Gibson Assembly Method: A Comprehensive Overview
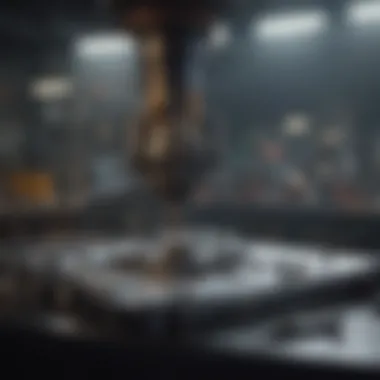
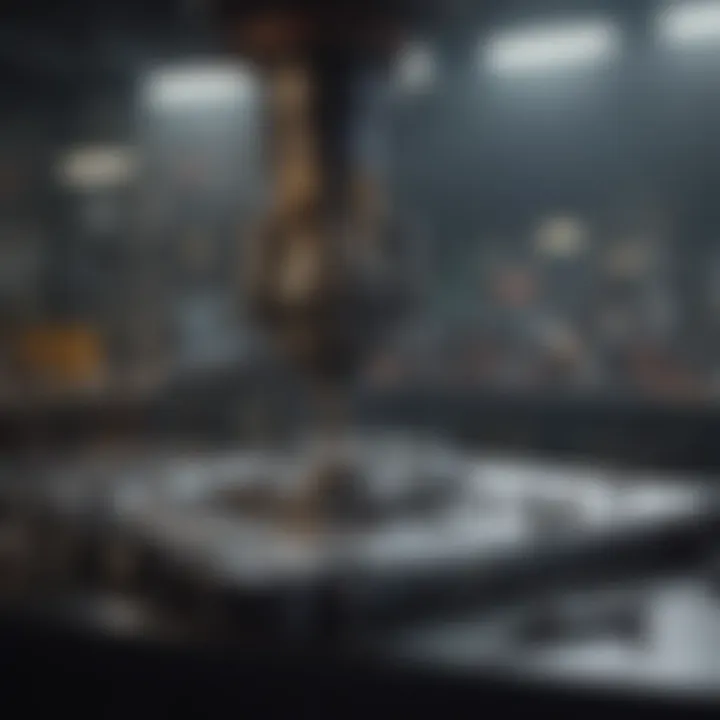
Intro
Gibson Assembly represents a pivotal advancement in molecular biology, particularly in the realm of DNA manipulation. This method provides an efficient way to join multiple DNA fragments seamlessly. Unlike traditional methods, Gibson Assembly minimizes the requirements for restriction enzymes and ligases. It allows scientists to construct complex DNA sequences with precision and ease.
Researchers in genetic engineering, synthetic biology, and genome editing utilize this technique extensively. The ability to join DNA pieces without the necessity for homologous ends enhances flexibility and speeds up the cloning process. Understanding the key concepts behind this method is crucial for both students and experienced professionals looking to innovate in their work.
Key Concepts
Definition of the Main Idea
Gibson Assembly involves the use of specially designed reagents to enable the overlap of DNA sequences for assembly without needing traditional restriction enzyme digestion. The method was developed by Dr. Daniel Gibson and his colleagues, providing a powerful tool for synthesizing DNA that adheres to desired sequences.
Overview of Scientific Principles
The plethora of principles underlying Gibson Assembly includes:
- Overlapping sequences: DNA fragments must have overlapping ends to ensure proper assembly. Typically, these overlaps are around 20-40 base pairs long.
- Temperature-controlled reaction: The reaction occurs in a single step at a moderately elevated temperature, facilitating the joining of fragments efficiently.
- Enzymatic action: The method employs specific exonucleases, DNA polymerases, and ligases to create a seamless bond between the DNA fragments.
Essentially, these principles converge to provide a method that is both straightforward and accurate, catering to a variety of experimental needs.
Current Research Trends
Recent Studies and Findings
Recent literature reveals a surge of interest in refining Gibson Assembly for broader applications. Studies indicate improvements in the efficiency of assembling larger constructs. For instance, innovations in enzyme formulations have led to enhanced yields in complex assembly reactions.
"The adaptability of the Gibson Assembly method has made it a foundational technique in genetic research."
"The adaptability of the Gibson Assembly method has made it a foundational technique in genetic research."
Significant Breakthroughs in the Field
Prominent breakthroughs include:
- High-throughput applications: Researchers are applying this method to create genetic libraries on a large scale.
- Medical applications: Gibson Assembly is instrumental in developing gene therapies and vaccines, particularly for emerging diseases.
These advancements illustrate the dynamic nature of research surrounding Gibson Assembly, highlighting its relevance and capabilities in modern molecular biology.
Intro to Gibson Assembly
The introduction of the Gibson Assembly technique marks a significant advancement in the field of molecular biology. It offers researchers a powerful tool for constructing complex DNA molecules efficiently and seamlessly. This section will explore the definition and purpose of Gibson Assembly, as well as its historical background, providing context for its importance in modern genetic engineering.
Definition and Purpose
Gibson Assembly is a method used to join multiple DNA fragments based on overlapping sequences. Unlike traditional cloning methods, it does not require restriction enzymes or separate ligation steps. The technique allows the assembly of entire plasmids or larger constructs in a single reaction. This is advantageous for various applications such as synthetic biology and genome editing, where precision and efficiency are crucial.
The core purpose of Gibson Assembly is to enable the creation of complex genetic constructs with high fidelity. By utilizing enzymatic reactions, it facilitates the smooth joining of DNA fragments, reducing the time needed for multi-fragment assembly. This method also increases the potential for error-free constructs, which is important for downstream applications like protein expression or functional studies.
Historical Background
Gibson Assembly was first described by Daniel Gibson and his colleagues in 2009. This technique emerged from a growing need for more efficient DNA assembly methods that could handle the increasing complexity of genetic constructs. Prior to this invention, researchers relied heavily on methods such as restriction enzyme cloning and traditional ligation, which often resulted in low efficiency and significant labor.
The introduction of Gibson Assembly represented a paradigm shift. It combined three enzymatic activities in a single reaction: exonuclease treatment to create overlapping ends, polymerase to fill in gaps, and ligase to seal nicks in the DNA backbone. This streamlined approach not only simplified the crafting of DNA constructs but also contributed to the rapid advancements in fields like synthetic biology, where large-scale DNA assembly is often required.
“Gibson Assembly has revolutionized the way we construct DNA, facilitating a new era of genetic engineering.”
“Gibson Assembly has revolutionized the way we construct DNA, facilitating a new era of genetic engineering.”
This historical innovation illustrates how Gibson Assembly has become a staple methodology for both academic and industrial applications, paving the way for future genetic advancements.
Principles of Gibson Assembly
In understanding the Gibson Assembly method, one must grasp its fundamental principles. This technique revolutionizes DNA manipulation through a seamless joining of DNA fragments. By eliminating the reliance on traditional restriction enzymes, Gibson Assembly offers a more effective and flexible approach to cloning. Its significance extends across diverse fields, including genetic engineering, synthetic biology, and genome editing. The principles that underpin this methodology are essential for anyone looking to harness its capabilities effectively.
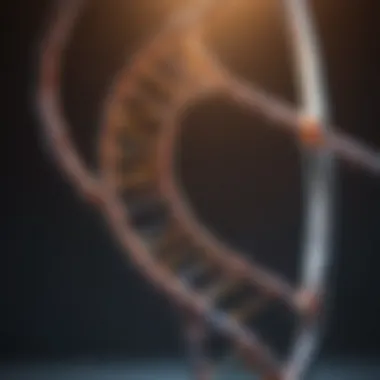
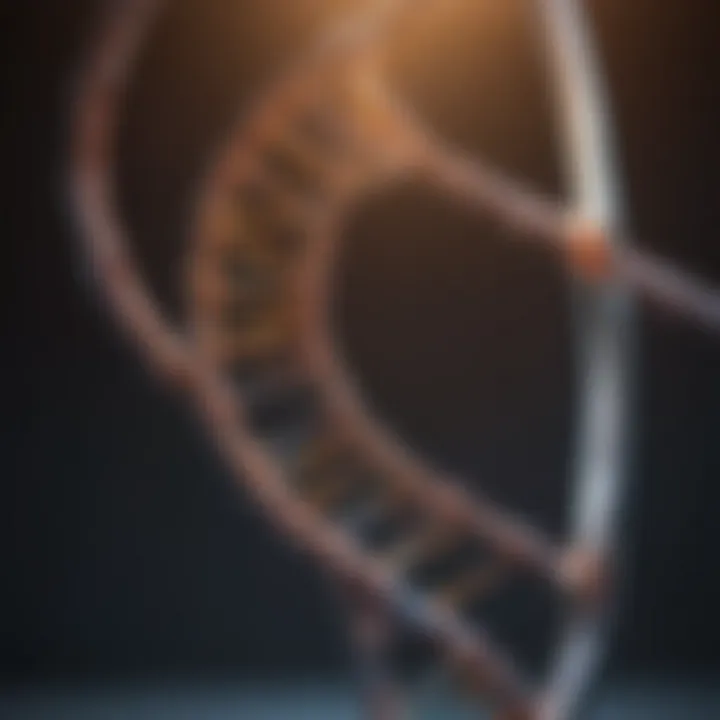
Mechanism of Action
The mechanism of action in Gibson Assembly centers around the strategic overlapping of DNA fragments. Typically, the desired fragments are designed with complementary ends that allow them to align accurately. When these fragments are mixed, a specific enzyme mixture initiates the assembly process. This mixture usually contains an exonuclease, a DNA polymerase, and a DNA ligase. The exonuclease trims the ends, generating cohesive overhangs. Following this, the DNA polymerase fills in any gaps, ensuring that all nucleotides are matched precisely. Finally, the ligase seals the sugar-phosphate backbone. This elegant combination of enzymatic activities results in a complete, joined DNA molecule.
Key Components and Enzymes
The success of Gibson Assembly relies on several crucial components and enzymes. First, the exonuclease plays a vital role in creating the complementary overhangs on the DNA fragments. This activity is pivotal, as it prepares the fragments for assembly by exposing the sequences that will anneal. Next, the DNA polymerase serves to fill in any gaps that appear during the assembly. This enzyme is crucial because it provides the necessary fidelity and efficiency in matching the nucleotide sequences.
Lastly, the DNA ligase facilitates the closure of the DNA backbone to create a continuous strand. It is noteworthy that these enzymes function optimally at specific temperatures, generally around 50 degrees Celsius, emphasizing the importance of adhering to proper thermal conditions during the assembly process.
"Gibson Assembly allows for a streamlined approach to genetic engineering, reducing the complexity often associated with traditional methods."
"Gibson Assembly allows for a streamlined approach to genetic engineering, reducing the complexity often associated with traditional methods."
Overall, the principles of Gibson Assembly, including its underlying mechanism and key components, showcase its innovative approach to DNA manipulation. By understanding these elements, researchers can better utilize this powerful tool in their scientific endeavors.
Gibson Assembly Protocol
The Gibson Assembly protocol serves as a vital method for researchers who aim to join multiple DNA fragments with precision and efficiency. This protocol streamlines the process of DNA assembly by overcoming the limitations associated with traditional cloning methods. Unlike those older techniques that may rely on restriction enzymes, Gibson Assembly allows for a seamless joining of DNA sequences, making it particularly advantageous in synthetic biology and genome editing fields.
Preparation of DNA Fragments
In preparing DNA fragments for Gibson Assembly, it is important to ensure that the fragments possess overlapping sequences. These sequences typically range from 20 to 40 base pairs in length. Preparing these fragments usually involves PCR amplification or the use of exonucleases. The use of exonucleases is notable as they can create specific 5' overhangs, which are essential for the later assembly reactions.
When designing the primers for PCR, researchers must consider the overlap intentionally. Precision in calculating the overlap length helps to ensure that the resulting fragments can anneal effectively, which is critical for a successful assembly. It is also advisable to assess the quality and concentration of the DNA fragments. Purification steps, such as gel extraction or column purification, might be necessary to remove contaminants and ensure that only the desired DNA fragments are used in the assembly.
Assembly Reactions
The assembly reactions in the Gibson Assembly protocol are straightforward yet critical for achieving the desired outcomes. The prepared DNA fragments are mixed with a master mix containing specific enzymes that facilitate the assembly process. T5 exonuclease, which has the capability to create single-stranded sticky ends, plays a key role alongside DNA polymerase and DNA ligase. This master mix incubates at a specific temperature, usually around 50 degrees Celsius, allowing for the fragments to anneal together at their complementary regions.
During this incubation period, the overlapping sequences anneal, followed by the action of DNA polymerase filling in the gaps. Lastly, DNA ligase seals the nicks in the assembled DNA, resulting in a covalently closed construct. The efficiency of this protocol often yields successful constructs in a shorter timeframe compared to traditional methods.
Combining the efficiency of Gibson Assembly with thoughtful preparation of DNA fragments can significantly enhance success rates in molecular cloning.
Combining the efficiency of Gibson Assembly with thoughtful preparation of DNA fragments can significantly enhance success rates in molecular cloning.
Overall, the careful preparation of DNA fragments and the execution of assembly reactions encapsulate the essence of the Gibson Assembly protocol.\nTheir combined efficiencies mean this method has become a substitute for many other approaches in genetic engineering.
Advantages of Gibson Assembly
The Gibson Assembly method stands out in molecular biology due to its numerous advantages. This technique offers a wide range of benefits that enhance DNA manipulation and construction processes. Understanding these advantages is crucial for scientists, researchers, and professionals looking to optimize their work in genetic engineering and synthetic biology. Here, we explore two primary advantages—high efficiency and accuracy, and seamless cloning.
High Efficiency and Accuracy
Gibson Assembly is known for its ability to efficiently join multiple DNA fragments in a single reaction. This efficiency translates into an increased rate of success compared to traditional methods, which often require several separate steps. The key factors contributing to this high efficiency include:
- Single Step Reaction: Gibson Assembly occurs in a single reaction vessel, reducing the time and resources required for fragment assembly.
- Overlap-Based Joining: Each DNA fragment includes overlapping regions, allowing for precise annealing. This increases the likelihood that the correct fragments will successfully join together.
- Reduced Error Rate: The enzymatic process minimizes the chances of inserting or deleting nucleotides, leading to highly accurate constructs.
These aspects make Gibson Assembly particularly advantageous in applications where precision is essential, such as genome editing and the creation of complex genetic circuits.
Seamless Cloning
A critical benefit of Gibson Assembly is its capability for seamless cloning. Traditional cloning methods often leave vector scars or unwanted sequences at the junction points of the assembled fragments. In contrast, Gibson Assembly facilitates the joining of DNA fragments without leaving behind extraneous sequences. Key points include:
- No Need for Restriction Enzymes: Unlike traditional cloning, which relies on restriction enzymes that can create cuts with specific overhangs, Gibson Assembly relies on overlapping regions. This eliminates the inherent limitations of restriction sites.
- Versatile Fragment Joining: Researchers can join fragments of varying lengths and sequences without the concern of compatibility, allowing for more creativity in genetic engineering efforts.
- Improved Functional Validation: The absence of nucleotide scars enhances the functionality of the new DNA constructs. This increases the chances that engineered genes will express correctly and perform as intended in biological systems.
In summary, the advantages of Gibson Assembly make it a superior method for DNA assembly in many applications, including genetic engineering and synthetic biology. Its high efficiency, accuracy, and the ability to perform seamless cloning are critical for researchers aiming to manipulate DNA with a high degree of precision.
"The efficiency and seamless nature of Gibson Assembly enable rapid and precise DNA construction, transforming molecular biology practices."
"The efficiency and seamless nature of Gibson Assembly enable rapid and precise DNA construction, transforming molecular biology practices."
Comparative Analysis
In the realm of molecular biology, a comparative analysis of various methods is essential. It provides significant context and understanding when evaluating the effectiveness of Gibson Assembly. By contrasting this innovative technique with traditional cloning methods and other assembly procedures, we can appreciate its unique advantages and limitations. Such analyses highlight the scenarios where Gibson Assembly proves superior and where alternative approaches might still hold value.
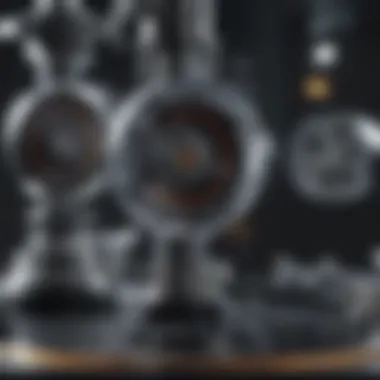
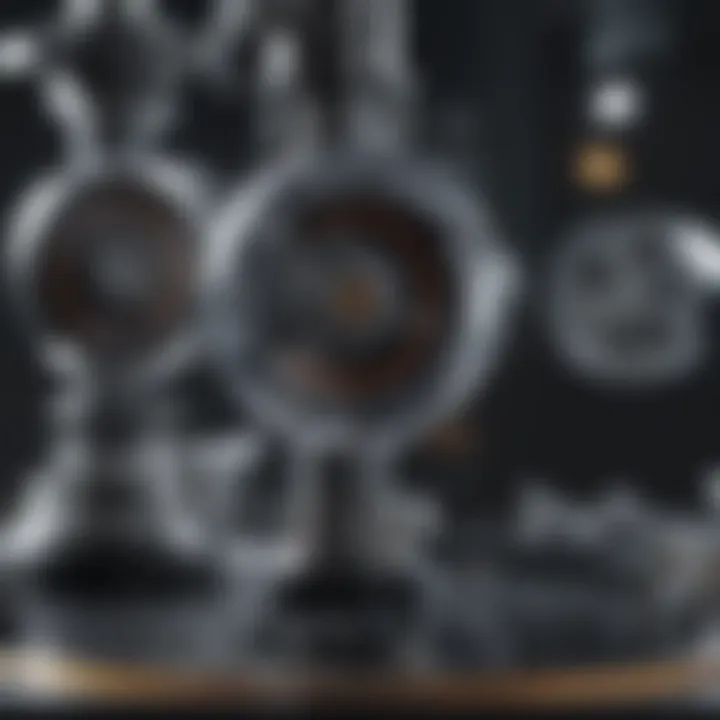
This section also helps to inform researchers and practitioners about the decision-making processes involved in choosing a cloning method for specific applications. With clarity on relative performance, efficiency, and flexibility, scientists can make more educated choices that ultimately enhance their work in genetic manipulation and synthetic biology.
Gibson Assembly vs. Traditional Cloning Methods
Traditional cloning methods often involve restriction enzymes and ligases. These methods require specific recognition sites on the DNA fragments, which necessitate prior planning. In contrast, Gibson Assembly does not rely on restriction sites. Instead, it utilizes overlapping regions of DNA, allowing for direct joining of fragments. This flexibility saves time and minimizes the need for precise site design.
Key differences include:
- Efficiency: Gibson Assembly typically yields higher transformation efficiencies compared to methods like restriction-ligation. This means more successful colonies can be derived from the assembled construct.
- Accuracy: The one-step assembly process reduces the risk of introducing errors, a common issue with traditional methods where multiple enzymes may create inconsistencies.
- Versatility: Gibson can seamlessly join multiple fragments in a single reaction, while traditional methods usually assemble one fragment at a time, complicating multi-gene constructs.
Understanding these differences is crucial for choosing the right approach for a given experimental design or desired outcome.
Gibson Assembly vs. Other Assembly Methods
There are various alternative methods for DNA assembly, such as Golden Gate Assembly and BioBrick Assembly, each with distinct features. Comparing them with Gibson Assembly reveals several important factors.
- Mechanism: Unlike Golden Gate Assembly, which relies heavily on restriction enzymes, Gibson Assembly’s mechanism is based on homologous recombination. This makes it less dependent on specific DNA sequences.
- Fragment Size: Gibson Assembly can accommodate longer DNA fragments compared to many other methods which can be restrictive regarding the size of the DNA pieces being joined. This capability is advantageous for constructing larger genetic constructs.
- Speed and Simplicity: Gibson Assembly has a straightforward protocol that typically takes less time than some other assembly methods which involve more complex procedures and multiple steps.
Research findings suggest that the choice of assembly method greatly depends on the desired outcome and specific project requirements. Individuals considering different assembly techniques must evaluate their unique strengths and weaknesses to optimize their work in biotechnology and molecular biology.
Choosing the appropriate assembly technique can lead to significant improvements in cloning efficiency and experimental outcomes.
Choosing the appropriate assembly technique can lead to significant improvements in cloning efficiency and experimental outcomes.
In summary, comparative analysis serves not only to illuminate the advantages of Gibson Assembly but also to acknowledge when other methods might be more appropriate.
Applications of Gibson Assembly
The applications of Gibson Assembly are vast and impactful in various domains of molecular biology. This method has revolutionized how scientists approach DNA manipulation, making it crucial in areas such as genetic engineering, synthetic biology, and genome editing. The efficiency and precision of this technique translate into significant advancements across multiple research fields.
Genetic Engineering
Gibson Assembly plays a vital role in genetic engineering, allowing for the seamless joining of multiple DNA fragments. This capability enables the construction of complex genetic constructs with minimal effort. With traditional methods, such as restriction enzyme cloning, the process can be cumbersome and less efficient. In contrast, Gibson Assembly simplifies these procedures by offering a one-step enzyme reaction.
The benefits of utilizing Gibson Assembly in genetic engineering include enhanced efficiency, higher accuracy, and the ability to create multiple modifications at once. Researchers often use this method to develop expression vectors or metabolic pathways, allowing for the optimization of gene function in various organisms. Moreover, the unique characteristic of overlapping ends in DNA fragments allows for the creation of custom genomic constructs, facilitating advancements in gene therapy and transgenic technology.
Synthetic Biology
In synthetic biology, Gibson Assembly stands out as an essential technique for designing and constructing new biological parts, devices, and systems. The precision it affords aligns with the goals of synthetic biology, which seeks to engineer biological components for novel applications.
Gibson Assembly enables the integration of synthetic circuits into living organisms. This is critical for developing microbial factories that produce valuable substances, such as biofuels or pharmaceuticals. The assembly of large synthetic genes can also lead to breakthroughs in biological research, increasing the efficiency of bioengineering projects.
Additionally, the versatility of this method allows for the rapid prototyping of genetic constructs. Scientists can iteratively modify and test various designs, accelerating the innovation cycle in synthetic biology. It provides a pathway to create tailored organisms with specialized functions, which can address some of the environmental and health challenges facing society today.
Genome Editing
Gibson Assembly is also used extensively in genome editing, complementing tools like CRISPR-Cas9. After targeted double-strand breaks are introduced in DNA by CRISPR, Gibson Assembly can facilitate the incorporation of new genetic material into the genome. This method ensures that the inserted sequences are seamlessly integrated without residual elements from traditional cloning methods.
In the context of genome editing, Gibson Assembly offers several advantages. First, the seamless nature of the assembly minimizes insertional mutagenesis risks, which could disrupt neighboring genes and lead to unintended consequences. Furthermore, it simplifies the integration of large DNA constructs, which is often challenging with other methods. Thus, researchers can explore new genetic configurations more effectively.
In summary, Gibson Assembly is pivotal in advancing numerous fields within molecular biology. Its applications in genetic engineering, synthetic biology, and genome editing facilitate the development of innovative solutions to pressing biological problems.
Limitations of Gibson Assembly
Understanding the limitations of Gibson Assembly is crucial for researchers and molecular biologists who wish to apply this technique effectively. While it is a groundbreaking method, several aspects can restrict its utility or affect its outcomes. Recognizing these limitations assists scientists in planning experiments better and in interpreting results with caution.
Fragment Length Limitation
One significant limitation relates to the fragment length that can be successfully assembled using Gibson Assembly. It is commonly suggested that fragments should ideally be between 500 and 2000 base pairs for effective assembly. Beyond this range, the efficiency often decreases. The longer the DNA fragments, the higher the chance of incomplete assembly, which leads to low yield of the desired product.
Researchers need to consider the complexity involved in longer fragments. Larger segments can introduce structural complications or secondary structures, such as hairpins, making assembly less predictable. For optimal results, scientists may need to design shorter overlapping regions that facilitate better assembly, or they may consider splitting larger fragments into smaller, manageable pieces to maintain assembly efficacy.
Context Dependence
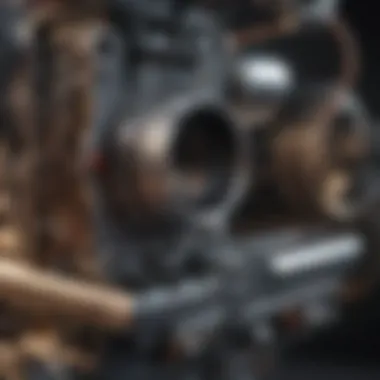
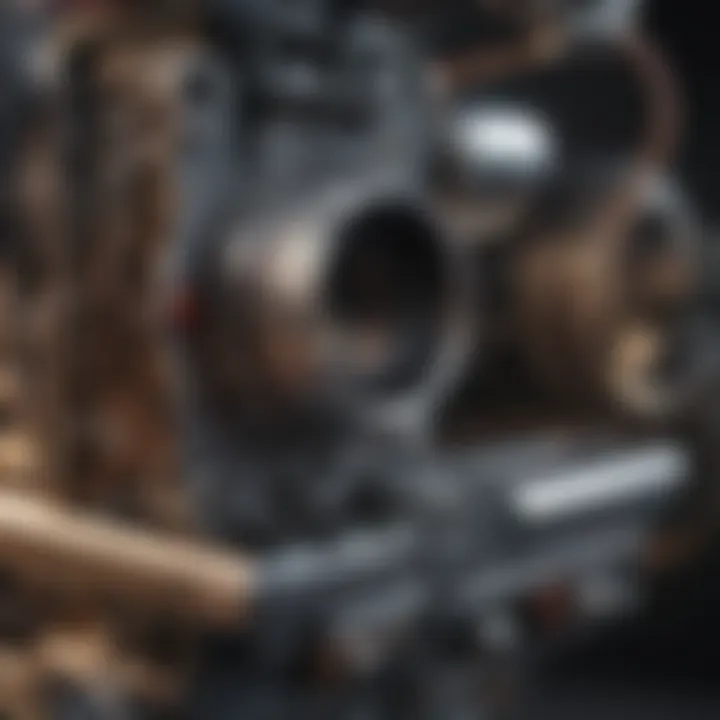
Another critical consideration is the context dependence of the Gibson Assembly technique. The success of assembling DNA fragments can vary based on multiple factors, including the sequence composition, purity, and the overall quality of the DNA segments. For example, the presence of specific nucleotide sequences may either enhance or hinder the efficiency of assembly due to their properties or the presence of secondary structures that interfere with the annealing of DNA.
"In molecular biology, a method's robustness is important, but context-sensitive performances can lead to unpredictable experiment outcomes."
"In molecular biology, a method's robustness is important, but context-sensitive performances can lead to unpredictable experiment outcomes."
The buffer conditions, temperature, and assembly time can also make a difference in whether the assembly is successful. These factors require researchers to often optimize each new assembly, which can be time-consuming and may lead to different results each time. It is vital to understand that achieving consistent results may demand extensive troubleshooting, especially when dealing with complex or unusual sequences.
Researchers and educators must be aware of these limitations, as they play an important role in the planning and execution of experiments involving Gibson Assembly. By actively addressing these concerns, one can mitigate the risks associated with unsuccessful assemblies and promote more reliable outcomes.
Recent Advances in Gibson Assembly
Recent advancements in Gibson Assembly have significantly enhanced its practical application in molecular biology. This section sheds light on key improvements and trends that shape the future of this technology. As the demand for precise and efficient genetic modifications grows, these innovations make Gibson Assembly an essential tool for scientists.
Optimizations and Modifications
Developments in Gibson Assembly methods focus on optimizing reaction conditions to increase efficiency and yield. Modifications in protocol now allow for the assembly of longer DNA fragments, thus overcoming the limitations seen in earlier techniques. Researchers have experimented with varying the concentrations of enzymes and DNA fragments, leading to quicker reaction times and higher success rates.
For instance, the use of engineered enzymes such as T5 exonuclease has improved the precision of overlap regions in DNA fragments. This precision reduces unwanted mutations, making the assembly process more reliable. Additionally, innovative buffer compositions are being tested to further enhance reaction conditions, enabling more robust assemblies even in complex systems.
These optimizations not only streamline the assembly process but also contribute to cost-effectiveness by reducing the need for expensive reagents.
Integration with Other Technologies
The integration of Gibson Assembly with other molecular techniques has opened new avenues for genetic research. For example, when coupled with CRISPR technology, Gibson Assembly allows for a rapid and efficient way to create large genomic constructs. This synergy enhances gene editing capabilities, facilitating advanced applications in therapeutic research and synthetic biology.
Moreover, the combination of Gibson Assembly with next-generation sequencing technologies has improved the verification process of assembled constructs. Using sequence-based approaches, researchers can quickly identify successful assemblies and ensure the fidelity of constructs before further experimentation.
Incorporating systems biology tools with Gibson Assembly also holds promise. By linking computational modeling with assembly practices, scientists can predict outcomes with greater accuracy, enhancing the development of genetic circuits.
"The intersection of Gibson Assembly with emerging technologies is driving precision in molecular biology, pushing the boundaries of what is possible with DNA assembly."
"The intersection of Gibson Assembly with emerging technologies is driving precision in molecular biology, pushing the boundaries of what is possible with DNA assembly."
In summary, recent advances in Gibson Assembly have made it a more powerful and versatile tool. Optimizations in the protocol and integration with other technologies not only improve efficiency but also elevate the overall scientific research landscape.
Future Directions
The realm of Gibson Assembly continues to evolve, driven by the need for greater efficiency and versatility in genetic research. Future directions in this field are critical, as they have the potential to enhance current methodologies and address existing limitations. Investigating these directions contributes not only to scientific understanding but also to practical applications in various biological fields.
Potential Innovations
Innovations in Gibson Assembly can take multiple forms. One promising area is the development of modified enzymes. These engineered enzymes could increase the efficiency and accuracy of the assembly process. Using variants that recognize diverse sequences may allow for broader application of Gibson Assembly across different organisms.
Another avenue of innovation involves optimizing the assembly protocol. Adjusting reaction conditions, such as temperature and concentration, could further improve yield. Additionally, implementing automation could streamline the process. This would facilitate larger-scale applications in synthetic biology, making Gibson Assembly accessible for high-throughput needs.
The integration of CRISPR technology presents another exciting potential innovation. Combining Gibson Assembly with CRISPR could revolutionize genome editing, allowing for more precise and efficient modifications. The synergy between these techniques could open new pathways in genetic research.
Broader Impacts on Molecular Biology
The advancements in Gibson Assembly have significant implications for molecular biology. As this technique matures, its adoption could lead to major breakthroughs in genetic engineering and synthetic biology. The ability to construct complex DNA sequences seamlessly enables researchers to build novel organisms or systems that were previously unimaginable.
Furthermore, as costs of synthetic biology decrease due to efficiency improvements, more labs may engage in bioengineering projects. This democratization of technology allows a wider array of researchers to contribute to the field. These developments can greatly enhance our understanding of genetic systems, which may lead to innovative applications in medicine, agriculture, and environmental science.
In summary, the future of Gibson Assembly holds the promise of transformative impacts across the biological sciences. Continued exploration of innovations and their subsequent applications will be vital for progressing the field and fostering a deeper understanding of molecular interactions.
End
Summary of Key Points
- Mechanism: The Gibson Assembly technique utilizes specific enzymes to join DNA fragments without the need for restriction enzymes, leading to seamless integration.
- Advantages: High efficiency and accuracy are paramount, allowing researchers to construct complex DNA sequences with minimal errors.
- Applications: Gibson Assembly has expanded the horizons of genetic engineering, particularly in synthetic biology and genome editing, facilitating groundbreaking research and advancements.
- Limitations: Despite its benefits, limitations such as fragment length and context dependence must be addressed to fully leverage its potential.
- Future Directions: Continued research into optimizations and integration with other technologies promises to enhance the breadth of Gibson Assembly's applications.
"The strength of Gibson Assembly lies in its ability to seamlessly join DNA segments, which is crucial for modern genetic manipulation."
"The strength of Gibson Assembly lies in its ability to seamlessly join DNA segments, which is crucial for modern genetic manipulation."
Final Thoughts
The insights provided herein demonstrate that Gibson Assembly represents a cornerstone in synthetic biology and genetic engineering. Emphasizing the balance between its effectiveness and limitations allows for a more realistic understanding of what these techniques can achieve. For students, researchers, educators, and professionals, mastering Gibson Assembly is essential not only for the advancement of individual projects but also for contributing to the broader scientific landscape. Staying informed about ongoing innovations in this field will ensure that one remains competitive and knowledgeable in molecular biology.
As we move forward, collaboration between disciplines and integration with emerging technologies may well shape the future of Gibson Assembly, enhancing its impact on molecular biology as a whole.