Comprehensive Guide to Boost Converter Schematics
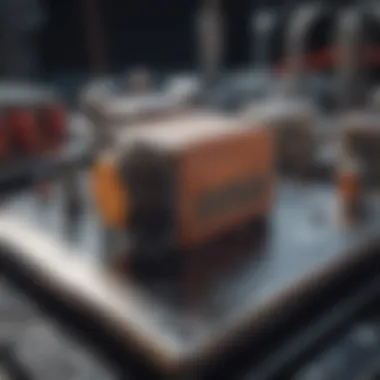
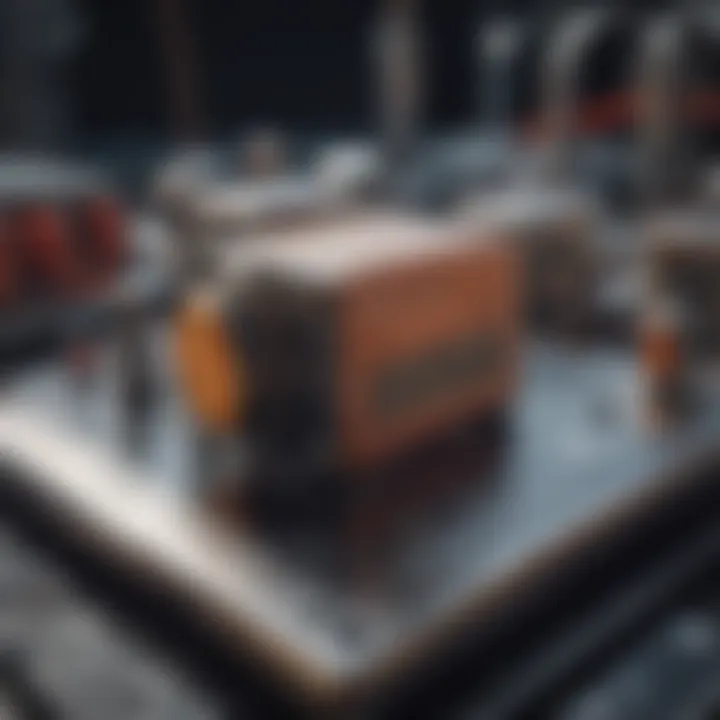
Intro
Boost converters play a pivotal role in various applications, from powering portable devices to handling renewable energy integrations. These circuits, though compact, can efficiently elevate voltage levels, which is crucial in many scenarios where power sources do not meet necessary voltage requirements directly. Understanding the schematics behind these converters is essential for engineers and enthusiasts alike.
At first glance, a boost converter may appear to be just another circuit, but as one digs deeper into its components and working principles, it becomes clear that there’s much to unpack. This exploration will offer insights not only into how these systems function but also into why they are so tremendously useful in power electronics.
As such, this article will layout the fundamental concepts, current trends in research, and the real-world applications of boost converters. By the end, you will appreciate the intricate design and operational dynamics of boost converters like never before.
Key Concepts
Definition of the Main Idea
A boost converter is a type of DC-DC converter that steps up voltage while simultaneously lowering current. At the heart of its operation is an inductive storage element, a switching element, and some form of energy regulation in the output. Essentially, when the switching element is activated, power gets stored in the inductor, and when it’s deactivated, the stored energy gets released at a higher voltage. This conversion mechanism is why it’s referred to as a boost converter.
Overview of Scientific Principles
The boost converter operates on the principles of electromagnetic induction and energy conservation. Key considerations include:
- Inductor Behavior: The inductor is critical in storing energy. During the switching cycle, when the switch is closed, current through the inductor builds up its magnetic field, storing energy.
- Switching Action: Fast switching impacts performance; the faster the switch can open and close, the more efficient the converter. Modern designs employ high-frequency transistors, like MOSFETs, to manage these cycles.
- Diode Functionality: During the off period of the switch, the diode allows the stored energy to flow to the load, ensuring voltage elevation.
These principles, when combined, allow boost converters to deliver efficiency rates often exceeding 90%, making them prime candidates for diverse applications.
"A boost converter is not just a component; it’s a gateway to innovative power solutions across numerous industries."
"A boost converter is not just a component; it’s a gateway to innovative power solutions across numerous industries."
Current Research Trends
Recent Studies and Findings
Recent advancements in the field indicate a strong focus on improving efficiency and reducing components. Research has shown that optimizing the control algorithms can lead to significant enhancements in performance. Studies have been conducted aiming to reduce the switching losses and improve thermal management, ultimately leading to longer-lasting devices.
Significant Breakthroughs in the Field
Notable breakthroughs can be seen in integrating smart technologies within boost converters. For instance, adaptive control strategies are finding their way into designs, allowing for real-time adjustments based on input variations or load demands. Such innovations pave the way for more resilient and adaptable power supply systems.
Prolusion to Boost Converters
Boost converters play a crucial role in the power electronics landscape, serving as indispensable components in various applications ranging from consumer electronics to renewable energy systems. At their core, boost converters are designed to elevate voltage levels, which can be any engineer's bread and butter when it comes to voltage regulation. Their importance in modern circuitry cannot be overstated, especially given the ever-increasing reliance on portable devices that require efficient power management.
Definition and Purpose
Simply put, a boost converter is a DC-DC converter that steps up the input voltage to a higher output voltage. Imagine a situation where you have a standard 5V input from a battery but need 12V to power a specific device. This is where a boost converter shines by manipulating the energy flow through its components—primarily an inductor, switch, diode, and capacitor—to achieve that voltage conversion. The main purpose is not merely elevating voltage but doing so while prioritizing efficiency and stability, ensuring that devices receive the necessary power without causing undue strain on the source.
One common analogy used in understanding boost converters is the idea of a water pump. In this analogy, the battery is the water source, and the boost converter acts like a pump that increases the water pressure (voltage) to reach another area (the load) that requires higher pressure to function effectively. Understanding this threshold is essential for anyone looking to design or integrate boost converters into their applications.
Historical Evolution of Boost Technology
The evolution of boost converter technology offers fascinating insights into how engineering advancements have shaped modern electronic devices. The concept dates back to the early 20th century when engineers began to explore the possibilities of converting electrical energy from one form to another. Early implementations were relatively inefficient, primarily focused on mechanical systems.
With the advent of semiconductor technology in the mid-20th century, the efficacy of power conversion processes skyrocketed. Early transistors acted as switches, allowing for better control over electrical circuits, thus paving the way for more refined boost converter designs. As the demand for compact and efficient power sources increased, researchers began refining these circuits, introducing improved inductors and capacitors that could handle higher frequencies and larger power switches.
"The development of power electronics from mechanical to solid-state devices sparked a revolution in how we manage electrical energy."
"The development of power electronics from mechanical to solid-state devices sparked a revolution in how we manage electrical energy."
The late 20th century saw an exponential rise in the use of DC-DC converters in consumer electronics, particularly due to advancements in integrated circuits. Devices such as smartphones and laptops rely on these converters to maintain battery longevity while providing sufficient power to various components. Today, with the growth of renewable energy technologies like solar power, boost converters are again becoming central to discussions on efficient energy utilization, enabling systems to store and use energy effectively. The trajectory of boost converter technology demonstrates how these components have evolved in complexity and performance, making them vital components for current and future electronic applications.
Fundamental Concepts
When discussing boost converters, understanding the fundamental concepts is crucial, as they form the backbone of how these devices function. The principles of voltage regulation and energy conversion provide the necessary framework for engineers and hobbyists alike to grasp the subtleties of this technology. Laying out these concepts not only aids in design but also plays a significant role in real-world applications that depend on efficiency and reliability.
Voltage Regulation
Voltage regulation in boost converters is about maintaining a steady output voltage despite variations in input voltage or load conditions. Simply put, if your device's input voltage fluctuates, the boost converter must adjust accordingly to ensure the output voltage remains stable. This is a vital task in many applications, ranging from everyday electronics to complex power systems.
- Feedback Loop: The primary mechanism for regulation involves a feedback loop. This loop continuously monitors the output voltage and compares it to a predefined standard. If it detects a drop, the control system adjusts the duty cycle of the switch to compensate. This makes sure the voltage doesn't stray too far from its target.
- Applications of Regulation: Think about mobile devices. They often operate at a fixed voltage. If the battery's charge drops, the boost converter needs to adapt and keep supplying the right voltage. So, engineers often implement sophisticated control algorithms to enhance stability and responsiveness.
"Voltage regulation is not just a technical aspect; it is a critical requirement for maintaining system integrity in power electronics."
"Voltage regulation is not just a technical aspect; it is a critical requirement for maintaining system integrity in power electronics."
- Challenges: Regulators must cope with complex scenarios such as variable loads. If a device suddenly demands more current, the converter should react swiftly to uphold performance. This challenge underlines the importance of vigilance in design and the role that precise components play in the overarching system.
Energy Conversion Principles
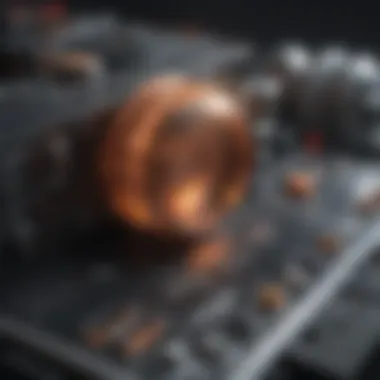
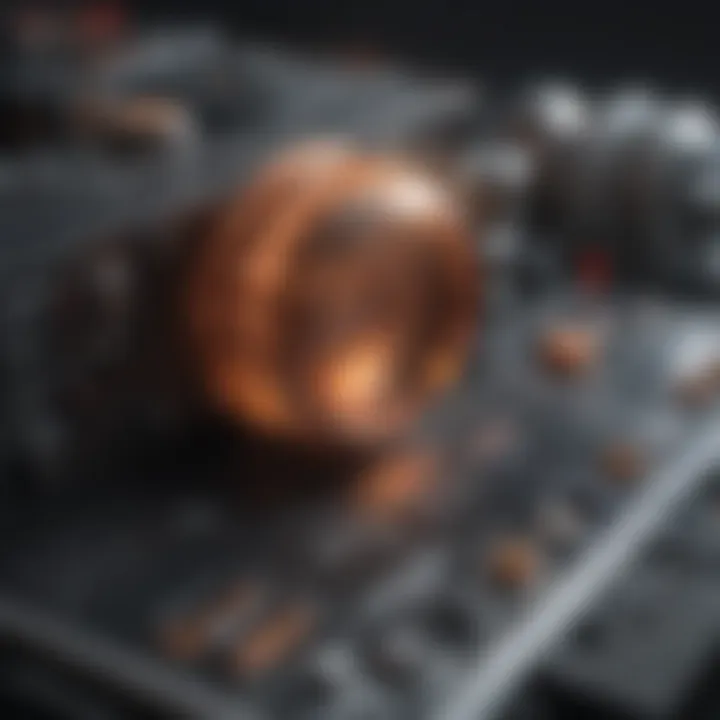
At the heart of every boost converter lies the need for efficient energy conversion. This process involves transforming energy from one form to another, specifically, increasing voltage while conserving power. This isn’t just about theoretical concepts; it has real implications in efficiency and sustainability.
- Basic Operation: When the switch in a boost converter is closed, energy is stored in the inductor. When the switch opens, this energy is released, increasing the output voltage. Though this might sound straightforward, achieving high efficiency in this process is where the complexities arise.
- Key Factors Influencing Efficiency: Factors influencing how well a boost converter performs include:
- Real-World Examples: Consider solar power applications. Here, the boost converter's energy conversion is essential for stepping up low-voltage outputs from solar panels to usable levels for batteries. Without this principle, converting renewable energy into usable electricity would be vastly inefficient.
- Inductor Quality: Poor inductor choices can lead to significant losses.
- Diode Forward Voltage Drop: This can sap efficiency if not accounted for.
- Switching Losses: The energy loss that occurs during switching periods must be minimized.
In summary, the fundamental concepts of voltage regulation and energy conversion are central to the design and application of boost converters. KNowing how these principles interact provides valuable insights that can spark innovation and optimization in various technological fields.
Key Components of a Boost Converter
The boost converter is a fundamental element in power electronics, serving a pivotal role in elevating voltage levels to meet the demands of a myriad of applications. Understanding the key components that make up a boost converter is crucial, as each part has its distinct function and influence on the overall performance of the system. This section will delve into the essential elements, the rationale behind their importance, and considerations for their integration.
Inductor: The Heart of the Converter
At the core of every boost converter lies the inductor, often considered the heart of the system. The inductor acts as a storage device for magnetic energy, allowing for the increase in voltage. When the switch is closed, current flows through the inductor, storing energy in its magnetic field. As the switch opens, this stored energy is released, boosting the voltage to a higher level and supplying it to the load.
This functionality is vital; an inefficient inductor can lead to significant losses, impacting the converter's overall efficacy. Factors to consider when selecting an inductor include:
- Inductance Value: Proper sizing is critical to ensure the desired current can be maintained without saturation.
- DC Resistance: Lower resistance means reduced losses, resulting in better performance.
- Current Rating: Must handle peak currents without overheating.
Switch: Control Mechanism
The switch is another major player in the boost converter's operation. When activated, it controls the current through the inductor, creating a direct link between the energy storage and voltage increase. The switch can be either a transistor or a MOSFET, with each type presenting its benefits in speed and efficiency.
Crucially, the speed at which the switch opens and closes reflects how effectively the converter can respond to load demands. High-frequency switching translates to smaller components, which can lead to a more compact design, while the thermal management of the switch is essential to avoid failures. The switch’s choice must align with:
- Switching Speed: Faster switches increase efficiency.
- Voltage Rating: Should be higher than peak voltage to withstand transients.
- Current Rating: Must handle load currents without risk of damage.
Diode: Directional Flow Regulator
The diode's role in a boost converter can be likened to a traffic cop, managing the flow of current. It ensures that the energy discharged from the inductor can only flow towards the output and prevents any backflow, which would otherwise discharge the inductor and defeat the purpose of voltage boosting.
Choosing the right diode involves understanding its characteristics. Key factors include:
- Forward Voltage Drop: A lower drop increases efficiency by reducing power losses.
- Reverse Recovery Time: Essential for high-frequency applications, determining how soon the diode can switch off.
- Peak Reverse Voltage: Must exceed the maximum output voltage to prevent breakdown.
Capacitor: Energy Storage
The capacitor in a boost converter plays a dual role: it smooths out the output voltage and acts as a reservoir for energy. When combined with the inductor, it helps maintain the desired output voltage under varying load conditions, ensuring stability in performance.
Factors to consider when selecting capacitors include:
- Capacitance Value: Larger capacitance helps in stabilizing voltage but increases size.
- Voltage Rating: Must withstand the maximum output voltage without risk of damage.
- Equivalent Series Resistance (ESR): Low ESR is preferred for efficiency, particularly in high-frequency applications.
"Understanding these components allows for better design choices and performance optimization in boost converter applications, crucial for anyone venturing into power electronics."
"Understanding these components allows for better design choices and performance optimization in boost converter applications, crucial for anyone venturing into power electronics."
With each of these components working harmoniously, they create a reliable boost converter capable of enhancing voltage levels effectively and efficiently. Whether in consumer electronics or renewable energy systems, knowledge of these components supports smarter applications and designs.
Schematic Representation
In the realm of boost converters, a schematic representation transcends mere illustration; it serves as a roadmap, guiding engineers through the intricate dance of electrical components. A well-crafted schematic not only demystifies the interconnections but also highlights the operational principles at play. Understanding these graphical manifestations is crucial in recognizing how each part contributes to voltage elevation. It’s an invaluable asset for students, researchers, educators, and professionals alike, facilitating better comprehension and effective troubleshooting.
Understanding a boost converter's schematic starts with appreciating its structural organization. Each symbol and line embodies a component and its functionality, directly relating to how the converter behaves under different loads or input conditions. Notably, the schematic allows for quick diagnostics, as any discrepancies from the expected design may reveal underlying issues.
Detailed schematics also ensure precision in the design phase. For those venturing into practical applications, seeing the flow of energy from one element to another enables engineers to assess performance metrics like efficiency and voltage output. Furthermore, insights drawn from schematics can aid in refining future designs, thus promoting continuous innovation.
"A schematic is not merely a depiction; it’s a blueprint of functionality, bridging the gap between theory and practice."
"A schematic is not merely a depiction; it’s a blueprint of functionality, bridging the gap between theory and practice."
By emphasizing clarity in schematic representation, professionals can improve communication among team members and clients. An easily understandable schematic means fewer missteps during the assembly and testing processes, ultimately resulting in effective solutions and optimized designs.
Reading a Boost Converter Schematic
Dissecting a boost converter schematic can be daunting for beginners, but with patience, one can unravel the layers of complexity. Each component carries its weight in the grand scheme. At first glance, you are greeted by the inductor, taking center stage. Its role in storing energy is critical to the voltage increasing function. Alongside, the switch toggles between open and closed states, creating an environment for energy transfer.
Next, the diode ensures directional current flow, preventing backflow which could undermine the divergent voltage levels. The capacitor, keeping everything balanced, stores the energy before it's released to the output. Understanding how these parts interlink gives a clear picture of voltage transformation.
Moreover, recognizing the input and output terminals is essential. The input side usually represents a lower voltage, which the boost converter elevates to a desired output level. Labeling these connections diligently is often seen in well-drafted schematics, making it easier to follow the flow of current.
Thus, by taking time to scrutinize the schematic and associating the electrical symbols with their physical counterparts, one can gain significant insight into the workings of the boost converter.
Common Symbols Used
When diving into circuitry design, familiarity with common symbols is as crucial as understanding the components themselves. This aids not only in reading schematics but also in communicating ideas effectively. Below are several symbols frequently encountered in boost converter schematics:
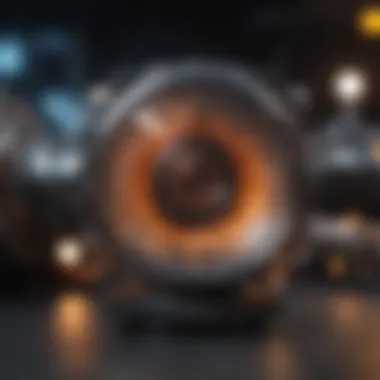
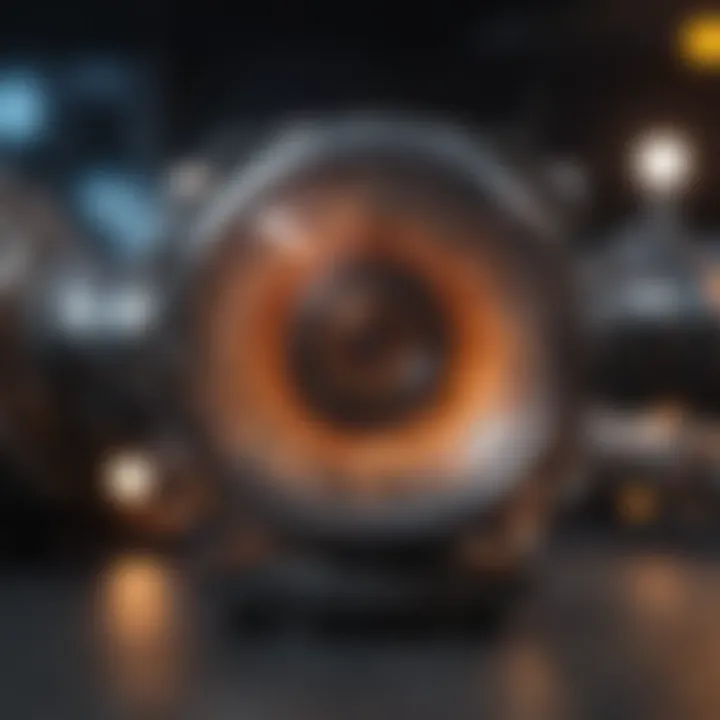
- Inductor (L): Often represented by a series of loops or squiggles, indicating its role in energy storage.
- Switch (S): Typically illustrated as a break in the line, a symbol indicating either a closed or open position.
- Diode (D): Marked by an arrow, it symbolizes the direction of current flow, with the triangle indicating the anode and the line the cathode.
- Capacitor (C): Shown as two parallel lines that can either be vertical or horizontal, storing and releasing energy with ease.
- Voltage Source (Vin/Vout): Denoted usually by a circle with a plus and minus sign, indicating positive and negative terminals.
By understanding these symbols, one can traverse a schematic with confidence, interpreting not only their meaning but also their significance in the converter's operation. This foundational knowledge enables engineers to discuss designs and troubleshoot issues efficiently, ensuring better outcomes in project developments.
Operation of Boost Converters
The operation of boost converters is a critical aspect of understanding their function within power electronics. Unlike their other counterparts that may simply convert energy from one form to another, boost converters effectively elevate voltage, transforming lower voltage to higher, which is often essential in various applications. The ability to step up voltage arises from the clever marriage of inductive storage and switch-controlled release, providing benefits that range from energy efficiency to practical implementations in everyday devices.
In this section, we will delve deeper into the mechanics behind boost converters, highlighting specific features and considerations that underpin their operation. Grasping these concepts is essential for anyone venturing into the world of power electronics, particularly if you’re looking to optimize performance in real-world situations.
Step-Up Voltage Action
At the heart of a boost converter is its fundamental purpose: to perform the step-up voltage action. This essence can be boiled down to a straightforward principle: energy conservation. When you apply a voltage to the inductor, it stores energy in the magnetic field. The current flows through the inductor, gaining energy until the switch opens, resulting in the inductor discharging and thus increasing the voltage.
Key Elements of Step-Up Voltage Action:
- Energy Storage: The inductor charged during the 'on' phase, effectively bottling up energy.
- Switch Mechanism: This component enables and disables the electrical connection to manage energy flow efficiently.
- Voltage Doubling: When the stored energy is released, the process can yield a voltage often greater than the input, influenced by the duty cycle of the switch.
This action can be represented mathematically as:
where V_out is the output voltage, V_in is the input voltage, and D is the duty cycle. Such relationships exemplify how a boost converter can significantly enhance the voltage available to connected loads.
Continuous vs. Discontinuous Mode
Understanding the operational modes of boost converters—specifically continuous and discontinuous modes—provides further insight into their performance. These modes define how the inductor behaves during operation, which in turn affects efficiency and design considerations.
- Continuous Mode: In this mode, the inductor current never fully discharges to zero before the switch closes again. This sustained current flow leads to improved efficiency as there is minimal disruption in energy transfer. Continuity in current helps manage smooth voltage output, making this mode favorable for applications demanding consistency, like battery charging systems.
- Discontinuous Mode: Conversely, in this mode, the inductor current can reach zero during part of the switching cycle. It typically occurs at low loads where energy demand is reduced. This mode can be less efficient in certain scenarios as it results in a higher peak current during operation. Discontinuous mode might introduce ripple in voltage, which could become a concern for sensitive applications.
In summary, choosing between continuous and discontinuous mode has repercussions for efficiency, performance, and application suitability. Selecting the right mode is pivotal for optimizing the overall operation of boost converters.
In summary, choosing between continuous and discontinuous mode has repercussions for efficiency, performance, and application suitability. Selecting the right mode is pivotal for optimizing the overall operation of boost converters.
By comprehending these two operational modes along with the underlying principles at play, one can appreciate the flexibility and utility of boost converters in multiple domains.
Design Considerations
When it comes to implementing boost converters, design considerations weigh heavily. The significance of this topic cannot be overstated. Proper design not only ensures optimal functionality but also safeguards against potential issues that can arise during operation. Key elements such as selecting component values and optimizing efficiency must be thoroughly understood and meticulously addressed.
Selecting Component Values
Selecting the right component values is crucial for the performance of a boost converter. Each component plays a unique role, and getting the parameters correct is like finding the right pieces for a puzzle. If one piece is mismatched, the entire picture suffers.
- Inductor: The inductance value directly influences the energy storage capability and ultimately affects the boost factor. A higher inductance allows for more energy storage during the switching cycle, which can improve performance.
- Capacitor: The output capacitor helps smooth the output voltage. It’s essential to select one with a voltage rating higher than the expected output, plus an appropriate capacitance to reduce ripple effectively.
- Diode: The rectifying diode must have a low forward voltage drop to increase overall efficiency. Its recovery time is critical too, especially in high-frequency applications.
- Switch: The switch must be rated for currents greater than the expected load. Also, factors like on-resistance and switching speed should be considered for optimal performance.
In addition to individual components, tolerances must be factored into the equation. The real-world performance may vary based on temperature, aging, and other environmental factors. Thus understanding the effects of these tolerances on performance is essential for designing reliable systems.
Efficiency Optimization Techniques
Boost converters are typically heralded for their ability to increase voltage. However, the efficiency of this operation can vary widely. Optimizing efficiency isn’t just a technical challenge; it’s a critical design consideration that directly impacts the device’s overall performance and viability for various applications.
Several techniques can boost efficiency:
- Minimize Copper Losses: Using wider traces or thicker wire for the inductor can significantly reduce resistive losses.
- Selecting High-Efficiency Diodes: Schottky diodes are often favored for their low forward voltage drop and fast switching speeds, making them ideal for high-frequency applications.
- Use Integrated Circuits: Many manufacturers offer ICs optimized for specific applications. These often include built-in components that boost overall efficiency.
- PWM Control Methods: Using pulse width modulation can help in efficiently managing energy transfer, reducing losses during switching.
- Thermal Management: Overheating leads to inefficiency and failure. Implementing heat sinks or fans can significantly increase lifespan and efficiency by maintaining optimal temperature levels.
"In the world of electronics, efficiency isn’t just desirable; it’s mission critical. One small misstep can lead to cascading failures that compromise entire systems."
"In the world of electronics, efficiency isn’t just desirable; it’s mission critical. One small misstep can lead to cascading failures that compromise entire systems."
In practice, considering both selecting appropriate component values and implementing efficiency optimization techniques can dramatically elevate the performance of a boost converter. For professionals, educators, and students alike, these design considerations will serve as the backbone for robust and reliable power management solutions.
By weaving these elements together thoughtfully, designers can push the boundaries of what boost converters can achieve, making them indispensable tools in modern technology deployment.
Applications of Boost Converters
Boost converters play a significant role in modern technology applications. Their ability to increase voltage efficiently makes them fundamental in various sectors. In this section, we will explore their importance in three pivotal areas: consumer electronics, renewable energy systems, and electric vehicles. Understanding these applications helps highlight the versatility and necessity of boost converters in our daily lives and industry innovations.
Consumer Electronics
In the realm of consumer electronics, boost converters are crucial for powering devices that require higher voltage levels from lower voltage sources. For instance, mobile phones and laptops often utilize lithium-ion batteries that typically deliver 3.7 volts. However, many components within these devices need higher voltages to function effectively.
Boost converters come to the rescue by stepping up the voltage to meet these demands. This not only sustains device performance but also helps in extending battery life by optimizing energy use. The compact size of these converters also allows manufacturers to integrate them seamlessly into small devices. As a result, consumers enjoy sleek designs without compromising on functionality.
"Harnessing optimal voltage levels ensures that consumer electronics deliver peak performance and user satisfaction."
"Harnessing optimal voltage levels ensures that consumer electronics deliver peak performance and user satisfaction."
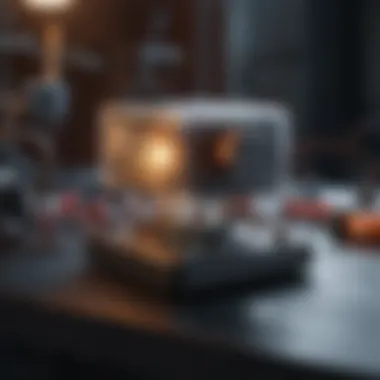
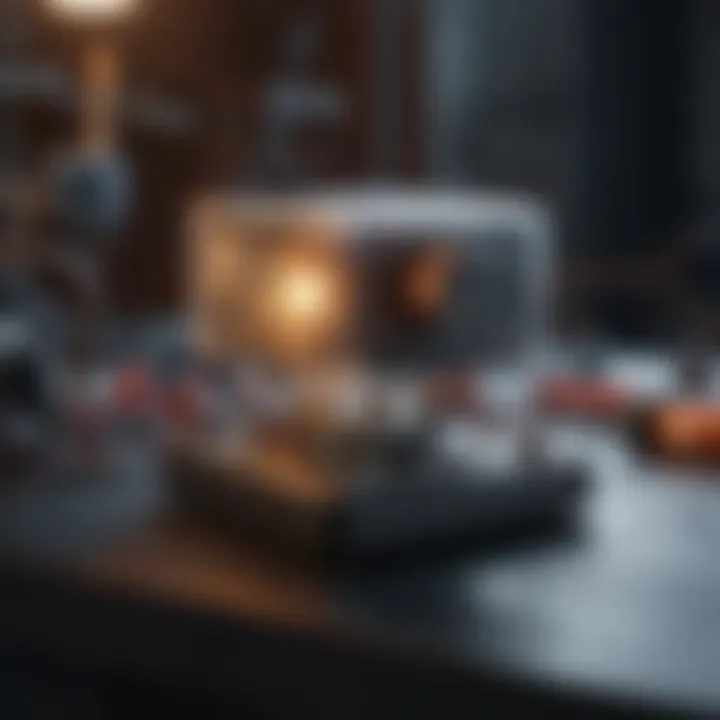
Key Benefits:
- Enhanced performance in portable devices
- Efficient power management leading to longer battery life
- Integration of compact design facilitating modern aesthetics
Renewable Energy Systems
Renewable energy systems, particularly solar and wind energy setups, benefit substantially from boost converters. They serve to convert the variable voltage output from solar panels or wind turbines into a higher, stable voltage that is necessary for grid connectivity or supporting electrical loads. This increases the overall efficiency of energy harvesting and utilization.
For example, a typical solar panel generates an output that fluctuates throughout the day due to changes in sunlight intensity. The boost converter stabilizes this variability by stepping up the voltage to a consistent level, ensuring that the energy harnessed is effectively used or stored. Moreover, as these systems evolve, innovative designs for boost converters are being developed, such as those tailored for maximum power point tracking (MPPT).
Considerations:
- Adaptability to varying input voltages
- Enhanced efficiency for energy storage solutions
- Supporting reduced carbon footprint by facilitating renewable sources
Electric Vehicles
Electric vehicles (EVs) represent one of the most significant advancements in the automotive industry. Boost converters are pivotal in managing power distribution inside EVs. They ensure that the battery’s low voltage can be transformed into the higher voltage required to drive the electric motor efficiently. This increases the overall drive range and performance.
Moreover, boost converters also play a role in regenerative braking systems, where kinetic energy is converted back into electrical energy to recharge the battery. This capability not only improves energy efficiency but also enhances the vehicle's overall performance.
Key Aspects:
- Voltage regulation for optimal motor performance
- Energy recuperation during braking, enhancing battery life
- Contribution to sustainable transportation initiatives
The diversified applications of boost converters underline their critical role in advancing technology across various fields. As we continue to innovate, understanding these applications helps us appreciate the underlying mechanisms that support modern efficiency in both consumer and industrial domains.
Troubleshooting Common Issues
Troubleshooting issues in boost converter systems is crucial, especially for engineers and technicians who rely on these components for stable power management. Identifying problems before they escalate can save time and resources. In this section, we will explore common issues, focusing on voltage drops and component failures.
Identifying Voltage Drop Issues
Voltage drop can be a silent killer in a boost converter. It occurs when the output voltage fails to reach the expected level due to various reasons such as resistive losses, poor connections, or even component inefficiencies. The result can be underwhelming voltage performance that plays havoc with the reliability of the entire system.
When addressing this issue, start by examining connections. Ensure that all wiring is secure and that solder joints are tight. Poor connections can create resistance that leads to unwanted voltage drop. Additionally, measuring the voltage at various points in the circuit can pinpoint where the drop is occuring.
Here are a few tips to assist in identifying voltage drop issues:
- Check Component Ratings: Ensure all components can handle the voltage and current levels.
- Inspect PCB Layout: A congested layout can cause unexpected resistive paths.
- Use Quality Cables: Low gauge cables contribute to voltage loss.
Once you have identified where the drop happens, comparing the readings with expected values can often highlight if other components are to blame.
"Look beyond the obvious, sometimes the smallest details hide the hugest problems."
"Look beyond the obvious, sometimes the smallest details hide the hugest problems."
Component Failure Diagnosis
Component failures in boost converters can lead to erratic performance or complete system shutdown. Understanding common signs of component failures saves time and effort during the troubleshooting process. Each key component in the boost converter plays a role, and if one part misbehaves, it can affect the whole system.
To get to the root of component issues, a systematic approach is key. You may want to start with the switch, which is often the first line of defense in regulating input power. A malfunctioning switch might show as excessive heat or an audible clicking sound.
Here’s how to diagnose component failures effectively:
- Check Inductors: Measure the inductance with a multimeter to ensure they don’t have short circuits.
- Inspect Diodes: When a diode fails, it tends to conduct in both directions, which can confuse your testing. Test it both ways with a multimeter.
- Capacitor Examination: Look for bulging or leaking capacitors. Capacitance measurement can also verify if they are functioning correctly.
Performing these simple inspections can dramatically simplify the troubleshooting process and make your boost converter as reliable as a ticking clock.
Future Trends in Boost Converter Technology
As we steere ourselves into an era where energy efficiency and smart technology take the front seat, understanding the future trends in boost converter technology becomes paramount. This section focuses on two fundamental aspects: the integration of these converters with smart technologies and advancements in materials aimed at improving efficiency. Recognizing these trends not only sheds light on the evolution of boost converters but also highlights the significant benefits they bring to various sectors.
Integration with Smart Technologies
The rise of the Internet of Things (IoT) and smart devices is reshaping our daily lives and, consequently, the design and application of boost converters. Smart technologies often require power solutions that are both flexible and efficient. A boost converter's capability to step-up voltage makes it ideal for powering smaller, low-voltage devices, which is a primary need for many IoT applications.
Incorporating artificial intelligence and data analytics into power management systems is another compelling trend. With smart converters, monitoring energy consumption and adjusting performance in real-time can drastically reduce energy waste. For instance, in a smart home setup, a boost converter coupled with machine learning algorithms can optimize power delivery based on the user's habits, thus enhancing battery life and overall performance.
Moreover, embedding connectivity solutions like Bluetooth or WiFi within boost converter circuits allows for remote monitoring and control, giving users the ability to manage energy use from their devices. This leads to not just convenience but also potentially significant cost savings on energy bills.
"The integration of boost converters with smart technology is not merely a trend; it’s a leap towards a more interconnected and efficient energy ecosystem."
"The integration of boost converters with smart technology is not merely a trend; it’s a leap towards a more interconnected and efficient energy ecosystem."
Advancements in Materials and Efficiency
Moving beyond the realm of circuitry, advancements in materials used in boost converters promise to enhance their efficiency and reliability. Traditionally, silicon has dominated the landscape; however, new materials like gallium nitride (GaN) and silicon carbide (SiC) are on the rise. These materials offer higher thermal conductivity and greater efficiency at elevated temperatures, allowing for more compact and powerful converters.
Switching losses are a major efficiency concern in boost converter designs. By utilizing GaN or SiC, engineers can achieve faster switching speeds with significantly lower losses, which not only improves overall performance but also reduces the heat generated during operation. This can lead to longer lifespans for the devices powered by these converters due to lower thermal stress.
Another consideration is the development of multilayer ceramic capacitors (MLCCs). These capacitors have become increasingly popular due to their ability to support higher performance while minimizing size. With the push towards miniaturization in consumer electronics, the integration of MLCCs into boost converters aligns perfectly with design requirements.
In summary, as the landscape of energy technology continues to evolve, boost converters are set to become more efficient, reliable, and versatile. The trends towards smart integration and novel materials are only just the tip of the iceberg; the future holds vast possibilities for enhancing energy systems worldwide.