility in the construction industry. Its ability to be crafted into both rigid and flexible forms allows it to be used in a variety of applications, from pipes to flooring. This adaptability offers architects and builders an extensive toolkit when designing infrastructure. However, the production processes for PVC can have a substantial environmental impact, raising sustainability questions.
Environmental Considerations
Environmental considerations regarding PVC present a mixed bag. On one hand, its long lifespan contributes positively to reducing waste; on the other hand, the chemical additives used in PVC can pose ecological risks. As the demand for sustainable construction materials increases, the recycling potential of PVC is under scrutiny. Developers must weigh the benefits against the environmental implications in their material selections.
"The selection of polymeric materials involves assessing various factors such as performance, cost, and environmental impact. Understanding these key examples aids in making informed decisions."
"The selection of polymeric materials involves assessing various factors such as performance, cost, and environmental impact. Understanding these key examples aids in making informed decisions."
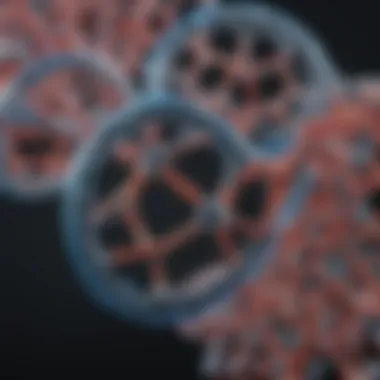
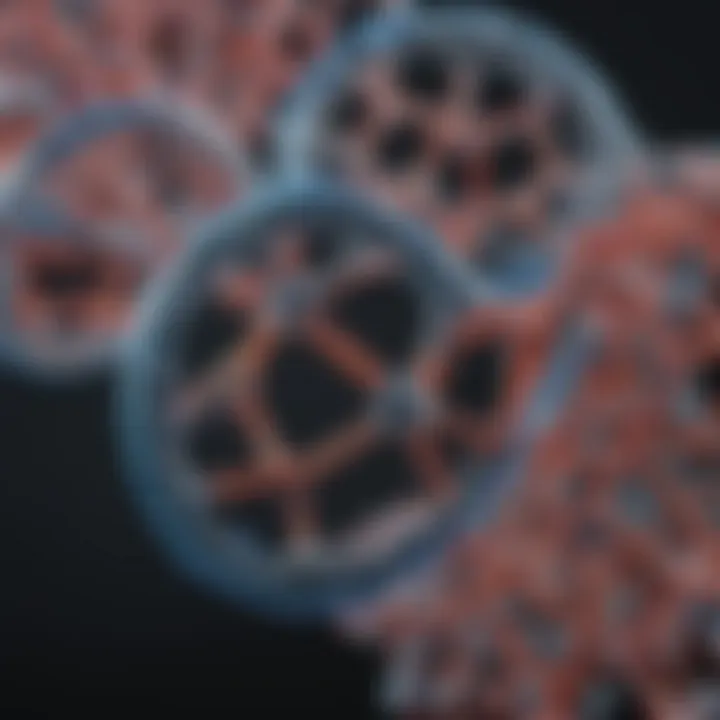
Biopolymeric Materials
Biopolymeric materials represent a crucial segment of polymeric materials, distinguished by their biodegradability and sustainability. As global concerns about environmental impact elevate, these materials gain attention for their potential to lessen waste and dependence on non-renewable resources. They offer a fascinating contrast to conventional polymers by leveraging biological sources for their production, aligning with ecological goals while providing utility across diverse applications.
Definition and Importance
Biopolymeric materials are defined as polymers derived from renewable biological resources. The significance of these materials lies in their dual ability to serve practical functions while contributing positively to environmental sustainability. By prioritizing materials that can return to nature without leaving a lasting footprint, biopolymers play a vital role in efforts to reduce plastic pollution and foster a circular economy. They ultimately represent a shift towards more responsible and eco-friendly production methods.
Natural Polymers
Natural polymers are foundational to the biopolymeric category, showcasing how native materials can be harnessed for modern uses. Two notable examples of natural polymers are cellulose and chitosan.
Cellulose
Cellulose is one of the most abundant natural polymers on Earth. Its structure consists of long chains of glucose molecules, providing high mechanical strength. This property makes cellulose a highly popular choice in applications such as paper and textiles.
- Key Characteristic: Cellulose is biodegradable, which means it can decompose naturally and help reduce landfill waste.
- Unique Feature: Its ability to absorb moisture allows for applications in biomedical fields as drug delivery systems and wound dressings.
- Advantages: The renewability of cellulose and its non-toxicity make it favorable compared to synthetic counterparts.
- Disadvantages: However, the processing of cellulose can be challenging, requiring extensive treatment to become usable in various applications.
Chitosan
Chitosan is another noteworthy natural polymer, derived from chitin, found in the shells of crustaceans. It is known for its biocompatibility and biodegradability, making it particularly relevant in healthcare and environmental applications.
- Key Characteristic: Chitosan possesses antibacterial properties, making it useful in wound healing and food preservation.
- Unique Feature: It can form hydrogels, which are valuable in drug delivery and tissue engineering.
- Advantages: The marine source of chitosan makes it sustainable, with potential to utilize by-products from the seafood industry.
- Disadvantages: Its solubility is limited to acidic conditions, which can restrict its application scope.
Synthetic Biopolymers
Synthetic biopolymers, engineered from renewable resources, offer another compelling aspect of biopolymeric materials. They include polylactic acid (PLA) and polyhydroxyalkanoates (PHA), both of which are notable for their versatility.
PLA (Polylactic Acid)
PLA is a prominent synthetic biopolymer produced from the fermentation of sugars. It has gained popularity due to its wide-ranging applications, particularly in packaging and 3D printing.
- Key Characteristic: PLA is biodegradable under industrial composting conditions, making it an attractive alternative to traditional plastics.
- Unique Feature: It can be processed similarly to petroleum-based plastics in manufacturing, presenting a smooth transition for industries.
- Advantages: Its sustainable production from cornstarch enhances its profile as an eco-friendly option.
- Disadvantages: However, PLA can have limitations in heat resistance, making it unsuitable for high-temperature applications.
PHA (Polyhydroxyalkanoates)
PHA is produced by microbial fermentation and represents a family of polyesters. This synthetic biopolymer is particularly valued for its biodegradability and versatility.
- Key Characteristic: PHAs can be processed into various forms, from films to fibers, allowing for multiple uses.
- Unique Feature: They are produced by microorganisms, which can utilize diverse organic substrates, providing an adaptable production method.
- Advantages: PHAโs inherent biocompatibility makes it ideal for medical applications, such as sutures and drug delivery systems.
- Disadvantages: The production cost remains high relative to conventional plastics, which can limit broader adoption.
Innovations in Polymeric Materials
Innovations in polymeric materials have significantly changed the landscape of multiple industries. These materials are not static; they are evolving with advancements in science and technology. The development of smart polymers and nanocomposites exemplifies this evolution. Understanding these innovations is crucial for leveraging the advantages they offer.
Smart Polymers
Definition
Smart polymers, or stimuli-responsive polymers, are materials that can change their properties when subjected to specific environmental conditions. This includes variations in temperature, pH, or electric and magnetic fields. The adaptability of smart polymers makes them a key topic in this article. Their main feature is the ability to respond to stimuli, which allows them to be used in dynamic applications. This adaptability is beneficial for their deployment in many sectors, particularly healthcare. However, the complexity in their design can be a challenge, impacting the cost and scalability of their production.
Applications in Healthcare
In healthcare, smart polymers are used in drug delivery systems and tissue engineering. This involves creating materials that release medications in a controlled manner, enhancing efficacy and reducing side effects. The key characteristic of these applications is their tailored response to physiological conditions, which ensures that drugs are delivered effectively. Smart polymers not only improve treatment outcomes but also facilitate personalized medicine.
Despite their promise, implementing these materials can be difficult. Regulatory hurdles and higher production costs can limit their widespread use. Nevertheless, their potential to transform healthcare and improve patient outcomes clearly delineates them as vital components of modern medicine.
Nanocomposites
Definition and Benefits
Nanocomposites are polymer blends that include nanoparticles to enhance the properties of the base material. By incorporating tiny particles, typically less than 100 nanometers, nanocomposites demonstrate improved mechanical strength, thermal stability, and electrical conductivity. The key aspect that makes nanocomposites popular in this article is their ability to combine the desirable features of both organic and inorganic materials. Their unique composition often leads to materials that are lighter and stronger than traditional polymers.
However, challenges exist regarding the uniform dispersion of nanoparticles within the polymer matrix. It can be a complex process that requires specific techniques and conditions to ensure optimal performance.
Use in Electronics
In the electronics field, nanocomposites are increasingly utilized for producing lighter and more efficient materials. Their enhanced electrical properties make them ideal for applications ranging from flexible displays to advanced batteries. Notably, the key characteristic that benefits electronics is conductivity. These materials reduce weight while increasing performance, which is crucial as devices become smaller and more compact.
While nanocomposites present several advantages, they also face challenges, such as the cost of production and potential environmental impacts associated with nanoparticle handling. Despite these concerns, the innovative nature of nanocomposites signal their ongoing relevance in the evolving landscape of electronics and material science.
Nanocomposites continue to exemplify how interdisciplinary approaches can lead to groundbreaking advancements in materials science.
Nanocomposites continue to exemplify how interdisciplinary approaches can lead to groundbreaking advancements in materials science.
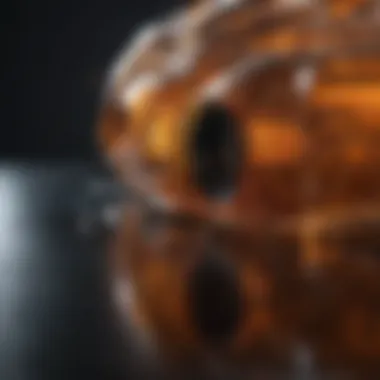
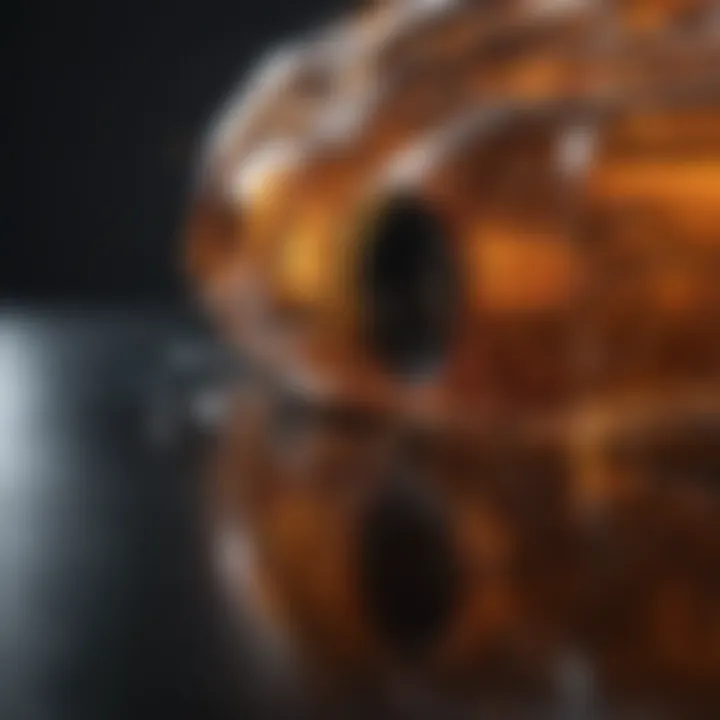
Environmental Impact of Polymeric Materials
The significance of understanding the environmental impact of polymeric materials cannot be overstated. As their usage pervades various industries, the consequences of such widespread application must be examined. Polymeric materials have durable properties, which contribute to their popularity, but these same qualities can make them challenging when it comes to waste management and environmental sustainability.
Waste Management Challenges
One of the most pressing issues when discussing polymeric materials is waste management. The long lifespan of many polymers means they persist in the environment for hundreds of years if not properly disposed of. This poses a serious challenge for waste management systems.
- Efficient recycling of polymers is limited due to the complex structures of some materials. Sorting different types of plastics can be labor-intensive and costly.
- Landfills are often overwhelmed by the sheer volume of plastic waste. This can lead to pollution of soil and waterways.
- Incineration is one method used to reduce waste, but it has environmental drawbacks, contributing to air pollution and greenhouse gas emissions.
These factors highlight the critical need for better waste management practices tailored to polymeric materials.
Biodegradable Polymers
The exploration of biodegradable polymers offers a avenue toward tackling waste issues. Biodegradable polymers are designed to break down more efficiently under certain conditions, which can minimize their environmental footprint.
Role in Reducing Waste
Biodegradable polymers serve an essential role in reducing waste. Their ability to decompose naturally means they can lessen the burden on landfills. Key characteristics include:
- Chemical Composition: Made from renewable resources, they are less harmful to the environment compared to traditional plastics.
- Natural Decomposition: When exposed to biological agents like bacteria and fungi, these materials break down within a much shorter time frame.
This feature is appealing as it reduces long-term pollution. However, challenges persist. Some biodegradable polymers require specific conditions to decompose effectively, making their disposal complex.
Case Studies
Examining case studies offers insight into the practical impacts of biodegradable polymers. For instance, certain packaging companies have turned to polylactic acid (PLA) as a sustainable alternative to conventional plastics.
- Adoption Rates: The shift towards PLA showcases growing awareness and demand for environmentally friendly materials.
- Comparative Analysis: Successes and failures in various applications highlight the importance of comprehensive understanding of the materials used.
Case studies demonstrate both the potential and limitations of biodegradable polymers in a real-world context, an essential element for informed decision-making in the industry. With continued research and innovation, the effectiveness of these materials can improve, providing valuable solutions to current waste management issues.
Future Directions for Polymeric Materials
The future of polymeric materials holds significant implications for various sectors, especially considering current challenges such as sustainability and technological advancement. This section addresses upcoming trends that will shape the research, development, and application of these materials. Notably, there is rising interest in enhancing the performance and functionality of polymers while considering their environmental impact. Understanding the evolving landscape of polymer science is critical, as it not only affects material choices but also influences industries ranging from healthcare to renewable energy.
Trends in Research and Development
Research in polymer materials is increasingly focusing on sustainability and multifunctionality. Scientists are exploring bio-based materials and processes that reduce dependence on fossil fuels. Innovative chemistries, such as those involving bio-polyethylene or functional additives, are gaining traction. The merging of polymers with nanotechnology is a notable trend, leading to products with enhanced mechanical, thermal, and barrier properties. These developments are aimed towards creating more efficient materials that cater to both consumer needs and environmental sustainability.
Potential Applications
In Renewable Energy
The role of polymeric materials in renewable energy is pivotal. They are increasingly used in solar panel manufacturing and energy storage devices, such as batteries. One key characteristic of these polymers is their lightweight nature, allowing for more efficient energy capture and transport. The integration of polymers enhances the flexibility and durability of solar modules, making them a popular choice for modern power solutions. Additionally, their ability to be produced from renewable resources aligns with the goals of reducing carbon footprints. However, challenges remain, such as the long-term stability of polymer-based systems under extreme conditions.
In Medical Devices
Polymeric materials are fundamental in the healthcare sector, particularly in the design of medical devices. Their versatility and biocompatibility make them ideal for applications ranging from drug delivery systems to prosthetics. A prominent characteristic of medical-grade polymers is their capacity to mimic biological tissues, which is essential for developing implants and other internal devices. This adaptability allows for better integration with the body and reduced risk of rejection. Furthermore, the ability to customize properties through various polymer compositions allows for an extensive range of medical uses. Nonetheless, regulatory considerations and the potential for wear over time are critical factors that require ongoing research to ensure safety and effectiveness.
"Polymers shape the future of technology and sustainability, addressing both current and emerging needs in innovative ways."
"Polymers shape the future of technology and sustainability, addressing both current and emerging needs in innovative ways."
Conclusively, these future directions illustrate the dynamic nature of polymeric materials and their broad spectrum of applications. As research progresses, it will be important to continue exploring both their potential and the ramifications of their use in a rapidly advancing world.
End
In this article, we have explored the complex landscape of polymeric materials. These materials hold a significant position in modern technology and various industries. Their versatility and diverse applications have driven innovations across sectors. The conclusion draws together the many aspects of polymers, highlighting their properties, examples, and potential future developments.
Understanding the range of polymeric materials is crucial for students, researchers, and professionals. Polymers play a vital role in everyday products as well as advanced applications. They provide solutions that are often more efficient, sustainable, and affordable compared to alternatives. This article presents key insights into types of polymers like thermoplastics and elastomers, alongside biopolymeric innovations and their environmental implications.
"Polymers have revolutionized industry and daily life, providing solutions that integrate function and sustainability."
"Polymers have revolutionized industry and daily life, providing solutions that integrate function and sustainability."
The significance of polymers goes beyond their physical properties. They contribute to shaping future technologies. With advances in research and applications, each technology utilizing polymeric materials builds towards a more sustainable future. The integration of smart polymers and biodegradable options is essential in addressing environmental concerns.
Summary of Key Points
This section encapsulates the essential knowledge shared in the article:
- Polymeric materials are essential in many sectors.
- They fall into several categories: thermoplastics, thermosets, elastomers, and biopolymers.
- Each category has distinct properties and applications.
- Understanding these materials is vital for innovation.
- Ongoing research focuses on sustainable practices and advanced applications in healthcare and renewable energy.
Final Thoughts on Polymeric Materials
The future of polymeric materials is bright and promising. Continued advancements in polymer science will lead to the development of new materials. Understanding the implications of these materials on our environment will be increasingly important. As challenges in waste management and sustainability grow, innovative polymer solutions can help mitigate these issues.
The study of polymeric materials is not just academic; it is a preview of the future of technology and environmental responsibility. Embracing these materials and their impacts is key for anyone involved in science, engineering, or industry.
In summary, polymeric materials are indispensable in our modern society, and their study can contribute to a more sustainable and efficient future.