Fringe Projection Profilometry: Principles and Applications
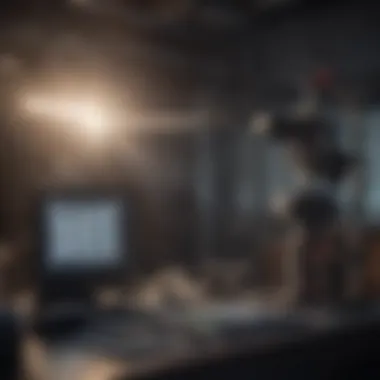
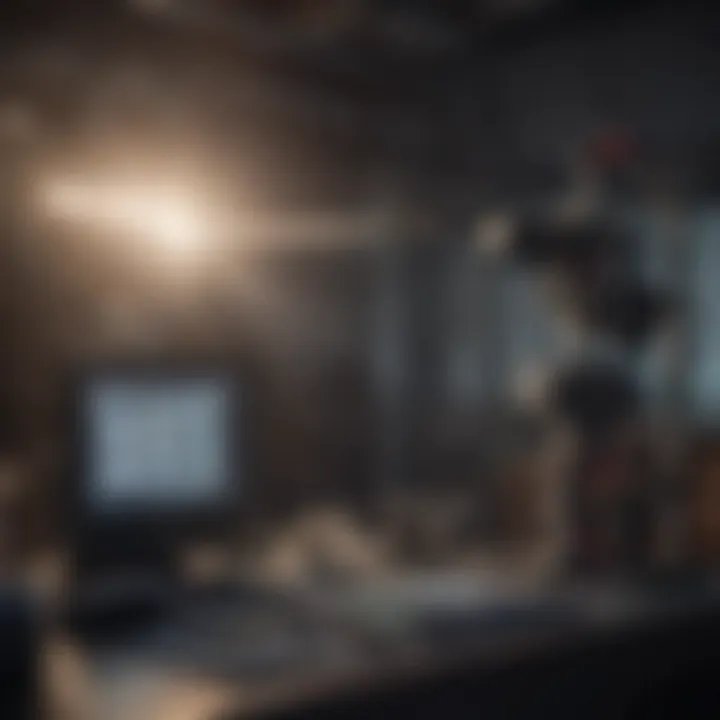
Intro
Fringe projection profilometry (FPP) stands at the intersection of optics and technology, offering a robust method for capturing three-dimensional shapes and profiles with remarkable accuracy. With the rapid advancements in measurement technologies, understanding FPP and its underlying principles becomes crucial, especially for those in fields such as manufacturing, biomedical engineering, and archaeology. This guide provides an exploration into the core concepts of FPP, alongside current trends and applications in various industries.
Key Concepts
Definition of the Main Idea
At its core, fringe projection profilometry is a non-contact optical measurement technique. It works by projecting a series of light patterns—fringes—onto the surface of an object. As these patterns deform according to the object's contours, high-resolution cameras capture the projected images. By analyzing these deformations, precise 3D profiles of the object can be constructed. Essentially, this method combines elements of optics and digital imaging to facilitate detailed measurements.
Overview of Scientific Principles
The scientific foundation of FPP rests on principles from optics, geometry, and image processing. The method typically employs a light source, often a laser or a structured light projector, to create several patterns. The algorithms involved in image processing are vital; they interpret the varying intensities and positions of the fringes after projection. This involves calculating the phase shift, which corresponds to the surface height variations across the scanned area. To put it simply:
- Fringe Patterns: Created by light interference, these are essential in determining surface shape.
- Phase Calculation: Determines the exact position of each point on the object by analyzing the deformation of fringe patterns.
- 3D Reconstruction: The final step where all data gathered is combined to form a 3D profile.
Current Research Trends
Recent Studies and Findings
Recent research in fringe projection profilometry has expanded its capabilities. Researchers are exploring enhancing the robustness of algorithms used in phase unwrapping—an essential step for reducing errors in measurement under varying light conditions or surface textures. Furthermore, combining FPP with other technologies such as computer vision and machine learning is on the rise, enabling more adaptive and intelligent measurement systems.
Significant Breakthroughs in the Field
Several notable advancements have been observed in the FPP landscape:
- Increased Speed of Capture: Newer systems can process data and create 3D models in real time, improving efficiency in industrial applications.
- Higher Precision: Advances in projector resolution and imaging sensors enable capturing finer details, essential for applications like biometrics.
- Integration with Other Technologies: The marriage of FPP with technologies such as AI and robotics is paving the way for more versatile and effective measurement solutions.
"Fringe projection profilometry symbolizes a blend of optical science and engineering that is propelling the frontiers of measurement techniques into new territories."
"Fringe projection profilometry symbolizes a blend of optical science and engineering that is propelling the frontiers of measurement techniques into new territories."
Understanding basic principles and current trends in fringe projection profilometry equips professionals with the knowledge necessary to harness this technology effectively. As it continues to evolve, staying abreast of innovations ensures that applications remain at the cutting edge, providing significant advantages in accuracy and efficiency.
Intro to Fringe Projection Profilometry
Fringe projection profilometry (FPP) is a fascinating optical measurement technique that offers a window into three-dimensional (3D) analysis, pivotal in numerous fields such as manufacturing, biomedical engineering, and cultural heritage. This article aims to illuminate the core principles and applications of FPP, highlighting its importance in contemporary optical measurement methodologies.
Understanding fringe projection profilometry opens up avenues for more precise measurements of object shapes and surfaces than traditional methods. It uses projected light patterns to create these nuanced 3D representations, fostering advancements that can lead to improved product quality in manufacturing, enhanced customization in healthcare through tailored prosthetics, and greater accuracy in archaeological documentation.
The elegance of FPP lies in its capability to provide high-resolution, non-contact profiling, minimizing the risk of damaging delicate surfaces while ensuring that artifacts or materials are accurately represented. This aspect is particularly significant when working with intricate designs or fragile components.
Defining Fringe Projection Profilometry
Fringe projection profilometry is best understood as a technique that employs projected light fringe patterns onto an object’s surface to derive its 3D structure. The projection creates a series of parallel lines or fringes on the object, which distort based on the contours of the surface. By capturing these distortions with an image acquisition system, a computational method can reconstruct the surface profile.
Key to this method is the principle of triangulation, where the position of the light source and the camera are known, allowing for computations based on the observed fringe patterns. Essentially, FPP synthesizes complex optical physics with computational analysis to achieve remarkable precision in 3D measurements.
Key Elements of FPP:
- Projected Light Patterns: The technique predominantly uses structured light from lasers or LEDs to mark surfaces with a specific pattern.
- Surface Distortion: The way fringes bend and deform upon contact with an object in focus provides necessary data for reconstruction.
- Computational Analysis: Algorithms and software handle the massive amounts of data generated, translating fringes into accurate measurements.
Historical Context of FPP Development
The roots of fringe projection profilometry reach back to advancements in optics and metrology during the mid-20th century. Initially, this technique was primarily used in scientific research, with improvements in light sources and imaging technologies paving the way for modern applications.
In the 1980s, the development of more sophisticated algorithms significantly enhanced the measurements' accuracy and speed. This period marked a transition from manual methods to more automated systems, pushing the technology further into industrial applications. As computer processing power increased, fringe projection profilometry blossomed in fields requiring high precision and efficiency.
Presently, industries harness this innovative technique, blending it with other technologies such as computer vision and machine learning to refine processes and outcomes further. Understanding this history provides context that shapes the ongoing development and application of FPP, suggesting that its potential will continue to grow in response to both technological and industrial demands.
"Knowing where we've been helps illuminate the path forward. FPP not only reflects technical evolution but also demonstrates how applied science can meet real-world challenges efficiently."
"Knowing where we've been helps illuminate the path forward. FPP not only reflects technical evolution but also demonstrates how applied science can meet real-world challenges efficiently."
By delving into the principles and applications of fringe projection profilometry, professionals across various domains can appreciate its significance in enhancing precision measurement strategies while maintaining the integrity of complex objects.
Fundamental Principles of FPP

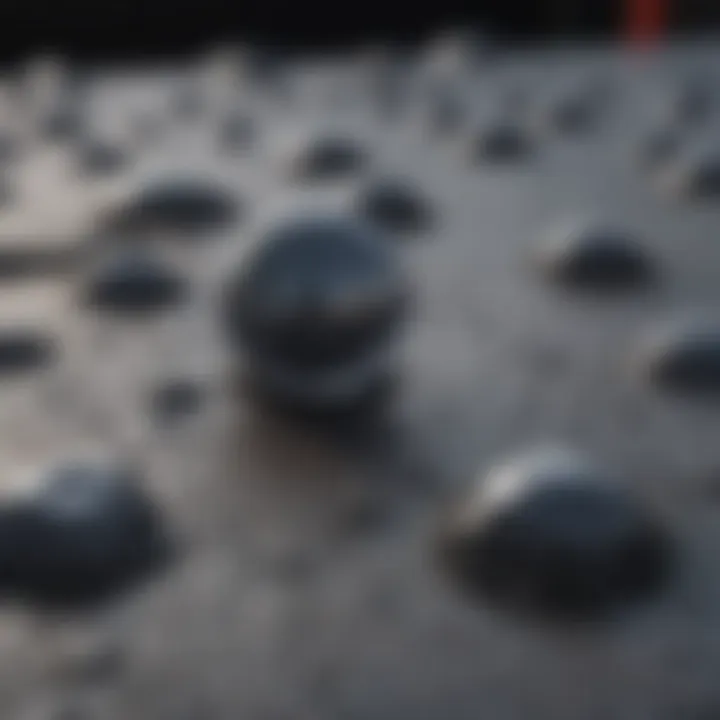
Understanding the fundamental principles of fringe projection profilometry (FPP) is crucial for anyone delving into the realm of optical measurement. These concepts form the bedrock upon which the technology is built, guiding researchers and professionals as they navigate its applications. By grasping how light interacts with objects, how images are reconstructed, and how phase data is managed, one can appreciate the depth and implications of this technique.
Light Interference and Fringe Patterns
At the heart of FPP lies the phenomenon of light interference. Simply put, when two or more light waves overlap, they can constructively or destructively interfere with each other, producing a pattern known as a fringe pattern. This is where the magic begins.
Fringe patterns can vary in intensity and color, generated by projecting a series of light bands onto an object. The manner in which these bands distort and shift can reveal a great deal about the surface structure of that object. As light waves bounce off varying heights or angles, the resulting shifts in the fringe pattern offer detailed insights into the three-dimensional geometry of the surface. For instance, if you're inspecting a car body for manufacturing defects, the fringes would help you pinpoint areas that might need adjustments—crucial in maintaining quality control.
Triangulation and 3D Surface Reconstruction
Triangulation is another pivotal component of FPP. It operates on a straightforward premise: by measuring the angles and distances involved when light hits the object and is captured by the camera, it’s possible to reconstruct the object's 3D shape accurately.
In this process, a known distance is established between the projector and camera while a fringe pattern is projected onto the object. The camera captures the resulting changes in the fringe pattern, and from this data, the disparities in the surface's height can be calculated. The combination of these measurements creates a detailed 3D map of the object’s surface. This is particularly useful in industries like aerospace, where precision is paramount. The ability to reconstruct complex geometries enables engineers to design components that fit together perfectly, minimizing errors and streamlining production.
Phase Unwrapping Techniques
Phase information is inherently wrapped within the interference patterns captured by the system. Phase wrapping occurs when the inherent values exceed 2π (approximately 6.28). As a result, the measurements can appear distorted or misleading if not correctly unwrapped. This is where phase unwrapping techniques become critical.
Various methods exist to unwrap phase data, enabling the accurate retrieval of 3D surface information. Techniques such as the branch-cut method or minimum-norm approach help navigate through this complexity. Failure to apply appropriate unwrapping can lead to severe inaccuracies, potentially resulting in flawed products or research outcomes. It’s important to select the right technique based on factors like noise levels and surface texture to ensure the highest quality reconstruction.
"To get accuracy in reverse engineering or quality inspection, understanding phase unwrapping is not just crucial; it is essential."
"To get accuracy in reverse engineering or quality inspection, understanding phase unwrapping is not just crucial; it is essential."
In summary, the principles of light interference, triangulation, and phase unwrapping are integral to the function of FPP. Mastery of these concepts enhances not just the practical applications but also deepens the understanding of the technology’s potential in creative ways to solve real-world challenges.
Components of a Fringe Projection Profilometer
In the realm of fringe projection profilometry, understanding the components of a fringe projection profilometer is pivotal. These components work in unison to capture and analyze the intricate details of three-dimensional surfaces. Each element holds significance, impacting the overall accuracy, efficiency, and applicability of the profilometr.
Light Source Selection
Choosing an appropriate light source is crucial in fringe projection profilometry. This decision affects the quality of the fringes produced, which in turn influences the measurement precision. Several types of light sources can be used:
- Laser Sources: Specially designed gas lasers emit coherent light, yielding sharp fringe patterns. This coherence is vital for high-resolution measurements but can be costly and complicated.
- LEDs: LEDs are gaining traction due to their affordability and versatility. They can produce various wavelengths, allowing flexibility in capturing different surface types.
- Projectors: High-definition projectors offer advantages in certain applications, especially where larger surfaces are considered. However, managing light divergence poses some challenges.
The right light source, aligned with the surface characteristics of the object being measured, can significantly enhance the output of the profilometer.
Optical Elements and their Functions
The optical components play numerous roles within a profilometer, starting from the generation of fringe patterns to image capture. Among the key optical elements are:
- Lenses: They focus and shape the light beams, influencing the visibility and clarity of the fringe patterns. Selecting appropriate focal lengths can mitigate distortions when capturing data across various distances.
- Beam Splitters: These elements are essential for dividing the light beam into multiple paths, allowing simultaneous projection onto the object and the reference surface. This setup is paramount for obtaining accurate phase measurements.
- Filters: Optical filters can enhance contrast by eliminating unwanted wavelengths. Utilizing band-pass filters, for instance, can improve the signal-to-noise ratio in the captured images.
Careful consideration in the arrangement and quality of optical elements can drastically affect measurement effectiveness, making their selection a strategic challenge in system design.
Image Acquisition Systems
The image acquisition system is another linchpin in fringe projection profilometry, responsible for transforming the optical information into digital data. This system's architecture can greatly impact data fidelity. Key components include:
- Cameras: Modern high-resolution cameras with rapid frame rates drastically improve image capture speed and quality. A camera's sensitivity and pixel density are paramount; they must align with the projected fringe's frequency and spatial resolution to ensure minimal data loss.
- Synchronization Circuitry: It coordinates the timing between the projector and the camera, ensuring that measurements align precisely. Any discrepancy here can result in blurred data and unreliable surface reconstructions.
- Data Processing Units: These units interpret the captured images, applying algorithms for processing the fringes into 3D representations. The choice of processing software can influence both the speed and accuracy of the resulting data.
Effectively integrating these image acquisition components is critical to obtaining valid measurements and can directly affect the outcome of the profiling process.
"In profiling, a well-aligned system of components can make the difference between accurate measurement and wasted effort."
"In profiling, a well-aligned system of components can make the difference between accurate measurement and wasted effort."
Understanding and selecting the right components is essential not only for precision measurement but also for streamlining overall workflow in various applications. Whether it’s in manufacturing settings or detailed biomedical analysis, the components work together like cogs in a machine, each contributing to the final product's reliability and accuracy.
Applications of Fringe Projection Profilometry
Fringe projection profilometry (FPP) has transcended mere academic curiosity to become a cornerstone in various fields, each drawn in by its ability to produce precise three-dimensional measurements. These applications not only reflect the versatility of FPP but also its significance in the realm of engineering, healthcare, and heritage conservation. Understanding the different sectors where this technology shines can shed light on its impact and relevance in today’s complex workflows.
Use in Manufacturing Processes
Quality Assurance and Control
In the world of manufacturing, quality assurance and control are paramount. Every component that rolls off the production line must adhere to strict specifications. FPP is invaluable in this aspect. With its non-contact nature and high accuracy, it enables manufacturers to monitor product quality in real time.
One key characteristic of using FPP in this sphere is its ability to capture detailed surface features quickly. Time is often of the essence in production environments, and utilizing this technology allows for expedited testing of parts without compromising accuracy.
Furthermore, the significant advantage it offers is its flexibility in adapting to different product shapes. Whether it’s automotive parts or delicate electronics, FPP handles a range of geometries with impressive precision. However, it does come with some downsides. For instance, parts must often be clean and free of reflective coatings as these can distort measurements, necessitating additional preparation steps.
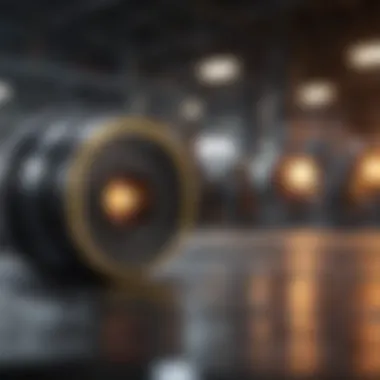
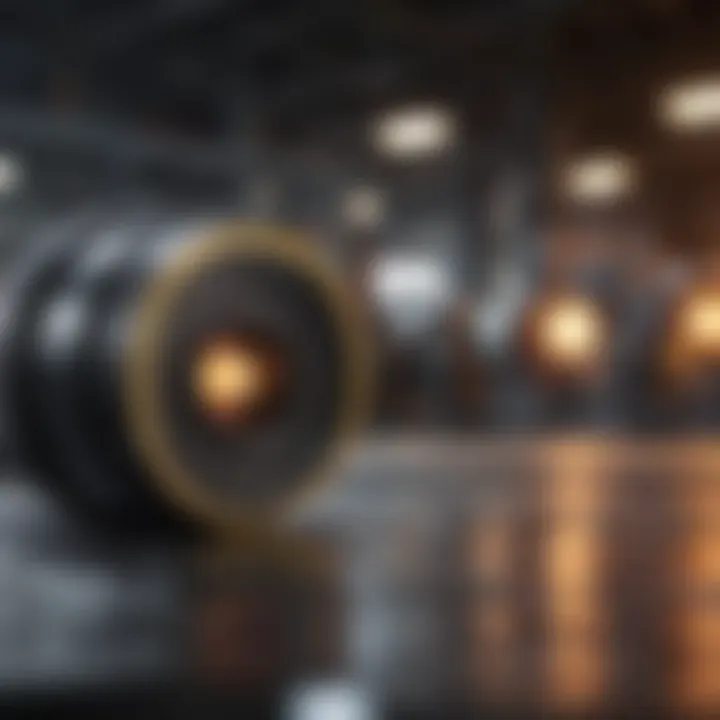
Reverse Engineering
Reverse engineering relies heavily on precise measurements to recreate or enhance existing objects, and FPP’s strengths can be a game changer here. At its core, reverse engineering involves deconstructing a product to understand its design or to improve it. The technology’s rapid 3D scanning capabilities enable engineers to acquire complex geometries quickly. What makes FPP particularly useful in reverse engineering is its ability to capture intricate details that traditional methods might miss. This capability helps in not just replicating the item accurately, but also in assessing areas where improvement can be made in design or function. Yet, like everything, there's a flip side. Occasionally, FPP can struggle with highly reflective surfaces, requiring additional modifications to the object or the process. This can lead to increased time and resource expenditure, which might not be ideal for every project.
Biomedical Engineering Applications
Prosthetics and Custom Implants
In biomedical engineering, FPP has carved a niche for itself, particularly in the design of prosthetics and custom implants. Its precision enables medical professionals to create devices tailored to individual anatomical requirements. This personalization highlights one significant characteristic: the ability to achieve anatomical fidelity, which is crucial for both comfort and functionality. The unique feature of applying FPP in this context lies in the seamless integration of 3D modeling with patient data. By utilizing this technology, practitioners can swiftly and accurately convert patient scans into manufacturable designs, paving the way for bespoke solutions. On the downside, the initial costs of integrating such advanced technology can be high, which may deter smaller institutions from adopting it.
Biometrics
Biometrics represents another critical area where FPP excels. It’s about measuring and assessing human physical traits, and precise measurement is non-negotiable. FPP stands out in this domain because of its non-invasive character—no need to touch the subject means greater comfort and lower risk of error.
Moreover, one of its distinctive benefits is the speed at which it can gather and process data. Unlike traditional biometric methods that rely on physical contact or slower scanning techniques, FPP can quickly capture detailed metrics of a person’s features for security, identification, or even healthcare purposes. However, the limitation remains that intricate facial features can sometimes result in variations based on lighting conditions and surface textures, leading to potential inaccuracies.
Cultural Heritage and Archaeology
Preservation of Artifacts
In cultural heritage and archaeology, preserving artifacts is not just about safe storage. It involves accurate documentation and analysis to prevent loss due to time or environmental factors. FPP shines bright here due to its ability to create 3D models without direct contact. This non-intrusive method ensures that the integrity of fragile artifacts is maintained, making it a popular choice among conservators and archaeologists alike. The unique advantage of utilizing FPP in preserving artifacts is the detailed surface mapping it provides, which can aid in restoration efforts. Yet again, the drawback includes the technology's dependence on ideal lighting and surface conditions. Dim lighting or highly textured surfaces can pose challenges, occasionally necessitating additional adjustments to the workspace.
Site Documentation
Site documentation is crucial in archaeology for understanding historical contexts and planning future excavations. By employing FPP, archaeologists gain the advantage of detailed site mapping with ease. One key characteristic of this method is its ability to cover large areas quickly, resulting in more comprehensive data collection. A unique feature in this application is the capability to integrate various datasets, such as photos and scans into one coherent model. Though this brings efficiency, it also presents challenges. The initial setup can sometimes be cumbersome, requiring meticulous planning and coordination among teams, which may slow down the early stages of a project.
By examining these diverse applications, it becomes evident that FPP has solidified its place across multiple fields, showcasing both its versatility and its significant contributions. As technology continues to advance, its importance is likely to grow, promising exciting developments ahead.
Technological Advances in FPP
In recent years, the field of fringe projection profilometry (FPP) has seen significant technological strides that have enhanced its capabilities and applications. These advances not only improve accuracy and efficiency but also expand the potential uses across various industries. Understanding these developments is crucial for those involved in engineering, manufacturing, and research, as they can lead to better practices and innovative solutions.
Integration with 3D Imaging Technologies
The integration of fringe projection profilometry with 3D imaging technologies marks a pivotal leap forward. While FPP effectively captures surface profiles, coupling it with advanced 3D imaging methods allows for more comprehensive data analysis. This combination enables professionals to visualize physical objects in a more holistic manner, turning raw point data into detailed 3D models that can be further processed or analyzed using sophisticated software.
- Enhanced Data Quality: By utilizing complementary imaging technologies, the fidelity of the captured data improves significantly. This leads to more reliable measurements and reduces uncertainties associated with surface irregularities.
- Real-time Applications: Modern integration allows for real-time scanning and processing, greatly benefiting areas like quality control in manufacturing. For example, in automotive production, immediate feedback on part dimensions can facilitate timely adjustments, preventing costly corrections later.
- Interoperability: As systems become more interconnected, the sharing of data across platforms becomes seamless. This means information gathered from FPP systems can easily be interpreted alongside data from other 3D technologies, creating a rich informational tapestry for any given project.
Automated Processing Algorithms
Another notable advancement in fringe projection profilometry is the development of automated processing algorithms. These algorithms play a critical role in streamlining the workflow from data acquisition to analysis.
- Improved Efficiency: Automating the analysis process minimizes the time spent on manual calculations and adjustments. This efficiency is particularly noticeable in high-volume environments, where countless components need evaluation without sacrificing quality.
- Accurate 3D Reconstruction: Advanced algorithms utilize sophisticated mathematical models that refine the reconstruction of 3D surfaces. They can effectively handle noise and inaccuracies that often challenge traditional methods, resulting in cleaner, more precise outputs.
- Scalability: The automation of these processes increases scalability. Industries can effortlessly adapt to growing demands without needing to significantly increase labor costs. As businesses expand, automated systems can manage higher workloads with less intervention.
To summarize, the technological advances in fringe projection profilometry are not merely enhancements—they fundamentally alter the trajectory of how professionals approach measurement and analysis. The integration with 3D imaging technologies and the rise of automated processing algorithms represent just a glimpse into a rapidly evolving landscape where precision and efficiency reign supreme.
"The real revolution in measurement techniques springs from technological integration and automation, driving us towards unprecedented precision in industrial applications."
"The real revolution in measurement techniques springs from technological integration and automation, driving us towards unprecedented precision in industrial applications."
By embracing these changes, organizations not only keep up with industry standards but also set themselves apart by leveraging cutting-edge technology to fulfill their objectives.
Advantages of Fringe Projection Profilometry
Fringe Projection Profilometry (FPP) brings several advantages that position it as a pivotal technology in various fields. By harnessing optical methods to perform accurate 3D measurements, FPP stands out for its precision and adaptability. Understanding these benefits can help industries optimize their processes while ensuring quality and reliability. This section elaborates on two key advantages of FPP, shedding light on why it remains a valuable tool in the realm of measurement techniques.
Non-Destructive Testing
One of the hallmark advantages of FPP is its capability for non-destructive testing. In environments where maintaining the integrity of a material or product is vital, traditional measurement techniques often cause damage or exert inappropriate forces. FPP, however, utilizes light patterns and projections to ascertain profiles without touching the item itself. This characteristic is crucial in sectors like aerospace and automotive manufacturing, where the cost of even a minor defect can be astronomical.
For instance, consider the inspection of turbine blades. A single flaw can compromise functionality and safety. FPP allows engineers to measure micro-geometries and assess surface roughness without risking any harm to the delicate structures. Essentially, this means that components and systems can be evaluated thoroughly while preserving their usability and lifespan.
"Non-destructive methods in testing materials not only save resources but also extend the lifecycle of the tested components, leading to significant economic efficiency."
"Non-destructive methods in testing materials not only save resources but also extend the lifecycle of the tested components, leading to significant economic efficiency."
In addition to safety and durability, non-destructive testing using FPP translates into better data collection during the production phase. This leads to more effective quality control measures, ensuring that products meet specified standards before they reach the end users.
High Resolution and Accuracy
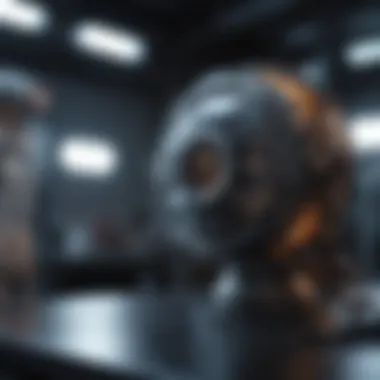
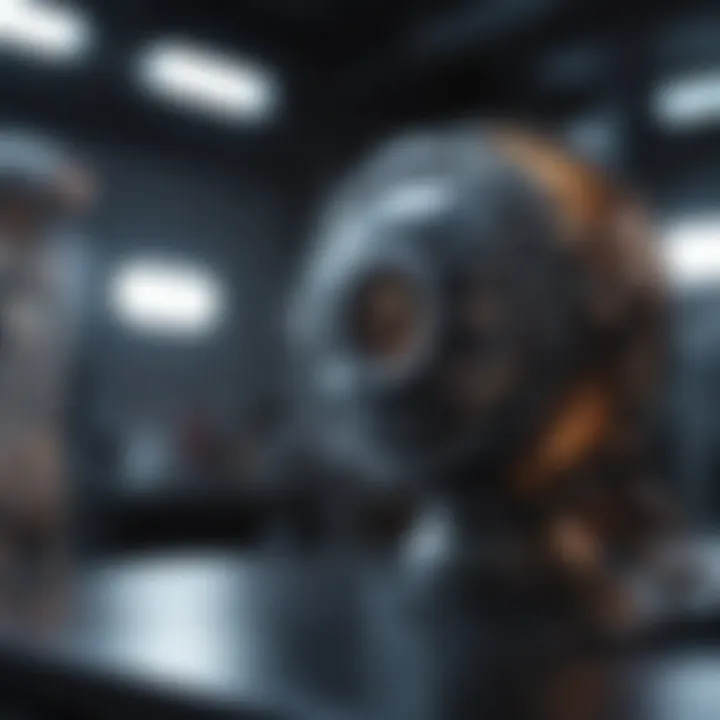
When discussing measurements, resolution and accuracy are two terms that often get thrown around. But in FPP, these concepts take on a practical significance that influences operational outcomes significantly. This technology achieves remarkable levels of detail, exceeding the capabilities of many traditional contact-based methods.
Modern fringe projection systems can capture surface details down to the micron level, making them indispensable in fields requiring extraordinary precision. For example, in the fabrication of intricate medical devices, the slightest deviation in measurements can severely impact performance and patient safety. Thus, FPP's high resolution ensures that crucial components are manufactured within tolerable limits.
Another compelling point about accuracy in FPP is its reduced susceptibility to errors. Traditional techniques often rely on human interpretation, which can introduce subjective biases and discrepancies. In contrast, FPP automates the process by employing sophisticated algorithms that analyze the light patterns created by projections on surfaces. This objective approach minimizes room for error, providing outcomes that are both repeatable and verifiable.
The combined benefits of non-destructive testing and high-resolution accuracy position Fringe Projection Profilometry as a frontrunner in measurement technologies. These factors contribute to higher efficiency in workflows while minimizing waste and ensuring consistent quality in product outputs.
Challenges in Fringe Projection Profilometry
Fringe projection profilometry, while an advanced and efficient method for capturing 3D shapes, is not without its set of challenges. Understanding these hurdles is crucial for professionals and researchers engaged in this field. The intricacies of measuring surface profiles mean that various factors can interfere with the accuracy and reliability of results. By examining the limitations posed by surface properties and environmental influences, it is possible to refine techniques and enhance outcomes.
Limitations in Surface Properties
One of the foremost challenges in fringe projection profilometry comes from the nature of the surfaces being measured. Not all materials are conducive to clear and distinct fringe patterns. For instance, surfaces that are too shiny or have irregular textures can lead to scattered light, making it difficult to extract accurate phase information. Here are some common limitations associated:
- Reflective Surfaces: Highly reflective materials can distort fringe patterns. The light reflects unpredictably, muddying the data.
- Transparent Materials: Items made from glass or plastics can complicate measurements, as they allow light to pass through instead of reflecting it back uniformly.
- Texture Variability: Materials with varying surface textures can create issues with coherence and interferometric patterns. Uneven surfaces may result in incomplete data capture.
Efforts can be made to overcome these limitations, for instance, by using special coatings that reduce reflectivity or by employing preprocessing techniques that enhance fringe visibility. However, it presents a continual challenge that researchers need to navigate.
Environmental Influences
The outside world can dramatically affect the performance of fringe projection profilometry systems. When measurement conditions fluctuate, it can lead to significant variances in accuracy. Consider these factors:
- Ambient Light: Excessive external lighting can interfere with the projection and detection of fringes. It’s essential to control the lighting environment or utilize adaptive filtering techniques.
- Vibrations: Any mechanical vibrations in the vicinity can disrupt the precise alignment of the optical components, leading to false readings. Use of vibration-isolation systems is often necessary in sensitive measurements.
- Temperature Variations: Swings in temperature might cause expansion or contraction of materials, which can alter the positioning or behavior of both the object and the equipment. Maintaining a stable environment is key.
Understanding and addressing these environmental influences is critical for ensuring reliable and valid measurements in fringe projection profilometry.
"Measurement accuracy often hinges on the meticulous management of environmental variables along with the surface characteristics of objects."
"Measurement accuracy often hinges on the meticulous management of environmental variables along with the surface characteristics of objects."
Future Prospects of FPP Development
As the field of optical measurement continues to evolve, fringe projection profilometry (FPP) is positioned at the forefront of innovation. The ongoing advancement in technology, such as improvements in light sources and image processing algorithms, suggests promising directions for FPP that may redefine its capabilities and applications. Understanding these upcoming possibilities is essential for researchers, students, and professionals who aim to leverage this technology effectively.
Emerging Trends and Innovations
In recent years, several emerging trends in fringe projection profilometry have begun to take shape, reflecting its adaptability in various fields. Here are some notable developments:
- Increased Integration with Machine Learning: Algorithms powered by machine learning are now being used to enhance the analysis of fringe patterns. By automating phase unwrapping and surface reconstruction, these advancements can make processes more efficient and less error-prone.
- Use of Advanced Light Sources: The advent of new light sources like laser diodes and high-resolution projectors significantly improves measurement speed and accuracy. These advancements help FPP deliver higher-resolution profiles while minimizing noise and artifacts.
- Portable and Miniaturized Systems: The trend towards compact and portable profiling systems means that FPP can now be deployed in more varied environments. This opens doors for in-field applications, especially in sectors like construction and quality assurance.
- Real-Time Data Processing: Developments in real-time image processing techniques allow for immediate feedback in measurement tasks. This feature is especially beneficial during manufacturing where timely decisions can enhance productivity and reduce errors.
These innovations signal that FPP is not just an established tool but one that is living and adapting to the needs of various industries.
Potential New Applications
The prospects for fringe projection profilometry aren't only limited to existing applications; they also stretch into unexplored territories that could yield significant benefits. Some potential new applications include:
- In Aerospace Engineering: The precision required in aerospace components makes FPP a candidate for inspecting complex geometries and surface features in turbine blades and wing structures.
- Digital Twin Technology: As industries move toward digitalization, FPP could play a crucial role in creating digital twins of physical systems. This integration would facilitate better monitoring and predictive maintenance of equipment.
- Cultural Heritage Documentation: Beyond the current uses in archaeology, FPP can assist in detailed documentation of cultural artifacts, enabling non-invasive scanning that preserves their integrity.
- Advanced Robotics: In robotics, especially where tactile sensors are fundamental, FPP could assist in enhancing perception for robots, improving their interaction with complex environments.
"Innovation is the heartbeat of progress; as FPP intertwines with emerging technologies, it paves the way for a future with broader horizons."
"Innovation is the heartbeat of progress; as FPP intertwines with emerging technologies, it paves the way for a future with broader horizons."
The adaptability and potential of fringe projection profilometry are evident, and its future appears bright. The intersection of technology and application will likely steer further research and investment, magnifying the impact FPP can have across numerous disciplines.
Ending
In wrapping up our exploration into fringe projection profilometry (FPP), it becomes clear that this technique holds immense value across various sectors. The conclusion here isn't just a summary of facts but an affirmation of FPP's significance in modern measurement practices. By adeptly capturing three-dimensional profiles with a level of precision that other traditional methods sometimes fail to achieve, FPP stands out as a pivotal tool for industries ranging from manufacturing to biomedical applications.
Summary of Key Insights
Fringe projection profilometry operates on the principles of light interference and triangulation, effectively transforming complex shapes into accurate digital representations. Key elements include:
- High-speed data capture: Capable of producing detailed measurements quickly, making it ideal for time-sensitive projects.
- Non-destructive testing: Ensures that the integrity of the object remains intact while analyzing its measurements.
- Adaptability across fields: Whether it's for developing custom prosthetics or meticulously documenting artifacts for cultural heritage, FPP proves its versatility.
Moreover, advancements in technology have ushered in new techniques, enhancing efficiency and accuracy in object scanning and surface analysis. With each stride, the insights gleaned from utilizing FPP pave the way for innovations that can redefine expectations in various domains.
Final Thoughts on FPP's Relevance
Looking into the future, the relevance of fringe projection profilometry is undeniably set to grow. As industries increasingly seek high accuracy and speed in measuring techniques, FPP is positioned to respond to these demands. The potential for new applications is expansive; from improving quality control in manufacturing to enhancing outcomes in personalized medicine, its impact could be substantial. This progress hinges on a deeper understanding of FPP's fundamental principles and overcoming current challenges, such as environmental influences on measurement fidelity.
In essence, FPP is not just a trend; it is a foundational element of optical measurement that continues to evolve and adapt, reinforcing its place in both academic and practical spheres. The ongoing research and application of FPP will likely lead to further innovations and greater adoption across diverse fields in the years to come.
In essence, FPP is not just a trend; it is a foundational element of optical measurement that continues to evolve and adapt, reinforcing its place in both academic and practical spheres. The ongoing research and application of FPP will likely lead to further innovations and greater adoption across diverse fields in the years to come.