GFP Immunostaining: Techniques and Applications
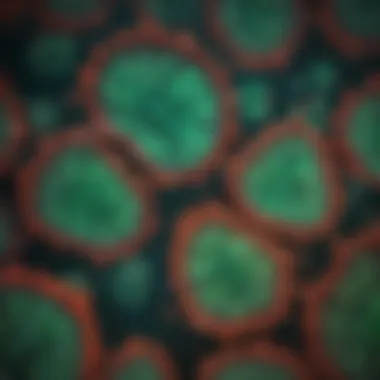
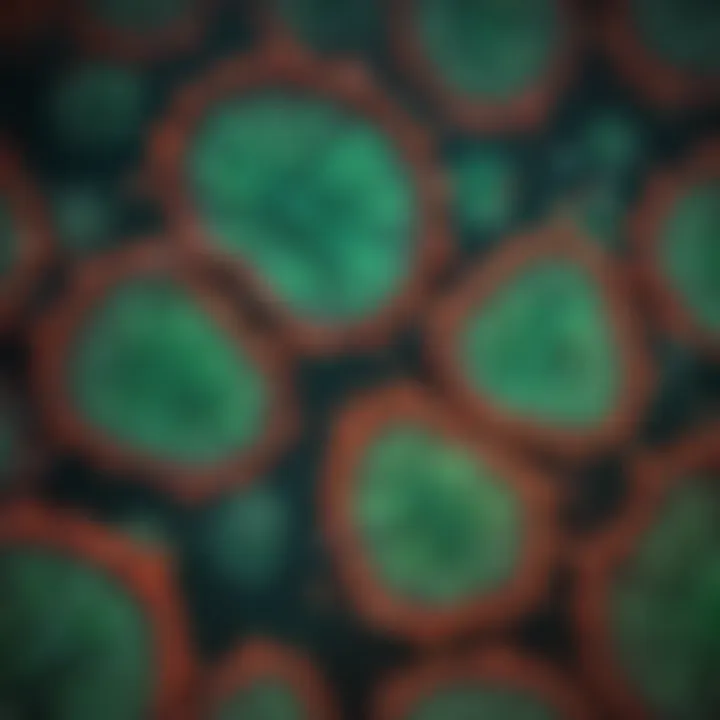
Intro
Green Fluorescent Protein (GFP) immunostaining has dramatically transformed how scientists visualize cellular structures and processes. This method leverages the intrinsic fluorescent properties of GFP, allowing for real-time observation of proteins, cells, and tissues under a microscope. What once required tedious labeling techniques now affords researchers unprecedented ease and reliability in tracking biological processes, making it a cornerstone technique in molecular biology.
The principle behind GFP immunostaining rests on the utilization of fluorescent proteins, a class of proteins that emit bright green light when exposed to specific wavelengths of light. Initially derived from the jellyfish Aequorea victoria, GFP has become a fundamental tool in a variety of experimental setups. From studying cellular localization to conducting gene expression analysis, GFP's applications are boundless, illuminating pathways in both research and clinical endeavors.
In this article, we aim to elaborate on pivotal concepts, current trends, and future directions in the realm of GFP immunostaining. Understanding both the science and techniques behind this powerful tool is essential for anyone venturing into advanced biological research.
Prelims to GFP Immunostaining
Green fluorescent protein (GFP) immunostaining involves the use of GFP to visualize specific proteins in cells and tissues. This technique is crucial for understanding cellular function, mapping protein interactions, and tracking gene expression in various biological systems. By making biological structures visible through fluorescence, researchers can gain insights into complex cellular processes that were previously obscured.
There are several reasons why GFP immunostaining holds a prominent place in the toolkit of modern biology:
- Versatility: GFP can be tagged to a wide variety of proteins, allowing for detailed studies across different organisms and cell types.
- Non-invasive Imaging: Once expressed, GFP allows for real-time imaging in living organisms, minimizing the need for destructive methods that may alter the natural state of the sample.
- High Sensitivity: The fluorescent nature of GFP provides a strong signal that can be detected even in low-abundance proteins compared to traditional staining techniques.
In addition to these benefits, there are also considerations to address when utilizing GFP immunostaining. Sample preparation methods must ensure optimal expression and localization of GFP. Potential pitfalls include issues stemming from the stability of the fluorescent signal, the specificity of the antibodies used, and the reliability of imaging setups. Thus, careful planning is essential to maximize the effectiveness of this powerful technique.
Historical Context of GFP Discovery
The story of GFP begins with the discovery of the jellyfish Aequorea victoria in the 1960s. Researchers found that this organism glows green when exposed to ultraviolet light. Later, it was identified that the glowing property was due to a protein present in the jellyfish, which was later named Green Fluorescent Protein. This groundbreaking revelation paved the way for the genetic manipulation of organisms to express GFP, leading to its widespread use in biological research.
In the late 1990s, the application of GFP took a revolutionary turn. Scientists such as Roger Tsien and Osamu Shimomura were key players in the development and optimization of GFP variants, expanding its utility in diverse fields of study. Their work eventually earned them the Nobel Prize in Chemistry in 2008. The discovery has not only enhanced our understanding of biological systems but has also set the scene for the development of novel imaging techniques.
Significance of Immunostaining in Biology
Immunostaining plays an integral role in biological research, serving as a bridge between theory and practice. It allows scientists to visualize and accurately identify proteins, cellular structures, and networks of interaction within tissues and cells. The correlation between the fluorescence signals and the biological phenomena under study adds depth to quantitative analyses.
One of the more profound implications of immunostaining can be seen in developmental biology. By labeling and tracking cells as they divide and differentiate, researchers can unravel the mysteries of embryonic development and organ formation. This can lead to advances in regenerative medicine and tissue engineering.
Moreover, immunostaining is instrumental in clinical diagnostics as well, allowing for the identification of specific biomarkers associated with diseases, including cancers. Understanding the localization and abundance of these proteins can provide valuable insights into disease mechanisms and inform treatment strategies.
"Immunostaining has transformed how we observe and interpret the intricate dance of biological molecules in live cells and tissues, revealing a wealth of information previously hidden from view."
"Immunostaining has transformed how we observe and interpret the intricate dance of biological molecules in live cells and tissues, revealing a wealth of information previously hidden from view."
In summary, the introduction of GFP immunostaining into the scientific community represents not just a technical advancement but also a paradigm shift in how biological research is conducted. The evolution of techniques surrounding GFP has empowered scientists to explore new frontiers in biology, promising insights that could lead us to significant breakthroughs.
Fundamentals of Green Fluorescent Protein
Green Fluorescent Protein (GFP) has become a cornerstone in molecular biology, offering powerful insights into cellular processes. Its fundamental aspects are critical for grasping its implications in research and clinical applications. Understanding GFP goes beyond just its usage; it encompasses the molecular architecture and biochemistry that make it a reliable tool in visualizing biological phenomena.
Structure and Function of GFP
GFP, originally sourced from the jellyfish Aequorea victoria, boasts a unique three-dimensional structure notable for its chromophore, which is responsible for its green fluorescence. The backbone is primarily composed of a beta-barrel structure, with the chromophore situated within its interior. This configuration provides stability and protection for the chromophore, allowing it to emit light when excited by specific wavelengths. It’s fascinating that the GFP’s natural fluorescence can be attributed to a single amino acid modification, which gets formed through an autocatalytic process. Such simplicity amidst complexity is a hallmark of biological systems.
Importance of GFP’s Structure
The rigidity of its structure not only ensures consistent fluorescence but also minimizes photobleaching, making it suitable for long-term imaging. This characteristic is especially crucial in live cell studies where researchers aim to monitor dynamic biological processes in real-time. For instance, in developmental biology, GFP can be used to trace cell lineages, providing significant insights into cellular differentiation and organ development.
Mechanisms of Fluorescence
Understanding how GFP emits fluorescence is essential for harnessing its potential in biological research. The fluorescence of GFP arises when the chromophore absorbs light, resulting in an excited state. This excited state quickly returns to a ground state, releasing energy as light. It’s an elegant dance of electrons, where the energy transfer is efficient and robust.
- Excitation: Exposure to a light source with the right energy prompts the electrons of the chromophore to jump to a higher energy state.
- Emission: As these excited electrons return to their original state, excess energy is released as light.
The specific wavelengths of light involved define the operational parameters of GFP in practical experiments. Typically, GFP is excited at around 395 nm, with an emission peak at approximately 509 nm. This makes GFP particularly useful in fluorescence microscopy, as its emission spectrum overlaps nicely with common fluorescence filters.
GFP's mechanisms can be influenced by external factors like pH and temperature, serving as a vital consideration while designing experiments. In practical terms, researchers must often calibrate their settings, ensuring that they squeeze every bit of valuable information from their observations.
"GFP is not just a tool; it is a window into cellular activity, illuminating secrets that were once hidden from scientific inquiry."
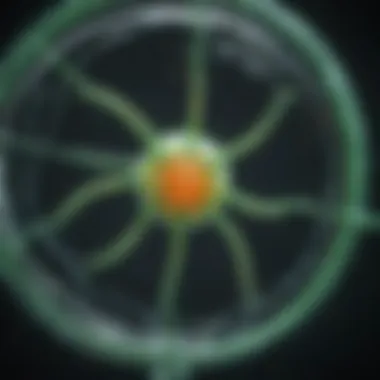
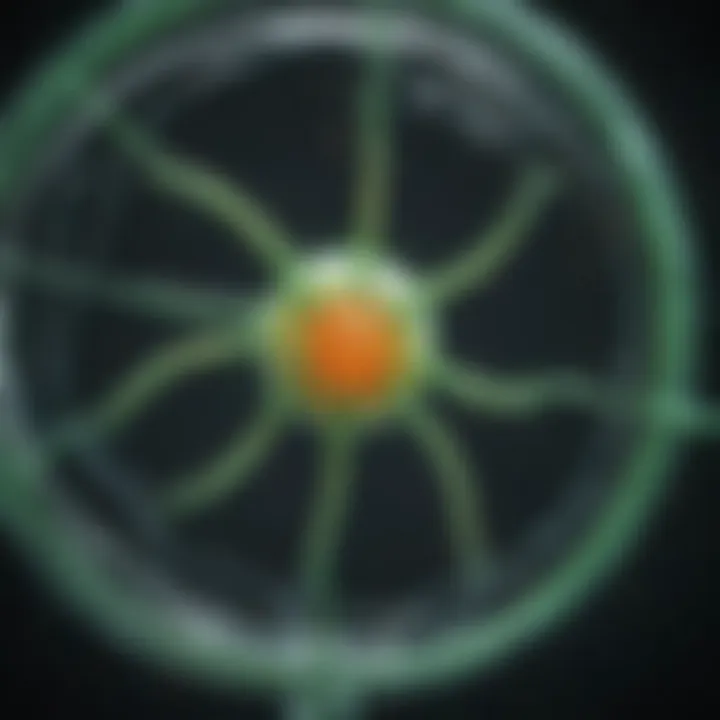
"GFP is not just a tool; it is a window into cellular activity, illuminating secrets that were once hidden from scientific inquiry."
Beyond the intrinsic qualities of GFP, advances in technology continue to enhance its application. Novel derivatives of GFP, engineered for various purposes, exemplify how this foundational knowledge can lead to innovative breakthroughs, such as multi-color labeling in complex tissue studies. Such developments assure that the realm of GFP immunostaining is vibrant, with potentials waiting to be uncovered.
The Immunostaining Process
The immunostaining process holds a critical position in the domain of GFP analysis. Without this important step, the nuances of cellular and molecular biology might remain obscured. Immunostaining essentially offers a refined method to visualize and identify specific proteins within complex biological samples. Failing to grasp the intricacies of this process could lead to misinterpretations in research outcomes, which can ripple through entire studies.
Preparation of Samples for Staining
The preparation of samples is foundational for effective immunostaining. It’s like laying a solid groundwork before building a house; if the base isn’t strong, the structure won’t hold. Typically, biological samples, whether they come from cultured cells, tissues, or organisms, must be properly handled to preserve their architecture and spatial relationships. This is where fixation processes come into play.
Common fixation methods include formaldehyde and methanol, each impacting the subsequent staining outcomes differently. For instance, formaldehyde, while preserving the morphology, may mask certain antigenic sites, necessitating additional steps to unmask these hidden proteins. Hence, the choice of fixation warrants careful consideration to ensure that the antigens of interest remain accessible for antibody binding.
Moreover, after fixation, samples often undergo a series of washing steps. These procedures, generally with phosphate-buffered saline (PBS), remove excess fixative and prepare the samples for antibody application.
Primary and Secondary Antibodies
Once the samples are prepped, the next pivotal phase involves the application of antibodies. Antibodies serve as critical tools in immunostaining, more so the primary antibodies, which directly bind to the target antigen. Choosing the right primary antibody is paramount. Specificity is crucial; a well-suited antibody will adhere to its target like a glove fitting a hand.
After the primary antibody has been incubated with the sample, a secondary antibody is then utilized. This secondary antibody typically binds to the primary antibody and is usually conjugated with a fluorophore, such as a variety of variants of GFP or other fluorescent dyes.
An effective strategy is to select a secondary antibody that corresponds to the host species of the primary antibody. For instance, if the primary was derived from a rabbit, an anti-rabbit secondary antibody would be appropriate. This amplification step increases the sensitivity of detection and ensures that even low levels of the target antigen can be visualized.
Detection Systems and Fluorescence Microscopy
Finally, the detection systems and fluorescence microscopy techniques forge the pathway for visualization post-staining. The aim here is to translate the fluorescent signals emitted by the attached fluorophores into viewable images. Multiphoton excitations, for example, have opened new frontiers in imaging depth beyond the limitations of traditional microscopy.
Fluorescence microscopy has evolved significantly, allowing for advanced imaging techniques like live-cell imaging and multicolor staining. For the observer, understanding these systems is key, as they dictate the clarity and accuracy of the displayed images.
It's important to note that the quality of the detection system directly affects the resolution of the fluorescence images, underlining the necessity for top-notch equipment and techniques.
It's important to note that the quality of the detection system directly affects the resolution of the fluorescence images, underlining the necessity for top-notch equipment and techniques.
Using appropriate settings, such as correct excitation wavelengths and emission filters, can drastically alter the visibility of your target structures. Poor settings may swamp the image in background noise or blurry details, which can lead to erroneous conclusions in research.
In summary, the immunostaining process, comprising preparation, antibody application, and advanced detection mechanisms, serves as the backbone for successful GFP studies. A meticulous approach to each phase can yield enlightening insights into cellular functions and behaviors, enriching the tapestry of molecular biology.
Techniques in GFP Immunostaining
In the realm of cellular biology, understanding the nuances of GFP immunostaining is paramount. With a myriad of techniques available, the journey starts with the fundamental choice between direct and indirect methods. Choosing the right technique can greatly influence the clarity and accuracy of findings. It’s essential to navigate these methods, as each offers unique benefits and considerations that warrant a close look.
Direct vs. Indirect Immunostaining Methods
Direct immunostaining employs a single antibody that is conjugated to a fluorophore, allowing for immediate visualization of the target. This method is appreciated for its simplicity and speed. Researchers may find this approach particularly useful in scenarios where time is of the essence — fast-paced experimental situations where results are needed quickly. However, the downside is often a lower signal intensity, which can obscure subtle interactions.
On the flip side, indirect immunostaining involves using two antibodies: a primary antibody that targets the antigen of interest, followed by a secondary antibody that is conjugated to a fluorophore. This increases the signal due to the amplification from multiple secondary antibodies binding to a single primary antibody. While this method might take longer due to additional steps, its sensitivity can uncover minute details often missed in direct methods.
"Choosing between direct and indirect methods can make or break the clarity of your findings. It's like opting for a single spotlight versus a flood of lights to illuminate a scene—each has its place depending on what you need to see."
"Choosing between direct and indirect methods can make or break the clarity of your findings. It's like opting for a single spotlight versus a flood of lights to illuminate a scene—each has its place depending on what you need to see."
Confocal Microscopy Applications
Confocal microscopy serves as a powerful tool in GFP immunostaining, offering high-resolution imaging capabilities. It provides a unique advantage by eliminating out-of-focus light, resulting in clearer images of specimens. This technique allows for detailed 3D reconstructions of cellular structures, which can reveal more about cellular interactions and dynamics than traditional microscopy.
In applications such as developmental biology, confocal microscopy can track the fate of individual cells over time, giving researchers insight into the mechanisms of development. This granularity can add substantial value in studies examining tissue organization or differentiation, where observing how cells interact spatially and temporally is crucial. The ability to gather optical sections can also enhance data set quality, offering comprehensive insights that are essential for accuracy in subsequent analysis.
Live Cell Imaging Techniques
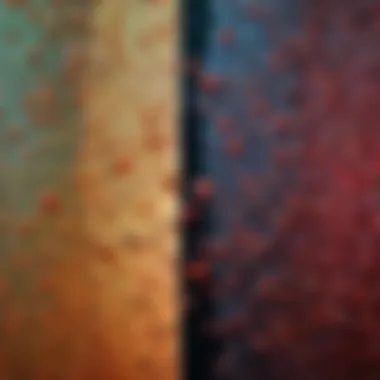
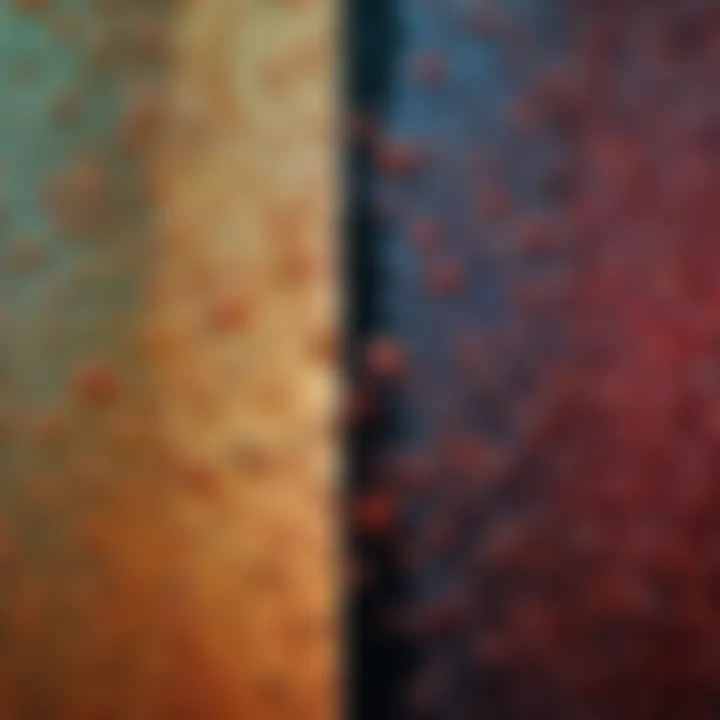
Live cell imaging stands as a groundbreaking aspect of GFP immunostaining, allowing scientists to observe biological processes in real-time. This approach enables dynamic observation of cellular functions and interactions as they occur, providing invaluable data that static studies cannot offer. The use of advanced microscopy combined with GFP-tagging invites researchers to witness events such as cell division, signaling responses, or movement—capturing the essence of life itself in action.
However, live imaging is not without its challenges. Maintaining cell health during observation can be tricky, as excessive light exposure or environmental disturbances can yield artifacts or damage cells. It requires a delicate balance to ensure viable samples while obtaining high-quality images. Efforts to minimize phototoxicity and optimize environmental conditions are critical for successful live imaging studies, making this a continuously evolving field.
Each of these techniques offers distinct advantages and challenges, requiring careful consideration based on the goals of the research. A thoughtful approach to employing these methods can illuminate the path forward in the ongoing exploration of biological phenomena through GFP immunostaining.
Applications of GFP Immunostaining
GFP immunostaining is a cornerstone technique in modern cell biology that has opened up new horizons for research applications. Its ability to provide real-time visualization of protein expression and localization makes it indispensable for investigators delving into the complexities of cellular mechanisms. With its varied use cases ranging from basic research to clinical applications, GFP immunostaining serves as a powerful tool that enhances our understanding of biological processes. Here, we will dissect specific applications of this technique that highlight its significance across multiple fields.
Cell Biology and Developmental Studies
In cell biology, GFP immunostaining allows researchers to track proteins involved in various cellular processes. For instance, in developmental studies, the expression pattern of GFP-labeled proteins can elucidate the pathways that guide cell differentiation and morphogenesis. The time-lapse imaging capabilities of live-cell GFP tagging enable scientists to observe dynamic processes in real-time, providing insights into phenomena like mitosis, apoptosis, or cellular migration.
Using transgenic organisms engineered with GFP constructs facilitates in vivo studies that show how cells interact in their natural environment. For example, the famous zebrafish model has been widely used due to its transparency during early development. Researchers can observe how particular cells develop and migrate, all while tracking specific proteins with GFP. With this method, developmental anomalies and cellular behaviors under different conditions become visible, allowing for more detailed examinations and conclusions.
Pathological Investigations
The importance of GFP immunostaining extends into the realm of pathology as well. It provides crucial information about disease states and mechanisms. By tagging proteins associated with tumorigenesis—such as oncogenes or tumor suppressors—scientists can observe their expression levels and localization in tissue samples. Such insights can help delineate pathological processes underlying diseases like cancer, neurodegenerative disorders, or autoimmune diseases.
In research involving infectious diseases, GFP can be utilized to visualize pathogen behavior within host cells. For instance, tracking the dynamics of a GFP-tagged virus or bacterium can help ascertain how these pathogens invade tissues and evade immune responses. This information could potentially lead to novel therapeutic strategies aiming to disrupt the lifecycle of these pathogens.
Genetic Engineering and Synthetic Biology
The field of genetic engineering has also been significantly influenced by GFP immunostaining. GFP serves as a reporter gene that simplifies the identification of cells or organisms expressing specific genetic constructs. By incorporating GFP into engineered systems, researchers can easily visualize the success of gene-editing endeavors or the expression of transgenes in various cell types.
Synthetic biology has embraced GFP to design versatile biological systems. For example, Escherichia coli or yeast can be engineered to produce GFP in response to certain environmental cues, showcasing how these organisms can change their behavior based on external stimuli. This adaptability provides crucial insights into gene regulatory networks and potential applications in biomanufacturing or biosensing.
"GFP immunostaining becomes a bridge connecting genetics, pathology, and development, illuminating the complex tapestry of life at a molecular level."
"GFP immunostaining becomes a bridge connecting genetics, pathology, and development, illuminating the complex tapestry of life at a molecular level."
Challenges and Limitations
In the realm of GFP immunostaining, acknowledging its challenges and limitations is paramount for researchers and practitioners alike. By thoroughly understanding these hurdles, one can navigate the complexities that arise during the technique's application. It’s not just about the dazzling fluorescence; understanding its imperfections can significantly enhance the reliability of experimental outcomes. Now, let’s dive deep into the specific obstacles that can crop up when using GFP immunostaining in research.
Fluorescence Quenching Issues
Fluorescence quenching stands as one of the foremost challenges in GFP immunostaining. This phenomenon refers to the process where the intensity of fluorescence diminishes, leading to potentially misleading results. During experiments, various factors can contribute to quenching, including environmental influences like pH, temperature, and the presence of specific ions or solvents.
Careful optimization of experimental conditions is essential to mitigate this issue. For instance, maintaining an appropriate pH helps preserve the fluorescent properties of GFP. Moreover, using freshly prepared reagents often yields better fluorescence; old or improperly stored solutions might not perform as expected.
To counteract quenching, researchers often switch to protocols involving antifade reagents, which help stabilize fluorescence under specific conditions. Some even utilize imaging techniques that minimize exposure times during microscopy to reduce photobleaching. Thus, staying vigilant about these factors can enhance the reliability of experimental results while employing GFP.
Antibody Specificity and Cross-Reactivity
Another significant concern revolves around antibody specificity and cross-reactivity. The success of the immunostaining process heavily relies on the antibodies used in detecting GFP. However, the presence of nonspecific binding can lead to false positives, obscuring true biological signals. Cross-reactivity occurs when an antibody binds to similar antigens, potentially causing erroneous interpretations of results.
It's crucial for researchers to choose highly specific antibodies and validate them rigorously using various controls. One effective practice is to employ a knockout system where the target protein is absent, ensuring that any detected signal arises solely from the intended interaction. Additionally, it’s often advisable to run parallel experiments using antibodies targeting different epitopes or proteins to confirm findings. This diligence pays off in the long run, as it bolsters the reliability of data collected from immunostaining assays.
Sample Preparation Artifacts
Sample preparation plays a critically important role in ensuring the integrity of GFP immunostaining results. Artifacts can arise from improper fixation, inadequate permeabilization, or other sample handling mistakes, potentially leading to misinterpretations of the cellular structures under study. Each step in the sample preparation pipeline presents opportunities for variability, which can affect the observed fluorescence.
For avoiding these artifacts, it’s essential to follow standardized protocols closely. Using fresh fixatives and keeping consistency in incubation times and temperatures are necessary practices. Also, the method of sample storage can have profound consequences. Storing samples for extended periods before staining can lead to the degradation of GFP or changes in cellular morphology, affecting results. Researchers should always prioritize optimal sample processing conditions to minimize these inaccuracies.
Future Directions in GFP Immunostaining
The field of GFP immunostaining takes on new life with advancements in both technology and methodology. As researchers dive into the intricate workings of cells, understanding where the field is headed can be transformative. Future directions suggest a richer tapestry of possibilities, bridging gaps between current applications and novel techniques. The exploration of this domain is not just a matter of keeping up with trends but also understanding the underlying implications extracted from these innovations.
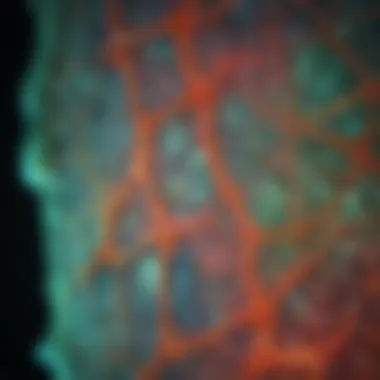
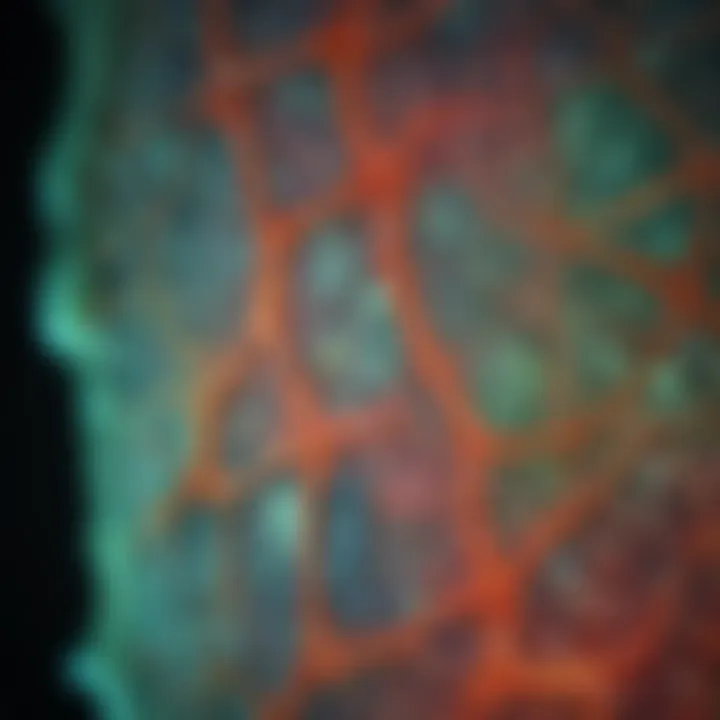
Novel Fluorophores and Technologies
In the constantly evolving landscape of GFP immunostaining, novel fluorophores are making waves. Traditional GFP has been a cornerstone in many studies, yet newer variants and entirely novel fluorophores offer superior properties. For instance, fluorophores with enhanced stability, higher brightness, and a wider range of detectable wavelengths are critical for in-depth cellular studies. The emergence of far-red and near-infrared fluorophores allows for reduced tissue autofluorescence, thus improving the image quality during microscopy. This creates an opening for examples like mNeonGreen or mPink, each tailored for specific applications.
Moreover, chemistries like bioorthogonal labeling are gaining traction. This technology allows for specific labeling of biomolecules within complex biological systems without interfering with cellular activities. A deeper dive into custom labeling strategies enhances the versatility of immunostaining methods.
"The introduction of new fluorophores continuously reshapes the boundaries of what scientists can visualize in living cells."
"The introduction of new fluorophores continuously reshapes the boundaries of what scientists can visualize in living cells."
Selecting the right fluorophore is now as critical as the selection of antibody methods. Researchers have to consider the light exposure, spectral overlap, and retention of fluorescence over time, which will substantially impact the outcomes of their investigations.
Enhancements in Imaging Techniques
As we look ahead, the enhancement of imaging techniques stands as a key player in the advancement of GFP immunostaining. Traditional microscopy methods struggled with resolution limits. However, modern techniques like super-resolution microscopy have lifted this veil, granting scientists the ability to see structures below conventional resolution limits two hundred nanometers.
Methods such as STED (Stimulated Emission Depletion) microscopy and PALM (Photo-Activated Localization Microscopy) provide exciting avenues for observing cellular dynamics with astonishing precision. These methods enable visualization of protein interactions and complex cellular machinations in ways that were previously inconceivable.
Additionally, artificial intelligence and machine learning algorithms are playing an increasingly important role in image analysis. They allow for rapid processing and better pattern recognition in large sets of imagery data, lending clarity to complex biological phenomena. Automation in image acquisition and analysis streamlines workflows, freeing researchers to focus on interpretation rather than tedious manual tasks.
In summary, the future of GFP immunostaining is marked by innovative fluorophores that enhance visibility and novel imaging techniques that break through resolution barriers. These advancements signal an exciting era for molecular biology, promising enriched insights into the dynamic processes that govern life at the cellular level.
Ending and Implications
The study and application of GFP immunostaining serve more than just a technical purpose; they are integral to advancing our understanding of biological systems. The significance of this technology in various fields of research cannot be overstated. Essentially, it provides a unique lens through which we can visualize cellular components, interactions, and processes. This ability to not just see but truly understand cellular dynamics is pivotal in disciplines such as developmental biology, pharmacology, and even clinical diagnostics.
Summarizing the Impact of GFP Immunostaining
The impact of GFP immunostaining is far-reaching and multi-faceted. Here are a few key points that encapsulate its significance:
- Visualization: GFP's vivid fluorescence allows researchers to track proteins and cells in real-time, providing insights into their behavior and interactions within their native environments.
- Diagnostic Applications: In clinical settings, this technique aids in identifying abnormal cells, such as cancerous ones, which can lead to timely interventions and tailored treatments.
- Research Innovation: The versatility of GFP tagging has spurred numerous advances in genetic engineering, leading to breakthroughs in how scientists manipulate and understand genetic information.
Thus, the incorporation of GFP immunostaining in research protocols enhances not only the quality of data obtained but also fosters innovative approaches to problem-solving in biology.
Encouraging Further Exploration in Research
As we continue to delve into the realm of GFP immunostaining, it is crucial for researchers, educators, and students alike to remain inquisitive. Continuing to explore the technology's potential can lead to significant discoveries. Here are some avenues for further exploration:
- New Fluorophores: There is ongoing research into developing novel fluorophores that offer distinct advantages over current GFP variants, including improved brightness, photostability, and spectral range.
- Multicolor Imaging: Expanding upon traditional methods by combining GFP with other fluorescent proteins can greatly enhance the resolution of multiparametric studies, allowing for a more comprehensive understanding of biological systems.
- Integration with Advanced Technologies: Leveraging GFP immunostaining alongside new imaging technologies, such as super-resolution microscopy, can bolster analytical capabilities, providing clearer insights into previously elusive cellular processes.
References and Suggested Readings
In any scholarly discourse, the importance of references and suggested readings cannot be overstated. This section serves as a pivotal foundation for understanding GFP immunostaining and its multifaceted applications. By exploring key literature and emerging studies, researchers, educators, and students can delve into the robust knowledge surrounding GFP, enabling informed exploration and innovation.
Key Literature on GFP
When it comes to grasping the complexities and methodologies involved in GFP immunostaining, the established literature serves as a treasure trove of insights. Key papers and books dissect various aspects of GFP, detailing how it became an essential tool in cellular and molecular biology. Noteworthy works often include discussions around the isolation of GFP from the jellyfish Aequorea victoria, the details of its structure, and the groundbreaking applications that followed its discovery.
Some influential resources worth considering:
- "Green Fluorescent Protein: Properties and Applications" by Tsien 1998: This text provides a comprehensive overview of GFP's photophysical properties and its myriad applications in biological studies.
- "Fluorescent Protein Guided Analysis of Gene Expression" by P. H. Gunter: Here, the author delves into the nuances of using fluorescent proteins to trace gene activity, which is pivotal for understanding developmental biology.
These readings enhance the fundamental understanding of GFP, allowing for a deeper appreciation of its intricacies. Furthermore, they illuminate the evolution of techniques in immunostaining, shedding light on how early challenges have been navigated successfully.
Emerging Studies in the Field
The landscape of GFP technology is continually evolving, propelled by innovative research that expands its scope and capability. Recent studies are illuminating novel applications that were once thought to be the stuff of science fiction. For example, researchers are exploring the integration of GFP with CRISPR technology, which has the potential to revolutionize gene editing and tracing methodologies.
Key aspects to consider include:
- GFP Variants: The development of new fluorophores extending beyond the original GFP can increase versatility in experimental design. New color variants enable multiplexing, permitting the simultaneous visualization of different cellular processes.
- Applications in Pathology: Emerging studies are increasingly applying GFP techniques in clinical settings, providing insights into tumor biology and the development of targeted therapies.
In summary, staying abreast of current studies enables scientists to adopt more refined techniques while contributing to the ongoing dialogue in the scientific community about the efficacy and relevance of GFP immunostaining.
"The quest for knowledge is never-ending; its objective is to close the gap between eras of ignorance and enlightenment."
By keeping an open mind and continuously seeking out the latest research through these references, one fosters a culture of inquiry and discovery that is invaluable in advancing the field of GFP immunostaining.