Understanding the GFP Nucleotide Sequence: Implications and Applications
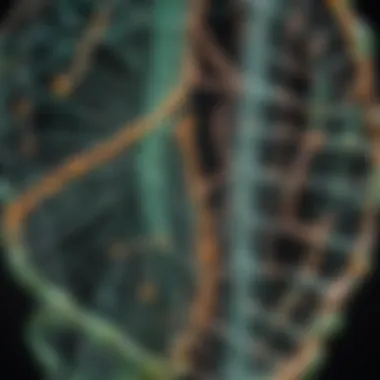
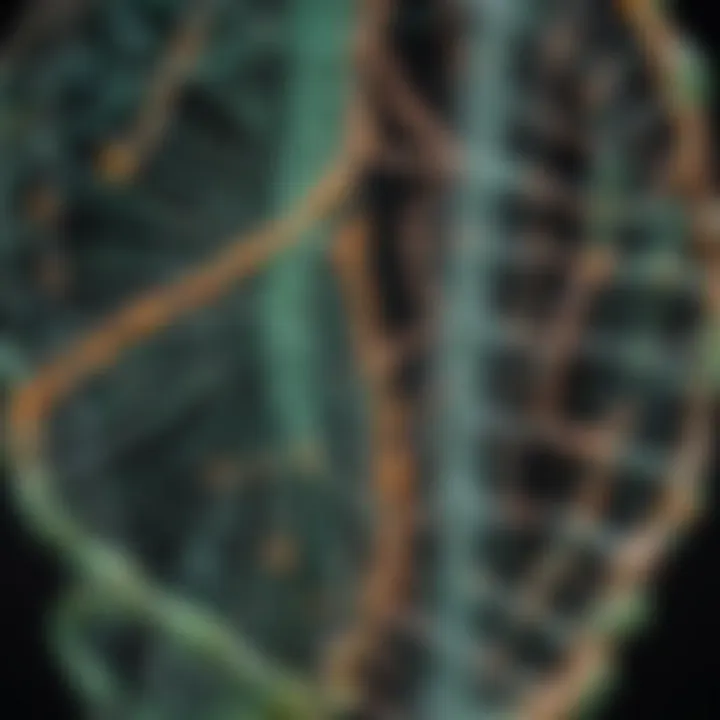
Intro
The green fluorescent protein (GFP) has provided significant insights in molecular biology and biochemistry due to its unique nucleotide sequence. Understanding this sequence is crucial for scientists and researchers working in various fields. The dissecting of GFP's nucleotide structure reveals not just its inherent properties but also the versatile applications it has spawned in scientific research.
This article will explore the genetic blueprint of GFP, its structural characteristics, and the implications of its modifications. Moreover, it will present recent trends in research surrounding GFP, thereby illustrating its impact on modern scientific practices.
Key Concepts
Definition of the Main Idea
GFP is a bioluminescent protein originally found in the jellyfish Aequorea victoria. The nucleotide sequence of GFP codes for this protein, allowing various organisms to express its fluorescent traits. This inherent property has made GFP a popular marker in genetic and cellular studies.
Overview of Scientific Principles
The nucleotide sequence of GFP comprises a series of codons, which are blocks of three nucleotides. These codons are responsible for translating into specific amino acids during the synthesis of the protein. GFP's unique structure includes an original chromophore that is responsible for its fluorescence. Understanding this mechanism involves having a solid grasp of genetic coding, protein folding, and light-matter interactions.
"The simplicity of GFP's structure belies its profound impact on molecular biology and related fields."
"The simplicity of GFP's structure belies its profound impact on molecular biology and related fields."
Current Research Trends
Recent Studies and Findings
Various studies have focused on GFP's nucleotide sequence to create enhanced versions of the protein. These include modifications that allow for improved fluorescent properties or distinct color variations. Research has shown that altering certain base pairs can enhance brightness or stability, thus expanding GFP's utility in live-cell imaging and tracking.
Significant Breakthroughs in the Field
Noteworthy breakthroughs include the development of GFP variants, such as enhanced GFP (eGFP) and other colors like mCherry or mVenus. These innovations have broadened the color spectrum available for use in tagging and imaging cellular processes. Recent advancements also demonstrate the potential of combining GFP with CRISPR technology to visualize gene editing in real time.
By dissecting the details of the GFP nucleotide sequence and its implications, this article aims to present a thorough understanding of GFP's contributions to various scientific disciplines.
Prolusion to GFP
The study of Green Fluorescent Protein (GFP) has become increasingly significant in the fields of molecular biology, genetics, and biochemistry. Understanding the GFP nucleotide sequence offers insights into its structure and functional implications, which are crucial for various scientific applications. It serves as a powerful tool for researchers, enabling them to visualize and track biological processes in real-time. This section aims to lay the groundwork for comprehending the vital role of GFP in scientific research.
Definition of Green Fluorescent Protein
Green Fluorescent Protein, commonly referred to as GFP, is a bioluminescent protein originally derived from the jellyfish Aequorea victoria. It emits bright green light when exposed to ultraviolet or blue light. This unique characteristic can be attributed to its specific amino acid sequence, which can absorb light and then re-emit it at a longer wavelength. The ability of GFP to fluoresce has made it an invaluable marker in various biological studies.
Discovery and Historical Background
The discovery of GFP traces back to 1962 when Osamu Shimomura first isolated this protein from the jellyfish. However, its potential as a biological marker was not recognized until the 1990s when researchers began to explore its applications in live cell imaging and gene expression studies. Douglas Prasher, who worked on cloning the GFP gene, and Martin Chalfie, who helped demonstrate its utility in cells, played pivotal roles in advancing GFP research. In 2008, the Nobel Prize in Chemistry was awarded to Shimomura, Prasher, and Chalfie for their contributions to the field, marking a significant milestone in fluorescence technology.
Understanding the origin and evolution of GFP is essential, as these elements underscore the relevance of the protein in contemporary biological research. The exploration of its nucleotide sequence leads to a greater appreciation of its functional roles, enhancing its applications across multiple domains.
The Structure of the GFP Nucleotide Sequence
The structure of the GFP nucleotide sequence provides critical insights into the functionality and versatility of green fluorescent protein. Understanding this structure is pivotal for applications in molecular biology, biotechnology, and genetic engineering. Knowing how the nucleotide sequences influence the characteristics of GFP enables researchers to tailor its properties for specific applications. Moreover, the structural details can directly impact the study of cellular processes, from tracking protein interactions to exploring cellular environments.
Overview of the GFP Gene
The GFP gene, first isolated from the jellyfish Aequorea victoria, encodes the amino acid sequence that forms the fluorescent protein. Its gene structure is relatively simple, composed of a coding region that directly translates to the protein and regulatory elements that control expression. The coding region contains approximately 720 base pairs, encoding a protein of about 238 amino acids. This structural simplicity belies the versatility GFP has showcased in scientific research.
The sequence's specific arrangement allows for the proper folding of the protein, essential for its fluorescence. This property has positioned the GFP gene as a fundamental tool in various biological studies, enabling experiments that require visualization of live cells and dynamic processes.
Key Components of the Nucleotide Sequence
The nucleotide sequence of GFP consists of several key components. Each nucleotide comprises a base (adenine, thymine, cytosine, or guanine), a sugar molecule, and a phosphate group. The precise order of these nucleotides determines the amino acids in the synthesized protein. This order is critical for achieving the desired fluorescent characteristics and stability of the protein.
In addition to the coding sequence, the GFP gene includes untranslated regions (UTRs) at both ends. These regions play essential roles in regulating gene expression and translation efficiency. Features such as the promoter region, which initiates transcription, and the terminator region, which signals the end, are also critical for the effective expression of GFP in host organisms.
"A clear understanding of the GFP nucleotide sequence unlocks potential innovations in science and medicine."
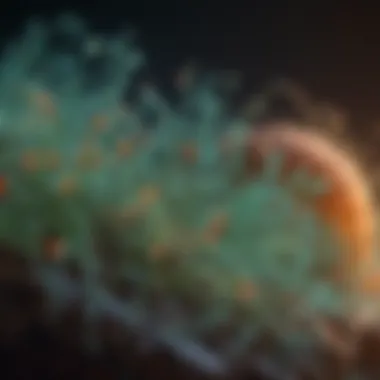
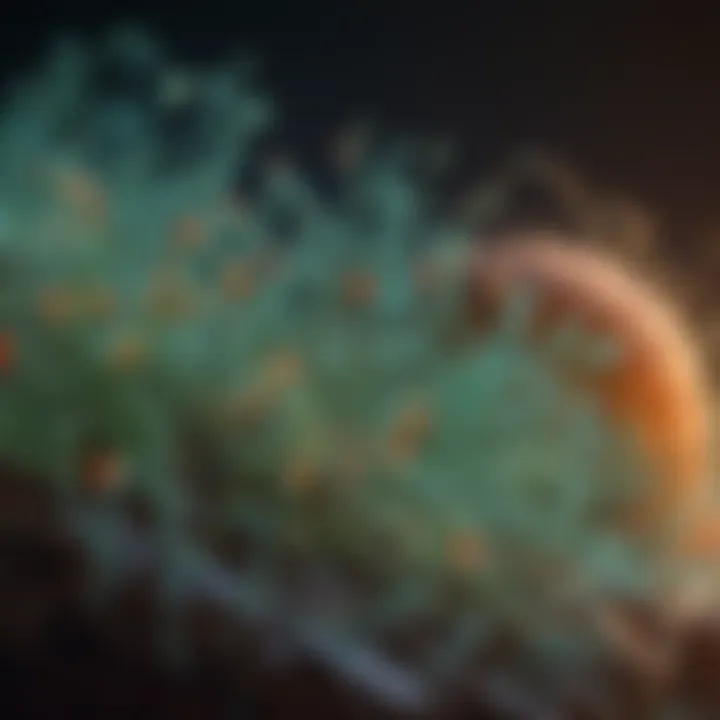
"A clear understanding of the GFP nucleotide sequence unlocks potential innovations in science and medicine."
Transcription and Translation Processes
The processes of transcription and translation are vital to the expression of the GFP gene. During transcription, the DNA sequence of the GFP gene is copied into messenger RNA (mRNA). This mRNA serves as an intermediary, carrying the genetic information from the nucleus to the ribosomes in the cytoplasm, where translation occurs.
In translation, ribosomes read the mRNA sequence and assemble amino acids in the order dictated by the mRNA. The result is the formation of the GFP protein. This protein will later undergo folding and post-translational modifications to achieve its functional form. The efficiency of these processes can be influenced by the nucleotide sequence itself, including the presence of regulatory elements that modulate how frequently the gene is expressed.
Sequencing Techniques for GFP
The sequencing of the GFP nucleotide sequence is a pivotal process that allows researchers to understand the intricate details of this protein at a molecular level. Understanding the sequence not only provides insights into the protein's structure and function but also opens new avenues for its application in various scientific fields. Through sequencing, scientists can identify mutations, predict structural changes, and explore how alterations to the sequence may affect protein functionality. This is essential for advancing biotechnological applications and enhancing the utility of GFP as a molecular tool.
Traditional Sequencing Methods
Traditional methods of sequencing GFP include techniques such as Sanger sequencing. This method, developed by Frederick Sanger in the 1970s, relies on chain-termination principles to selectively determine nucleotide sequences. Sanger sequencing has been highly reliable and cost-effective for sequencing smaller DNA fragments. However, it has limitations in terms of throughput and the amount of data it can handle. Typically, it requires labor-intensive processes that can be time-consuming when dealing with larger segments of DNA.
Using these methods, researchers can obtain reliable sequence data for GFP genes. The enzymatic incorporation of dideoxynucleotides terminates chain elongation and provides precise locations of DNA bases. This approach is critically useful in elucidating mutations that may arise during laboratory manipulations or naturally in biological contexts.
- Pros of Traditional Sequencing:
- Cons of Traditional Sequencing:
- High accuracy
- Extensive validation and optimization over years
- Limited scalability
- More time-consuming for extensive genomic work
Next-Generation Sequencing Technologies
Next-generation sequencing (NGS) has revolutionized the speed and scale of sequencing compared to traditional methods. NGS platforms, such as Illumina and PacBio, can process millions of DNA fragments simultaneously. This massive parallelization significantly enhances throughput, allowing researchers to sequence entire genomes in a matter of days.
For GFP, NGS technologies facilitate large-scale sequencing applications. They enable researchers to investigate variations within the GFP gene sequence across different species or to analyze the effects of mutations in real-time. This has profound implications in fields such as comparative genomics, synthetic biology, and protein engineering.
Next-generation sequencing allows for unprecedented insight into the functional diversity of GFP and provides a toolkit for innovative research practices.
Next-generation sequencing allows for unprecedented insight into the functional diversity of GFP and provides a toolkit for innovative research practices.
- Advantages of Next-Generation Sequencing:
- Challenges of Next-Generation Sequencing:
- High-throughput capabilities
- Cost-effective for large-scale projects
- Requires significant data management and bioinformatics expertise
- Initial setup costs can be high
In summary, the evolution from traditional sequencing methods to next-generation sequencing technologies marks a significant step forward in GFP research. Each approach has its benefits and challenges, influencing the choice of method based on the specific research objectives. The advancing field of sequencing not only enhances our understanding of GFP but also expands its applications in science and industry.
Functional Significance of GFP
The functional significance of Green Fluorescent Protein (GFP) extends far beyond its captivating glow. This protein has become an indispensable tool in a variety of scientific fields. By understanding its role and implications, researchers can harness its properties for innovative applications.
Role in Cellular Biology
GFP plays a crucial role in cellular biology, acting as a powerful visual marker. It allows scientists to track biological processes in real-time within living cells. By attaching GFP to specific proteins, researchers can observe the dynamics of cellular activities, such as protein localization, movement, and interactions. This capability has provided deeper insights into cellular mechanisms and pathways.
- Tracking Cellular Processes: Researchers can visualize events like mitosis or apoptosis, enabling the study of cell division and death mechanisms.
- Protein Interactions: GFP can be used in techniques like Fรถrster Resonance Energy Transfer (FRET) to explore interactions between proteins. This offers a dynamic view of how proteins communicate within the cellular environment.
- Genetic Studies: The integration of GFP in genes allows for the examination of gene expression patterns, essentially making invisible cellular processes observable. This has transformed our understanding of developmental biology and disease progression.
Applications in Live Cell Imaging
Live cell imaging is another significant application of GFP. This technique relies on the fluorescent properties of GFP to visualize living cells without altering their behavior. By imaging cells in real-time, researchers can gather quantitative data about cellular events.
- Dynamic Observations: Unlike fixed imaging techniques, live cell imaging reveals the dynamics of cellular processes, offering a more accurate view of biological functions.
- Drug Testing: GFP serves as an effective marker for evaluating cellular responses to pharmacological compounds, assisting in drug discovery and development.
- Disease Modeling: By studying how cells respond to diseases in real-time, researchers can develop better models for diseases like cancer and neurodegenerative disorders.
Modifications and Variants of GFP
The GFP nucleotide sequence finds its significance extended beyond its native form through a range of modifications and novel variants. Understanding these adaptations is essential in the expansion of its applications in modern science and technology. This section delves into two important subcategories: site-directed mutagenesis and the creation of novel fluorescent protein variants. Each method offers unique benefits and considerations that are vital to researchers and practitioners in the field.
Site-Directed Mutagenesis
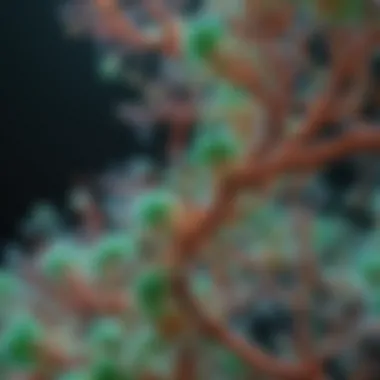
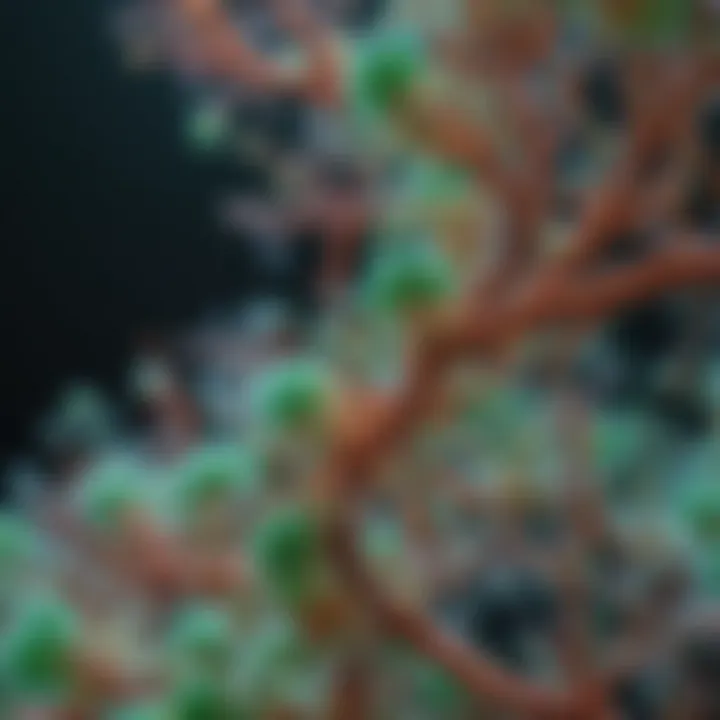
Site-directed mutagenesis is a powerful technique used to alter specific nucleotide sequences within the GFP gene. This precise alteration allows scientists to create specific mutations that can enhance or change the properties of the resultant protein. For instance, mutating particular amino acids can affect the fluorescence intensity, spectral properties, or stability of GFP.
The process begins with the design of oligonucleotide primers containing the desired mutations. These primers are incorporated into the PCR (polymerase chain reaction) process, amplifying the modified DNA sequence. Following amplification, the original DNA template is removed, resulting in a plasmid that contains the mutated version of the GFP gene.
Key Benefits of Site-Directed Mutagenesis:
- Customization: Researchers can precisely tailor the protein properties to fit specific experimental needs.
- Functional Analysis: By creating specific mutations, scientists can investigate the role of certain amino acids in protein function.
- Improved Characteristics: Enhanced solubility, stability, and other properties can be achieved through strategic mutations.
Creation of Novel Fluorescent Protein Variants
The exploration of GFP has led to the development of many novel fluorescent protein variants. These proteins often come from natural sources, like marine organisms, or are engineered through mutagenesis techniques. Each variant typically exhibits distinct fluorescence characteristics, which can be beneficial in various applications.
Some notable examples include:
- mCherry: Known for its red fluorescence, mCherry has been widely used in multi-color imaging studies alongside GFP.
- Supernova: This variant offers enhanced brightness and photostability, making it ideal for prolonged imaging experiments.
- Cerulean: With a blue emission, this variant allows researchers to label multiple proteins in cellular contexts without overlap.
The creation of these variants usually involves systematic screening of libraries of mutated GFP genes. The selected proteins undergo extensive characterization to assess their unique properties and potential applications.
End of Modifications
In summary, the modifications and variants of GFP significantly influence its utility in research. Techniques such as site-directed mutagenesis allow for targeted changes, while the creation of novel variants broadens the spectrum of fluorescent proteins available to researchers. The diverse applications stemming from these adaptations continue to advance multiple fields, including cellular biology, genetics, and biotechnology.
Changes in the GFP nucleotide sequence can drastically affect its functionality, showcasing the intricate relationship between genetic structure and protein properties.
Changes in the GFP nucleotide sequence can drastically affect its functionality, showcasing the intricate relationship between genetic structure and protein properties.
Applications in Molecular Biology
The role of the green fluorescent protein (GFP) in molecular biology cannot be overstated. It has become a cornerstone of various experimental techniques that allow researchers to observe and understand cellular processes in real-time. The applications of GFP are primarily centered around gene expression and protein interactions, serving critical functions in both basic and applied research fields.
Tracking Gene Expression
Tracking gene expression is crucial for understanding how genes are turned on and off within a cell. GFP serves as a powerful reporter gene, providing visual evidence of gene activity. When a specific gene is linked to the GFP sequence, the expression of that gene can be monitored by observing the fluorescence emitted in live cells. This visualization allows scientists to determine:
- When a gene is expressed during development.
- Where in the organism the gene shows activity.
- How strong the expression is, indicating the levels of transcription.
This method has revolutionized studies in developmental biology, gene therapy, and tissue engineering, enabling researchers to track complex biological processes without disrupting the natural environment of the cell. The ability to visualize gene expression in living organisms opens new avenues for understanding the dynamics of diseases and the effectiveness of treatments.
Understanding Protein-Protein Interactions
Protein-protein interactions are integral to numerous cellular processes, including signaling pathways and metabolic functions. Researchers use GFP to study these interactions through methods like FRET (Fรถrster Resonance Energy Transfer). In this approach, two proteins of interest can be tagged with different fluorescent proteins. When these proteins interact, the energy transfer between the two fluorophores can be measured, yielding valuable insights into:
- The kinetics of protein interactions.
- The localization of proteins within the cell.
- Changes in interaction dynamics under varying conditions or stimuli.
The ability to observe these interactions in real-time allows for a deeper understanding of complex cellular mechanisms and the identification of potential therapeutic targets.
"GFP not only illuminates cellular activities but also sheds light on the intricate workings of life at the molecular level."
"GFP not only illuminates cellular activities but also sheds light on the intricate workings of life at the molecular level."
GFP in Biotechnology
Green Fluorescent Protein (GFP) has emerged as a crucial element in the field of biotechnology. Its unique properties and capabilities make it an invaluable tool in various applications, cutting across different scientific and industrial disciplines. The implications of GFP in this domain are extensive, influencing not only the way research is conducted but also how results are interpreted and utilized.
GFP as a Reporter Gene
One of the most significant roles of GFP in biotechnology is its function as a reporter gene. A reporter gene is a gene that researchers attach to a regulatory sequence of another gene of interest in a cell. The presence of GFP allows scientists to visualize and monitor gene expression and activity in real-time.
- Non-invasive Monitoring: Traditional methods for observing cellular processes often require tissue destruction. GFP, however, allows for the observation of living cells without such invasive procedures.
- Quantitative Analysis: The fluorescence emitted by GFP can be quantitatively measured. This provides a reliable metric for gene activity, allowing researchers to draw meaningful comparisons across experiments.
- Applicability Across Species: GFP has been successfully used in various organisms, from bacteria to mammals. This versatility broadens the scope of research and enhances the comparability of results across different biological systems.
Using GFP as a reporter gene has transformed the landscape of molecular biology, providing clarity and depth to gene expression studies.
Using GFP as a reporter gene has transformed the landscape of molecular biology, providing clarity and depth to gene expression studies.
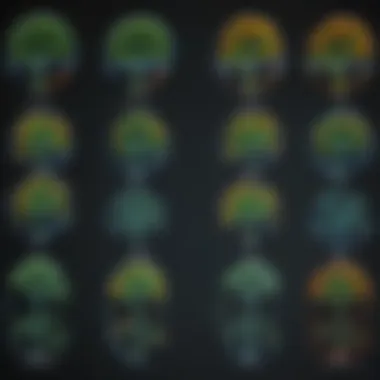
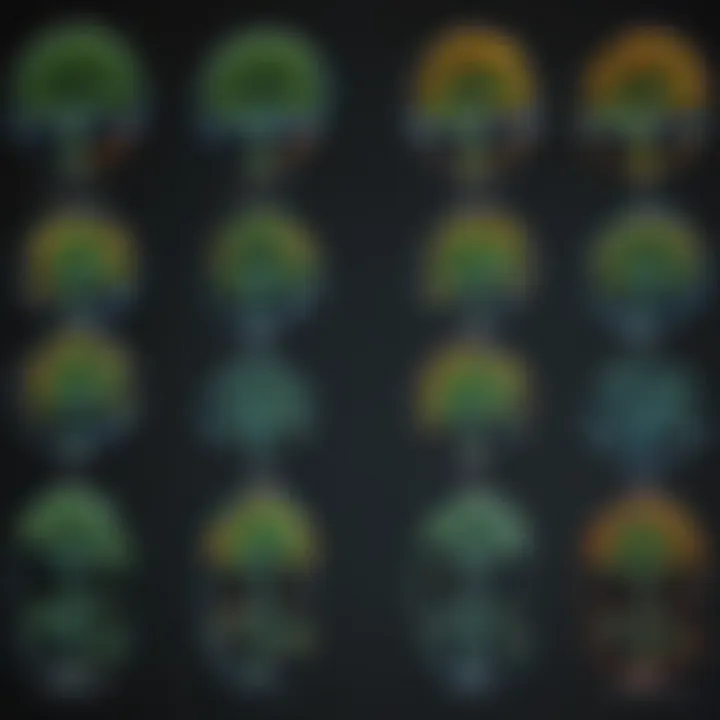
Industrial Applications of GFP
Apart from fundamental research, GFP holds considerable promise in industrial applications as well. Its utility extends to several sectors, including pharmaceuticals, agriculture, and environmental science.
- Biopharmaceuticals Production: In the pharmaceutical industry, GFP can be utilized for the tracking and identification of recombinant proteins. This is critical for the production of therapeutics, ensuring that the proteins produced are correctly folded and functional.
- Agricultural Biotechnology: GFP can facilitate the development of genetically modified organisms (GMOs). By tagging specific traits with GFP, researchers can easily select for crops that express desired characteristics, such as pest resistance or enhanced nutritional content.
- Environmental Monitoring: GFP can be employed in environmental biotechnology to monitor pollution levels. For instance, bacteria that are modified to express GFP in the presence of certain pollutants can serve as biosensors, providing immediate feedback on environmental health.
In summary, the integration of GFP into biotechnology showcases its significance as both a research tool and an industrial agent. Its role spans from fundamental science to practical applications, underscoring its versatility and importance in advancing both scientific understanding and practical solutions.
Ethical Considerations in GFP Research
Ethical considerations play a critical role in scientific research, particularly in studies involving genetically modified organisms and bioluminescent proteins like GFP. The use of GFP raises significant questions about biosafety, environmental influences, and the broader societal implications of genetic engineering. These issues ensure that researchers maintain a responsible approach when employing GFP in experiments or applications.
Biosafety Issues
Biosafety encompasses the policies and practices that ensure safe handling, use, and disposal of biological materials. In GFP research, biosafety considerations are crucial for several reasons:
- Potential Risks: The introduction of genetically modified organisms can yield unforeseen consequences. Adequate measures must be in place to mitigate risks of contamination or unintended effects on ecosystems.
- Containment Protocols: Adhering to strict containment protocols helps prevent the escape of engineered organisms into wild populations. Facilities must implement specific guidelines to manage these organisms effectively during research.
- Pathogen Safety: When using GFP in pathogenic organisms, it is crucial to assess any risks posed to human health or the environment. Attention must be given to how GFP alters the behavior or virulence of these pathogens.
The biosafety aspect does not just ensure the success of research; it safeguards public health and environmental integrity. Researchers must continuously scrutinize their methodologies to uphold these standards.
Environmental Impact Assessments
Assessing environmental impacts is integral to understanding how GFP affects ecosystems. With its wide array of applications, from tracking gene expression to bioremediation, informed assessments help indicate both benefits and potential adverse effects:
- Ecosystem Dynamics: Introducing GFP into non-native species may disrupt local ecosystems. Monitoring ecological relationships can highlight any negative implications on biodiversity.
- Resource Utilization: Studies that introduce GFP into organisms involved in agriculture could lead to changes in resource utilization. This can impact food supply and pest management strategies.
- Regulatory Compliance: Environmental impact assessments are often mandated by regulatory agencies before proceeding with such research projects. Conducting systematic evaluations allows researchers to navigate compliance effectively.
"Ethics is knowing the difference between what you have a right to do and what is right to do."
โ Potter Stewart
"Ethics is knowing the difference between what you have a right to do and what is right to do."
โ Potter Stewart
Engaging with these ethical dimensions of GFP research will enhance the credibility and societal acceptability of the findings, encouraging responsible innovation in this dynamic field.
Future Directions in GFP Research
The exploration of green fluorescent protein (GFP) has significantly advanced scientific understanding in various disciplines. The future directions in GFP research present numerous opportunities for further innovations and applications. Researchers are focusing on enhancing the properties of GFP and discovering new uses across multiple scientific fields. This section delves into the potential innovations in fluorescent proteins and the possibilities of therapeutic applications, which could expand the horizons of GFP's utility.
Innovations in Fluorescent Proteins
Future studies on GFP will likely concentrate on the development of novel fluorescent proteins with enhanced characteristics. New types of fluorescent proteins can improve the versatility of tagging and imaging in various biological systems. Some specific areas of innovation might include:
- Increased brightness and stability: Fluorescent proteins that exhibit higher brightness levels and more robust photostability will allow for clearer imaging over extended periods.
- Color variants: Developing new fluorescent proteins with distinct emission spectra can enable simultaneous multicolor imaging. This differentiation in color allows researchers to track multiple biological processes in real time.
- Improved targeting capabilities: Innovations that enhance the specificity of fluorescent proteins would aid in directing them to specific cellular locations, improving their usefulness for studying complex interactions within cells.
- Environmentally responsive proteins: Fluorescent proteins that respond to changes in their environment, such as variations in pH or ion concentrations, could provide valuable insights into cellular processes.
Advancements in these areas could make GFP and its variants not only more efficient for research but also more applicable in practical settings, providing fresh perspectives on existing biological questions.
Potential for Therapeutic Applications
The potential for therapeutic applications of GFP is a significant area of interest. This extends beyond research into tangible benefits in medicine. Key considerations include:
- Gene therapy: Using GFP to monitor the success of gene therapy approaches, researchers can track the expression of therapeutic genes within target tissues.
- Cancer treatment: Innovations in GFP may lead to the creation of targeted cancer therapies that utilize fluorescent tagging to distinguish between malignant and healthy cells during surgical procedures.
- Drug delivery systems: Developing fluorescent proteins that can act in conjunction with drugs offers a method to visualize the distribution and effectiveness of treatments across various cell types.
"The progressive application of GFP in therapeutic contexts showcases its evolution from a research tool to a potential lifesaving technology."
"The progressive application of GFP in therapeutic contexts showcases its evolution from a research tool to a potential lifesaving technology."
Overall, the implications of these advancements in GFP research are profound. As scientists delve deeper into the genetic mechanisms underlying fluorescent proteins, we can expect to see novel applications arise that benefit a myriad of fields, from molecular biology to medicine. The ongoing exploration of GFP will certainly continue to unveil its full potential, facilitating significant breakthroughs in our understanding and treatment of various biological conditions.
Closure
In summarizing the exploration of the green fluorescent protein (GFP) nucleotide sequence, it becomes clear that this topic carries significant weight in modern scientific research. The ability for GFP to serve as a marker in various applications underscores its value. Researchers and scientists leverage GFP for tracking biological processes with precision and efficiency. As this article demonstrates, understanding the structure and function of GFP provides insights essential for advancing techniques in molecular biology and biochemistry.
Recap of Key Points
- The GFP nucleotide sequence plays a crucial role in the protein's fluorescence, which is vital for live cell imaging.
- Several techniques for sequencing and modifying GFP have emerged, driving the evolution of fluorescent protein applications.
- Ethical considerations must be addressed, particularly concerning biosafety and environmental impact, to ensure responsible use in research.
- Innovations in GFP research point towards exciting future applications in therapy and diagnostics.
The Importance of GFP in Scientific Research
Green fluorescent protein is not just a tool; it is a transformative element in biology. The importance of GFP in scientific research lies primarily in its versatility and utility.
- Tracking Mechanisms: The GFP sequence allows scientists to visualize and track cellular components in real-time. This visualization aids in understanding complex biological processes.
- Research Innovation: New GFP variants continue to emerge, enhancing the scope of what can be studied. These innovations maintain GFP's relevance in cutting-edge research.
- Broader Implications: Beyond academic research, GFP's applications in biotechnology and medical research showcase its broader implications for health and technology.