WES Analysis: A Comprehensive Exploration of Genomics
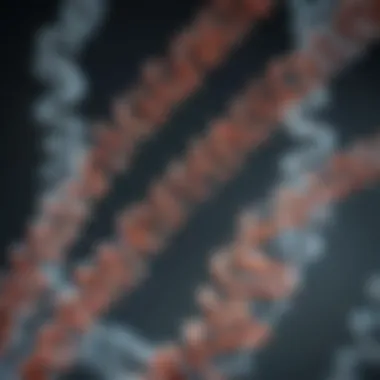
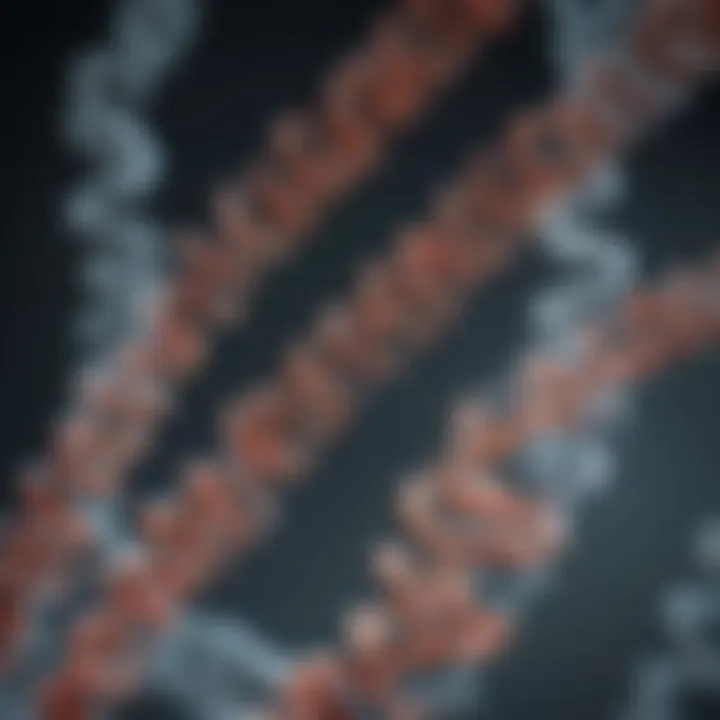
Intro
Whole Exome Sequencing (WES) is a cutting-edge technique that allows scientists to analyze the exons of genes in a genome. By focusing on these regions, which represent only about 1-2% of the entire genome but encompass around 85% of known disease-related variants, WES provides valuable insights into genetic disorders. This article aims to thoroughly explore WES analysis, looking at its methodologies, applications, and the implications of findings in the context of modern genomics.
A significant driving force of genomic research today is the quest for understanding how genetic variations influence health and disease. WES has emerged as a powerful tool in this endeavor, enabling the identification of mutations that may contribute to various ailments. With a deeper comprehension of WES, students, researchers, educators, and professionals can leverage this knowledge to drive advancements in personalized medicine and improve patient outcomes.
Key Concepts
Definition of the Main Idea
Whole Exome Sequencing is a method primarily engaged to target exons, the coding regions of genes. The process involves several steps, including sample collection, DNA extraction, library preparation, sequencing, and data analysis. In this analysis, researchers can pinpoint specific genetic variants that may play roles in disease development.
Overview of Scientific Principles
WES capitalizes on next-generation sequencing technology, which allows for rapid and cost-effective sequencing of genetic material. The workflow generally involves:
- Sample Collection and DNA Extraction: Blood or saliva samples are collected to extract genomic DNA.
- Library Preparation: The extracted DNA is fragmented, and specific exons are captured and enriched using various biological techniques.
- Sequencing: High-throughput sequencers then read the captured exons. Each sequencer may use slightly different technologies and platforms, such as Illumina NovaSeq or Thermo Fisher Ion Proton.
- Data Analysis: Computational tools are used to assemble and interpret the sequence data.
The output from these processes yields a comprehensive list of genetic variants, which can then be further investigated for their potential role in various health conditions.
"WES analysis has revolutionized the field of genetics, allowing for unprecedented insights into the connections between genotypes and phenotypes."
"WES analysis has revolutionized the field of genetics, allowing for unprecedented insights into the connections between genotypes and phenotypes."
Current Research Trends
Recent Studies and Findings
In recent years, WES has been pivotal in uncovering genetic underpinnings of many diseases, including rare genetic disorders and certain cancers. Several studies highlight its capacity to identify novel genetic mutations and elucidate their clinical significance. For instance, large-scale WES analyses of individuals with Mendelian disorders have led to the discovery of many previously unrecognized genetic causes.
Significant Breakthroughs in the Field
Notable breakthroughs include:
- The identification of BRCA1 and BRCA2 mutations in breast and ovarian cancers, which have profound implications for screening and treatment strategies.
- Advances in understanding the genetic basis of autism spectrum disorders, revealing multiple loci associated with the condition.
- The development of WES into a routine diagnostic tool in clinical settings, illustrating its potential in real-world applications.
As WES continues to refine our understanding of genetic variability, the future of gene-focused therapies seems increasingly promising.
Prolusion to WES Analysis
Whole Exome Sequencing (WES) has emerged as a crucial technique in the field of genomics. It focuses on sequencing the exonic regions of the genome, which contain the coding sequences of genes. This approach allows for a targeted exploration of genetic variations that can be linked to various diseases. Understanding WES is fundamental for researchers, clinicians, and students who delve into the complexities of genetic data.
The importance of WES lies in its ability to provide insight into the genetic underpinnings of health and disease. Unlike whole-genome sequencing, which examines the entire DNA sequence, WES zeroes in on regions that are more likely to have functional implications. This makes it a cost-effective choice and speeds up the process of identifying potential pathogenic variants.
Furthermore, WES is increasingly recognized for its role in personalized medicine, where understanding individual genetic profiles can lead to more tailored treatment options. By focusing on exomes, scientists can pinpoint critical mutations that contribute to disease processes.
Understanding the Basics
At the basis of Whole Exome Sequencing is the concept of targeting the exons, approximately 1-2% of the entire genome. These regions are significant because they code for proteins, which play essential roles in cellular functions. The exome is rich in information pertinent to various health conditions, including cancers and genetic disorders.
WES involves several steps: sample collection, library preparation, sequencing, and data analysis. During sample collection, DNA is extracted from tissues or cells. In library preparation, the DNA is fragmented, and exonic regions are selectively enriched. This enrichment allows for focused sequencing, which is less data-intensive and more efficient.
The sequencing process itself can be performed using high-throughput platforms. Technologies from companies like Illumina or Thermo Fisher Scientific have revolutionized how we approach sequencing. After sequencing, data analysis begins, which requires robust bioinformatics tools to interpret the large volumes of data generated.
Significance in Genomic Research
WES has greatly impacted genomic research by enabling scientists to explore the genetic basis of diseases with greater precision. It has facilitated the discovery of mutations linked to various conditions, from rare genetic disorders to common diseases like diabetes and heart disease.
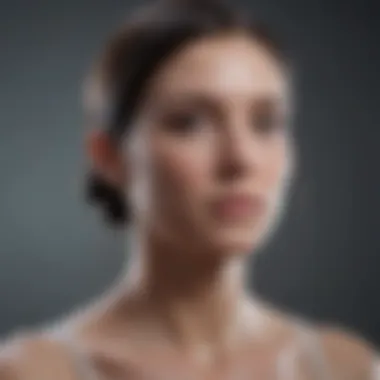
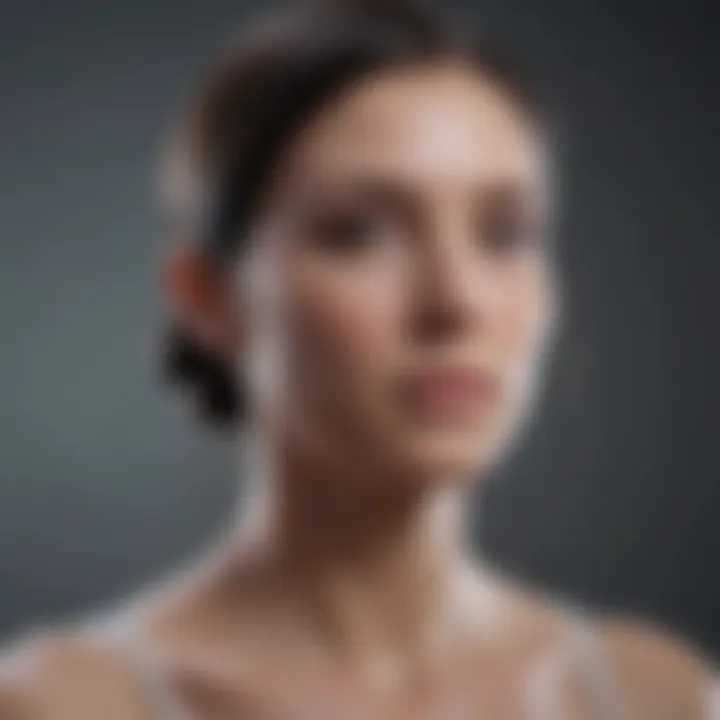
The ability to identify these mutations helps in several key areas:
- Disease Diagnosis: WES can uncover genetic variations that might not be evident through traditional diagnostic methods.
- Cancer Genomics: Tumor profiling using WES allows researchers to understand the genetic changes that promote tumorigenesis. This can inform treatment choices and patient management.
- Pharmacogenomics: Understanding how genetic variations affect drug metabolism can help customize patient care, ensuring effective therapy tailored to individual genetic make-up.
Additionally, WES contributes to large-scale population studies. By examining genetic variations across diverse groups, scientists can discern patterns that inform our understanding of evolutionary biology and susceptibility to diseases. This kind of research helps address public health issues by linking genetic factors to disease prevalence in specific populations.
The Methodology of WES
The methodology of Whole Exome Sequencing (WES) is integral to harnessing the power of genomic analysis in modern medicine. By focusing on the exome, which constitutes only about 1% of the human genome, WES provides a cost-effective means of identifying genetic variations related to diseases. This section explores various components of the methodology, including sample collection and preparation, library construction techniques, sequencing platforms, and data processing pipelines.
Sample Collection and Preparation
The first step in WES analysis is the collection of biological samples. These samples can come from various sources such as blood, saliva, or tissue biopsies. The choice of sample type often depends on the clinical question posed and the availability of material. Proper collection and handling of samples are crucial to ensure the integrity and quality of DNA extracted. Furthermore, it is vital to obtain informed consent from participants, addressing any ethical considerations.
Once samples are collected, DNA extraction follows. The extraction method can vary, but common techniques include silica-based columns or magnetic beads. These methods efficiently isolate the DNA while minimizing degradation. Quality control at this stage is essential, as the purity and concentration of the DNA will impact downstream applications. Techniques like gel electrophoresis or spectrophotometry are often employed to assess DNA quality before proceeding to library construction.
Library Construction Techniques
Library construction involves preparing the DNA for sequencing. This process typically consists of several steps, including fragmentation, adapter ligation, and amplification. During fragmentation, the DNA is broken into smaller pieces, often using mechanical methods or enzymatic digestion. This step is crucial, as the size of the fragments can influence the sequencing results.
After fragmentation, specific DNA adapters are ligated to the ends of the fragments. These adapters are necessary for sequencing, as they contain sequences needed for binding to the sequencing platform and include indices for multiplexing. Amplification can be performed with techniques like PCR, which increases the quantity of DNA available for sequencing.
Key Considerations:
- Fragment size: Different sequencing technologies might favor different fragment sizes.
- Adapter design: Proper adapter design is crucial for optimal sequencing performance.
Sequencing Platforms
The choice of sequencing platform is a critical aspect in the WES methodology. Several platforms are commercially available, each with its advantages and limitations. Popular platforms include Illumina, Ion Torrent, and PacBio, among others.
Illumina's sequencing technology is notable for its high-throughput and accuracy. It works well for exome sequencing due to its ability to generate a large amount of data quickly. Ion Torrent, on the other hand, offers a faster and simpler setup, making it suitable for smaller studies or clinical environments. PacBio provides long-read sequencing capabilities which can be beneficial in certain applications, such as detecting structural variants or resolving complex regions of the genome.
When selecting a sequencing platform, researchers must consider factors like:
- Read length: Longer reads might be necessary for particular applications.
- Throughput capabilities: Depending on the number of samples being processed concurrently.
Data Processing Pipelines
Once the sequencing is completed, data processing is the next phase in WES analysis. This step involves several computational processes to turn raw sequencing data into interpretable genetic information.
Data processing pipelines typically include stages such as demultiplexing, alignment, variant calling, and annotation. In demultiplexing, the sequenced data is sorted based on the unique indices attached during library construction, allowing for the identification of different samples in pooled sequencing runs.
Alignment is the next critical step, where the sequenced reads are matched to a reference genome. This process requires sophisticated algorithms to account for sequencing errors and variations among the samples.
Following alignment, variant calling determines the differences between the aligned reads and the reference genome. Various tools, such as GATK or FreeBayes, are often utilized for this purpose. Finally, annotation places functional context around identified variants, providing insights into their possible clinical significance.
"Data processing is a complex, yet vital step in turning raw sequencing data into valuable insights in genomic research."
"Data processing is a complex, yet vital step in turning raw sequencing data into valuable insights in genomic research."
Through understanding the methodology behind WES, researchers can better appreciate the advantages of this technology in the context of genetic research and personalized medicine.
Applications of WES Analysis
Whole Exome Sequencing (WES) represents a significant advancement in genomic research, providing insights that extend across numerous fields. The applications of WES analysis are vast, underscoring its role in diagnosing genetic diseases, advancing cancer genomics, informing pharmacogenomics, and exploring population genetics. Each of these applications addresses specific questions about genomic variations and their implications, enriching the collective understanding of human health and disease.
Genetic Disease Diagnosis
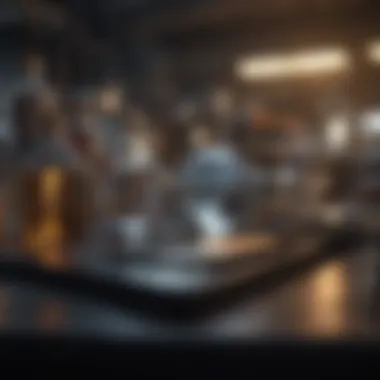
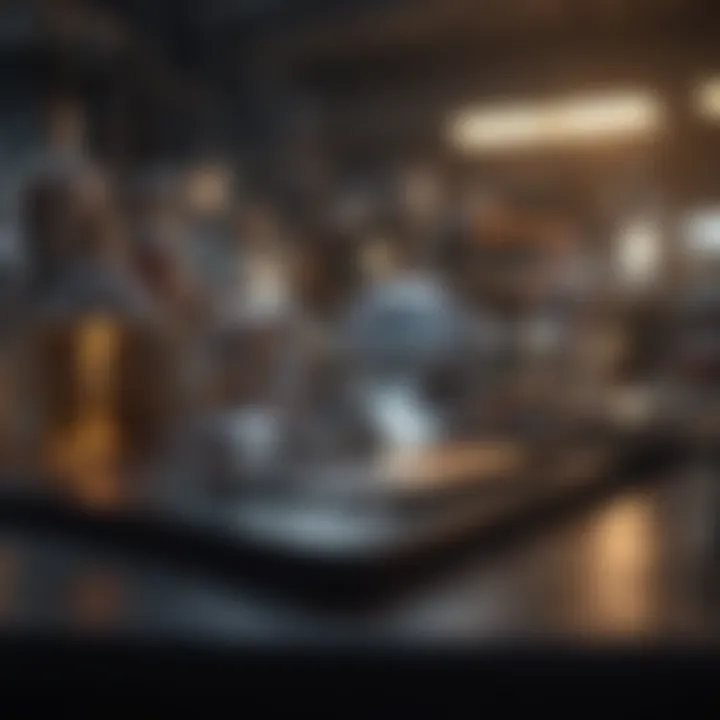
WES has become a pivotal tool in the diagnosis of genetic disorders. Unlike traditional sequencing methods that may cover only a small fraction of the genome, WES focuses on the exome, which consists of coding regions that are critical for understanding genetic diseases. This approach has proven immensely valuable in identifying pathogenic variants associated with rare disorders. The ability to detect these variants can facilitate clinical decisions and personalize patient care.
In cases where a patient presents with unexplained symptoms, WES can illuminate previously unrecognized genetic causes. Studies demonstrate that the diagnostic yield of WES can reach up to 30% in patients with suspected genetic disorders. By accurately pinpointing genetic anomalies, healthcare providers can better inform patients and families, offering clearer prognoses and targeted therapy options.
Cancer Genomics
In the realm of oncological research, WES allows for a deeper understanding of tumor biology. By sequencing the exomes of both tumor and normal tissues, researchers can identify somatic mutations that drive cancer progression. This information is crucial as it can lead to the development of targeted therapies tailored to specific mutations.
Cancer is a heterogeneous disease, and WES sheds light on the unique genetic landscape of individual tumors. Understanding these variants can help predict patient responses to treatment, ultimately guiding oncologists in making informed choices regarding therapy. Publications have noted that WES contributes significantly to biomarker discovery, paving the way for breakthroughs in personalized medicine for oncology.
Pharmacogenomics
Pharmacogenomics utilizes WES to understand how genetic variations affect individual responses to drugs. This knowledge can enhance precision medicine, allowing doctors to customize treatment plans based on a patient's genetic makeup. Adverse drug reactions and inefficacy can be minimized by selecting medications that align with a patient's unique genetic profile.
WES enables researchers to identify specific genetic variants that influence drug metabolism and efficacy. Consequently, patients may experience better therapeutic outcomes, reducing the trial-and-error aspect of medication management. This application highlights the relevance of WES in improving drug development processes and establishing guidelines for personalized therapy.
Population Genetics Studies
Population genetics benefits greatly from WES as it provides insights into genetic diversity within and between populations. This application helps elucidate human evolutionary history by examining patterns of genetic variation. WES can reveal how certain variants confer advantages or predispose individuals to diseases, contributing to our understanding of human adaptation.
Moreover, WES has implications for public health. By analyzing genetic data from diverse populations, scientists can identify risk factors and develop strategies for disease prevention. This application also underscores the importance of inclusivity in genomic studies, aiming to ensure all populations are represented in genetic research.
By understanding the applications of WES analysis, researchers and clinicians can leverage this technology to address various health challenges, ultimately improving patient outcomes and enriching scientific discovery.
By understanding the applications of WES analysis, researchers and clinicians can leverage this technology to address various health challenges, ultimately improving patient outcomes and enriching scientific discovery.
Interpreting WES Data
Interpreting data from Whole Exome Sequencing (WES) is a critical component in genomics research. The accuracy of interpretation directly influences the utility of WES in clinical and research settings. As WES generates vast amounts of data, effectively analyzing and interpreting this data can be challenging yet essential for deriving meaningful biological insights.
Variant Calling Techniques
Variant calling is the initial step after sequencing. This process involves identifying differences between the sequenced sample and a reference genome. Common techniques used in variant calling include alignment algorithms such as BWA (Burrows-Wheeler Aligner) and GATK (Genome Analysis Toolkit). Each technique has its unique approach to aligning reads and identifying variants. BWA is known for its speed and efficiency, while GATK is widely used for its comprehensive toolkit that offers variant calling alongside filtering and annotation.
It's important to ensure accurate variant detection as false positives or negatives can mislead further analyses and interpretations. The output from these tools typically includes SNPs (single nucleotide polymorphisms) and indels (insertions and deletions), which need to be carefully reviewed and validated.
Annotation of Variants
Once variants are called, the next step is annotation. Variant annotation provides context about the biological significance of identified changes. This involves linking variants with information from genomic databases such as dbSNP, ExAC, or ClinVar.
Annotation helps in classifying variants based on their potential impact on the gene function. For example:
- Benign variants: Often seen in populations, not associated with disease.
- Pathogenic variants: Linked to specific diseases, requiring further clinical attention.
- Uncertain significance: Variants with unclear implications.
Effective annotation is essential for prioritizing variants for potential clinical relevance, guiding researchers and clinicians in identifying which variants to investigate further.
Clinical Relevance of Variants
The final step in interpreting WES data is assessing the clinical relevance of the identified variants. Variants that can cause genetic disorders or influence drug response are of key interest. With the right interpretation, clinicians can make informed decisions regarding patient management and therapeutic strategies.
Integrating variant data with clinical features forms the basis for precision medicine. This approach tailors treatment based on individual genetic profiles. For example, pharmacogenomic findings in drug metabolism can impact which medications are safely prescribed to patients.
In summary, interpreting WES data requires a multi-faceted approach including rigorous variant calling, thorough annotation, and a focus on clinical implications. The outcome of this process can significantly impact patient care and drive further genomic research.
Challenges in WES Analysis
Whole Exome Sequencing (WES) analysis is a transformative evolution in the field of genomics. However, it is accompanied by a multitude of challenges that impact both research and practical applications. Understanding these challenges is crucial for students, researchers, and professionals engaged in genomic studies. Each of the elements discussed in this section illuminates significant hurdles that can affect overall outcomes in WES analysis.
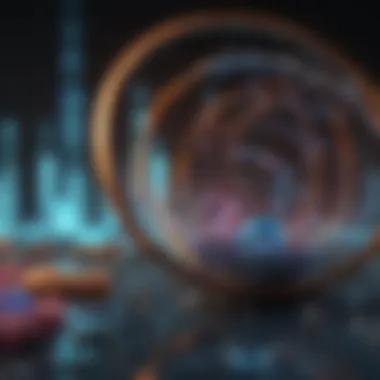
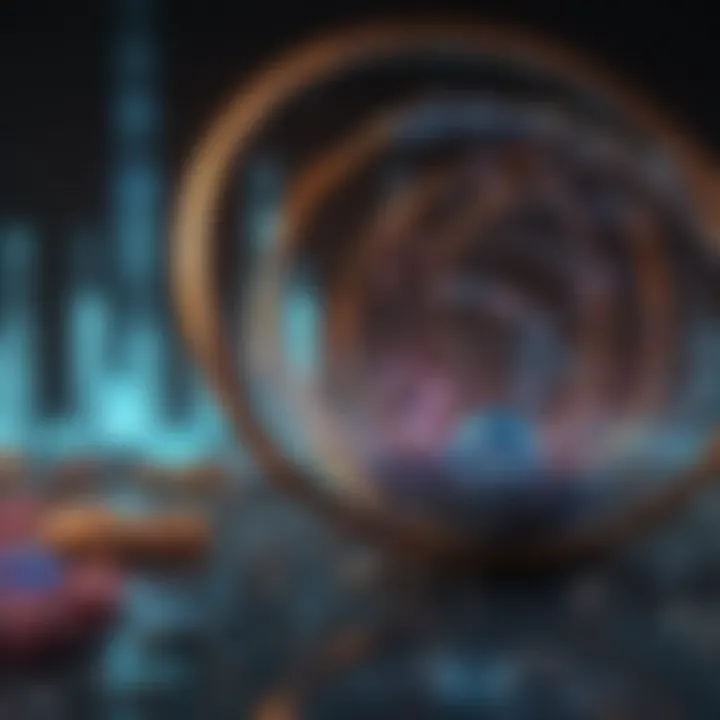
Data Complexity and Volume
Data complexity and volume represent a fundamental challenge in WES analysis. The sheer amount of data generated during sequencing can overwhelm both computational resources and researchers themselves. With each sample producing millions of reads, systems require advanced algorithms for effective storage, analysis, and interpretation.
- Storage Needs: High-performance computing resources are essential to accommodate the large datasets. Researchers must invest in storage solutions that are both scalable and efficient.
- Processing Overheads: Data processing entails multiple stages including alignment, variant calling, and filtering, each with its own computational demands. Errors in early processing stages can cascade into significant interpretive issues later.
The complexity of the data also arises from the variation present within the human genome. Distinguishing between benign variants and those contributing to disease remains a daunting task.
Interpretive Challenges
Interpretive challenges in WES revolve around accurately analyzing and gleaning actionable insights from the data collected. While advances in bioinformatics have improved variant identification and classification, nuances still complicate the interpretation process.
- Variant Classification: The categorization of genetic variants requires extensive databases and expert knowledge. Variants may be classified as benign, likely benign, uncertain significance, likely pathogenic, or pathogenic, introducing uncertainty into clinical decisions.
- Computational Models: Algorithms used for variant prediction are not infallible. The incorrect interpretation of variants can lead to inappropriate treatment decisions.
These interpretative challenges are essential for establishing clear links between genetic variations and clinical outcomes, making it critical for researchers to be well-versed in data interpretation methodologies.
Ethical Considerations
Ethical considerations are paramount in WES analysis. The potential misuse of genetic data poses significant risks to individual privacy and consent. As genomic data becomes more accessible, ethical issues surrounding data ownership and usage come to the forefront.
- Informed Consent: Acquiring informed consent from individuals undergoing genetic testing is crucial. Participants should understand how their data will be used and the implications of the findings.
- Privacy Issues: Protecting sensitive genetic information from unauthorized access is essential. In the event of data breaches, the consequences can be severe, both for individuals and research institutions.
- Societal Implications: The societal impact of genetic findings must be evaluated, particularly regarding discrimination in employment or insurance based on genetic predispositions.
Future Directions in WES Analysis
As Whole Exome Sequencing (WES) continues to evolve, understanding its future directions is crucial. This section highlights several important elements that will shape the future landscape of WES analysis. The implications of emerging technologies, integration with other genomic data types, and the shift towards personalized medicine are essential components in comprehending WESโs potential in modern research and clinical settings.
Technological Advancements
Technological advancements play a vital role in the progression of WES analysis. New sequencing technologies are emerging at a rapid pace, allowing for faster and more accurate data collection. For example, improvements in sequencing platforms such as Illumina NovaSeq and Pacific Biosciences are notable. These advancements can lead to a decrease in costs while increasing throughput, providing researchers access to more samples in a timely manner.
Furthermore, bioinformatics tools are becoming increasingly sophisticated. Enhanced algorithms can now handle massive datasets more efficiently. Tools such as GATK (Genome Analysis Toolkit) are crucial in variant calling and may provide real-time analysis capabilities. These technological shifts promise to improve the accuracy of WES and expand its applications in various fields, from clinical diagnostics to population genomics.
Integration with Other Genomic Data
The integration of WES data with other genomic information is becoming essential. Combining Whole Exome Sequencing with Whole Genome Sequencing (WGS) offers a more comprehensive view of genetic variations. WGS provides insights beyond the exome, including regulatory regions and intronic sequences that WES cannot capture. This holistic approach can improve variant interpretation, revealing new aspects of genetic contributions to diseases.
Moreover, integrating WES findings with transcriptomic data can shed light on how genetic variations affect gene expression and, subsequently, phenotypes. Utilizing databases such as The Cancer Genome Atlas (TCGA) and Genotype-Tissue Expression (GTEx) can facilitate these integrative analyses, enriching our understanding of complex diseases.
Personalized Medicine
The trend towards personalized medicine is arguably one of the most crucial future directions for WES analysis. WES enables clinicians to tailor treatments based on an individual's genetic makeup, leading to more effective therapies. For instance, understanding a patient's unique genetic variants can inform decisions about which drugs may be most beneficial or make them aware of possible adverse reactions due to genetic predispositions.
The development of pharmacogenomics, a field focused on how genes affect a person's response to drugs, is greatly supported by WES. As more drug-gene interactions are catalogued, the role of WES will become increasingly pivotal in prescribing medications tailored to an individualโs genetic profile.
"Personalized medicine signifies a shift from a one-size-fits-all approach to a more individualized healthcare strategy, enhancing patient outcomes and ensuring effective treatment modalities."
"Personalized medicine signifies a shift from a one-size-fits-all approach to a more individualized healthcare strategy, enhancing patient outcomes and ensuring effective treatment modalities."
Epilogue
The conclusion of this article is crucial as it encapsulates the essence of Whole Exome Sequencing (WES) analysis, synthesizing the various elements discussed throughout the text. Understanding WES is increasingly important in modern genomics and medicine. It allows for the identification of genetic variants that may be responsible for various diseases, providing opportunities for early diagnosis and targeted therapies.
Summary of Key Points
- Understanding of WES: WES focuses on the exome, the protein-coding regions of the genome. This makes it a cost-effective approach compared to whole-genome sequencing, while still capturing the most clinically relevant genetic information.
- Methodologies Used: The article detailed the entire WES process, starting from sample collection to data interpretation. Knowing these methodologies is critical for researchers and clinicians aiming to utilize WES effectively in their studies or practices.
- Wide Applications: The applications of WES are numerous, ranging from genetic disease diagnosis to cancer genomics and pharmacogenomics. This versatility presents numerous opportunities for advancement in personalized medicine.
- Interpretive Challenges: It is essential to acknowledge the hurdles posed by data complexity and the ethical considerations surrounding genetic data. Understanding these challenges prepares professionals to manage them appropriately.
- Future Directions: The evolving nature of WES analysis suggests a trajectory toward integrating advanced technologies and improving clinical relevance, setting the stage for personalized medicine on a broader scope.
In summary, WES analysis is not just a technical process; it reflects a paradigm shift in understanding genetics and treating disease. The integrated approach to data processing, interpretation, and application holds immense promise for the future of genomics.
"WES is shaping the landscape of personalized medicine, translating genetic research into actionable insights for better patient outcomes."
"WES is shaping the landscape of personalized medicine, translating genetic research into actionable insights for better patient outcomes."
This brief summary can serve as a navigational tool for deeper engagement with each discussed segment, providing a framework for better understanding and application of WES in various contexts.