Heated Soil: Impacts on Agriculture and Climate
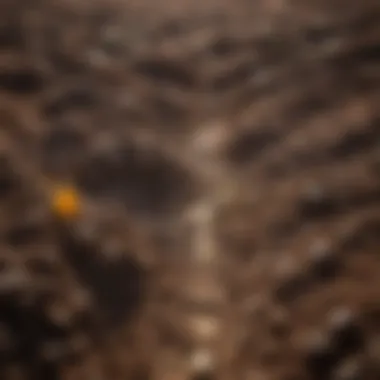
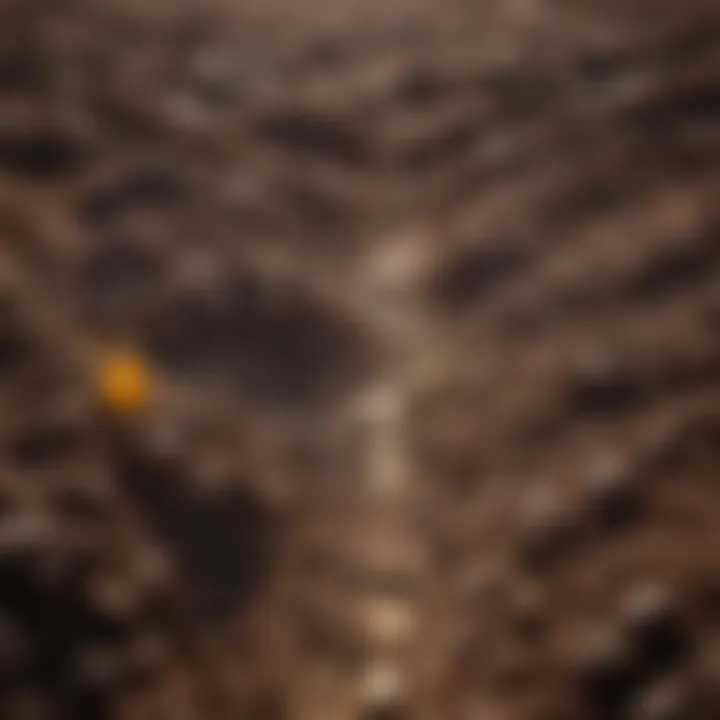
Intro
Heated soil presents a complex challenge that deeply intertwines with agriculture, ecology, and climate science. Understanding the dynamics of soil temperature is crucial for various stakeholders including farmers, researchers, and policy makers. Numerous factors influence soil temperature, ranging from climate variability to human activities. Consequently, the implications of heated soil extend beyond immediate agricultural impacts to encompass broader ecological and climatic systems.
Soil serves as a foundation for agricultural productivity, playing a critical role in plant health and development. When soil temperature elevates, it alters the microbial communities that support nutrient cycling and can lead to shifts in plant species composition. This article delves into these intricate relationships, examining how changes in soil temperature can disrupt established ecological balances and impact land management strategies. By synthesizing current research findings alongside real-world case studies, the aim is to illuminate the essential insights into how heated soil affects, and is affected by, the surrounding environment.
Preamble to Heated Soil
The phenomenon of heated soil warrants significant attention in today's discourse surrounding agriculture, ecology, and climate change. At its core, heated soil refers to soil temperatures that exceed normal levels due to various natural and anthropogenic factors. This section provides crucial insights into the complexities and implications of heated soil for sustained agricultural productivity, ecological balance, and climate stability.
Defining Heated Soil
Heated soil can be described as soil that experiences temperatures significantly above its typical range, predominantly due to solar radiation, altered land use, and climate change. Factors such as reduced vegetation cover, urbanization, and inappropriate agricultural practices exacerbate this heating. Understanding the specific temperature thresholds that characterize heated soil is key, as these thresholds influence various processes, including nutrient cycling, moisture retention, and biological activity.
Importance in Ecological and Agricultural Contexts
Heated soil holds extensive importance in both ecological and agricultural contexts for several reasons. First, it directly impacts the growth and development of plants. Elevated temperatures can disrupt physiological processes, leading to increased stress among crops. As crops struggle to adapt, yields may decline, threatening food security, particularly in regions heavily reliant on agriculture.
Second, soil heating affects microbial communities, which are vital for nutrient cycling and soil health. Changes in microbial activity can lead to altered decomposition rates and nutrient availability, further stressing plant systems. These shifts can initiate a feedback loop, wherein plant health declines, leading to more heated soil conditions.
Third, in the context of climate change, heated soil contributes to the emission of greenhouse gases, especially carbon dioxide and methane. This phenomenon not only exacerbates global warming but also disrupts local ecosystems by altering habitat suitability for various organisms.
In summary, understanding heated soil's implications is essential for formulating effective environmental policies and agricultural practices that can mitigate adverse outcomes. Such knowledge equips stakeholders—ranging from farmers and agronomists to ecologists and climate scientists—with the insights needed to adapt to and potentially counteract these challenges.
Mechanisms of Soil Heating
Understanding the mechanisms of soil heating is crucial for grasping how it impacts agriculture, ecology, and climate. Soil temperature is not a static measure; it fluctuates due to various natural and human-induced processes. Each mechanism plays a significant role in shaping the soil environment, affecting plant growth and microbial activity, and influencing overall ecosystem dynamics. The interrelation of these mechanisms can help researchers and practitioners develop better agricultural strategies and environmental management tools.
Natural Processes Affecting Soil Temperature
Natural processes are among the primary factors influencing soil heating. These include solar radiation, atmospheric temperature, and moisture content in the soil. The sun provides the most direct heat source, warming the top layers of soil during the day. This heating can change based on several phenomena:
- Seasonal Variations: Different seasons bring varying lengths of daylight and intensity of sunlight, which can significantly affect soil temperatures. For example, warmer months tend to lead to higher soil temperatures.
- Soil Moisture: Water has a high specific heat capacity, meaning it can absorb and store a lot of heat without a corresponding rise in temperature. Soils with high moisture generally heat up more slowly, while drier soils may experience more extreme temperature fluctuations.
- Topography: The landscape can also influence heating. For instance, slopes may warm faster than flat areas due to more direct sunlight exposure. Similarly, depressions might trap cooler air, leading to lower soil temperatures.
These natural processes highlight the complexity of soil heating and how various factors interact. Understanding these can help farmers and ecologists optimize land use and conservation efforts.
Anthropogenic Influences on Soil Heating
With growing human activities, the landscape of soil heating has undergone significant changes. Anthropogenic influences alter natural conditions, leading to increased soil temperatures and resultant effects on agriculture and the environment. The main areas of concern include:
- Urbanization: The expansion of cities leads to the creation of heat islands. These areas tend to have elevated temperatures due to heat-absorbing materials, such as asphalt and concrete, as well as reduced vegetation.
- Land Use Changes: Agricultural practices can dramatically alter soil temperature. Tilling and deforestation expose soil to sunlight and can reduce shade provided by plants. This can lead to increased evaporation and thus higher soil temperatures.
- Climate Change: The broader implications of climate change cannot be overstated. Rising global temperatures contribute to alterations in soil heating patterns, affecting moisture levels and thereby changing nutrient availability within the soil. Increased carbon dioxide concentration can also lead to changes in soil organic matter dynamics.
"The combination of natural and anthropogenic processes creates a complex framework affecting soil heating, ultimately linking back to agricultural and ecological outcomes."
"The combination of natural and anthropogenic processes creates a complex framework affecting soil heating, ultimately linking back to agricultural and ecological outcomes."
Overall, understanding these anthropogenic influences provides essential insight into developing practices that mitigate adverse effects on soil temperature. Awareness of both natural and human-induced mechanisms is foundational for addressing the implications of heated soil effectively.
Effects of Heated Soil on Plant Growth
Understanding the effects of heated soil on plant growth is crucial for several reasons. First, it directly influences crop yield and agricultural sustainability. As soil temperature rises, various physiological processes in plants can be affected. This includes aspects such as seed germination, root development, and overall growth rates. Furthermore, variations in soil temperature can lead to changes in water availability and nutrient dynamics, which are essential for plant health.
Thermal Stress and its Impact
Thermal stress occurs when plants are exposed to soil temperatures outside their optimal growing range. This stress can impede physiological functions, resulting in stunted growth, reduced productivity, and lower resistance to pests and diseases. Studies have shown that elevated soil temperatures can cause altered enzyme activity in plants, which subsequently affects metabolic pathways essential for growth.

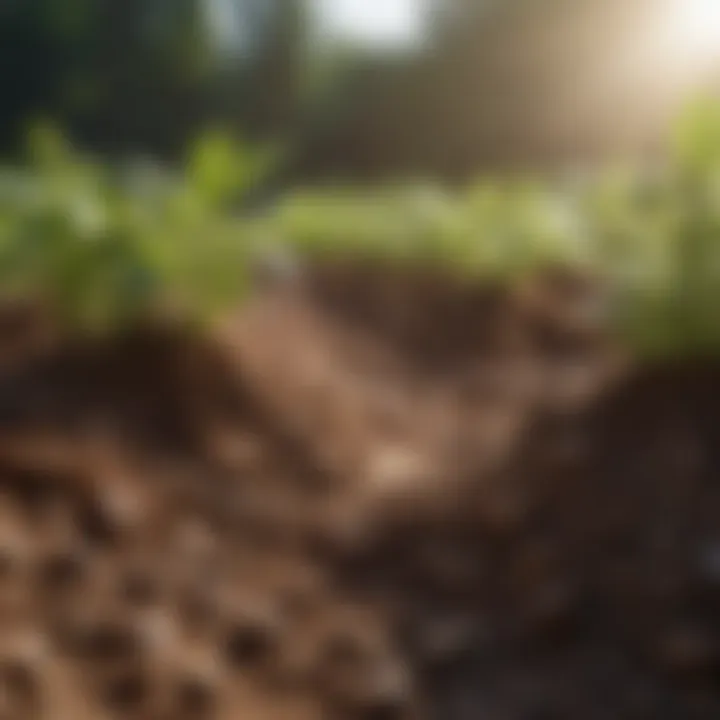
Some important points to consider:
- Germination Rates: Higher soil temperatures can accelerate seed germination, but excessive heat may inhibit it entirely, causing failure to sprout.
- Root Structure: Elevated soil temperatures can lead to compromised root structures. Roots may become dysfunctional, limiting their ability to uptake water and nutrients.
- Moisture Levels: Warmer soils can increase evaporation rates, leaving plants in a state of stress due to water deficiency.
The effects of thermal stress are particularly significant during critical growth stages. Early stages, like germination and seedling development, are especially sensitive. If temperatures exceed a certain threshold, it can lead not only to crop failures but also long-term soil degradation.
Nutrient Availability and Soil Thermal Dynamics
Nutrient availability is closely linked to soil thermal dynamics. Higher temperatures can alter the chemical processes that deliver nutrients to plants. For instance, nitrogen, phosphorus, and potassium availability can fluctuate due to changes in microbial activities that govern nutrient cycling.
Key factors affecting nutrient dynamics include:
- Microbial Activity: Warmer soils can enhance microbial metabolism, leading to increased nutrient mineralization. However, if temperatures are excessively high, microbial populations may decline, resulting in fewer nutrients being released into the soil.
- Soil Moisture: As previously mentioned, heated soil can reduce moisture levels, affecting nutrient solubility and transportation within the soil matrix. Low moisture levels limit the plants' ability to absorb essential nutrients effectively.
- Soil pH: Soil heating can affect its pH, which in turn influences nutrient availability. For example, an increase in temperature can cause some essential nutrients to become less available to plants, leading to deficiencies.
The interplay between temperature and nutrient availability directly impacts plant resilience and productivity. Understanding these mechanisms allows for more informed agricultural practices and can help tailor management strategies to mitigate adverse effects from heated soil.
Understanding soil temperature variations not only aids in improving crop resilience but also enhances overall soil management and sustainability practices.
Understanding soil temperature variations not only aids in improving crop resilience but also enhances overall soil management and sustainability practices.
Microbial Interactions in Heated Soil
Microbial interactions within heated soil are critical for understanding the broader implications on agriculture, ecology, and climate change. The increased soil temperature can significantly influence not just plant growth but also the microbial communities that facilitate nutrient cycling and soil health. When soil heats up, it shifts the dynamics of these microbial populations, often leading to changes that can have profound consequences across ecosystems. Recognizing these changes allows for better management practices and adaptations to climate challenges.
Shifts in Microbial Community Structure
Heated soil affects the composition of microbial communities. Warmer temperatures can favor certain microbial taxa while suppressing others. For example, thermophilic bacteria, which thrive at higher temperatures, may become more prevalent. This shift can alter nutrient pathways, impacting how carbon, nitrogen, and phosphorus cycles through the ecosystem.
The restructuring of microbial communities may disrupt symbiotic relationships essential for plant growth, such as those between plants and mycorrhizal fungi.
The restructuring of microbial communities may disrupt symbiotic relationships essential for plant growth, such as those between plants and mycorrhizal fungi.
Additionally, the decline of sensitive species can lead to a reduced diversity within the microbial community. A loss in diversity may hamper the ecosystem's ability to adapt to environmental changes. Factors such as moisture availability, organic matter decomposition, and soil texture could further influence these shifts, complicating land management efforts.
Functional Changes in Microbial Activity
As soil temperature rises, the activity levels of various microorganisms change. Warmer soils generally accelerate metabolic processes, leading to faster decomposition rates. This process increases the release of nutrients. However, it can also lead to increased carbon loss through respiration. The balance between nutrient availability and losses becomes delicate.
The efficiency of nutrient uptake by plants can be impacted by these functional changes. Beneficial microorganisms that foster nutrient absorption might be outcompeted by less advantageous species under higher temperatures. Furthermore, microorganisms crucial for nitrogen fixation could become inhibited, leading to lower soil fertility.
In summary, understanding microbial interactions in heated soils is essential for predicting future agricultural outcomes and ecological impacts. The implications of these shifts underscore the need for strategic soil management approaches that consider not only the immediate effects of heat but also the long-term health and productivity of our soils.
Soil Heating and Carbon Dynamics
In examining the implications of heated soil, the relationship between soil heating and carbon dynamics emerges as a critical area of focus. As soil temperature increases, its capacity to store and regulate carbon changes significantly. This is important for both agricultural productivity and the broader ecological balance. Additionally, understanding how soil heating affects carbon cycling can inform land management practices, aiming for sustainability in an era of climate change.
Impact on Soil Organic Carbon
Soil organic carbon (SOC) is a key component of soil health. It plays a crucial role in nutrient availability and soil structure. When soil temperatures rise, several processes come into play that affect SOC levels. Higher temperatures can enhance microbial activity, accelerating the decomposition of organic matter. This process increases the release of carbon dioxide into the atmosphere, contributing to greenhouse gas emissions and potentially exacerbating climate change.
To evaluate the effects of heated soil on SOC, consider the following key points:
- Decomposition Rates: Elevated temperatures may lead to faster decomposition rates of organic material. With more organic matter breaking down, the stability of SOC is compromised.
- Storage Capacity: Warmer soils are less effective at storing carbon over the long term. This can shift the soil from a carbon sink to a carbon source, impacting global carbon budgets.
- Nutrient Cycle Disruption: The rapid breakdown of organic matter may also lead to nutrient imbalances. This can ultimately affect plant growth, as essential nutrients may become less available.
Feedback Loops with Climate Change
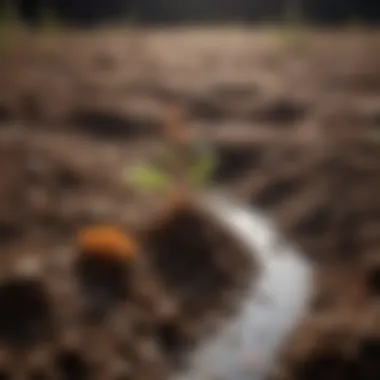
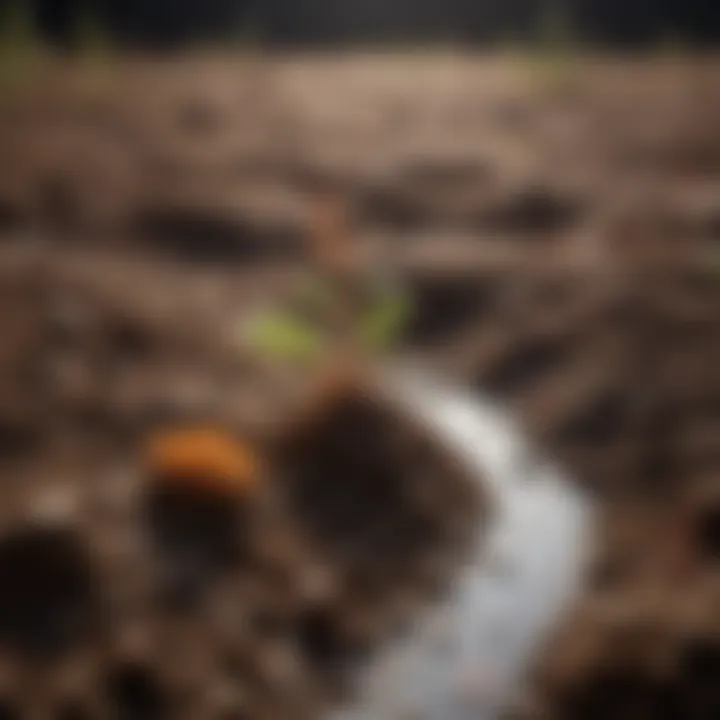
The interaction between heated soil and carbon dynamics creates important feedback loops that affect climate change. As climate change progresses, it leads to increased temperatures that further affect soil conditions. Conversely, changes in soil carbon dynamics can exacerbate climate change effects. This back-and-forth relationship is critical to understanding the larger ecological picture.
Consider these aspects of feedback loops:
- Temperature Regulation: Soil acts as a thermostat for the ecosystem. When soil warms, it can affect local climates, potentially leading to further warming in a cyclical manner.
- Greenhouse Gas Production: When organic carbon is lost through decomposition due to increased soil temperature, it releases more carbon dioxide and methane, potent greenhouse gases.
- Ecosystem Resilience: A decrease in SOC can diminish the resilience of ecosystems, making them less adaptable to climate stresses. This can lead to changes in biodiversity and habitat functionality.
Understanding these feedback mechanisms is essential for developing adaptive management strategies to mitigate climate impacts.
Understanding these feedback mechanisms is essential for developing adaptive management strategies to mitigate climate impacts.
In sum, the dynamics of heated soil and carbon are intrinsically linked to the climate crisis. Researchers and land managers must prioritize investigations into how heating affects carbon storage and emissions to devise effective interventions.
The Role of Soil Management Practices
The interaction between soil management practices and heated soil serves as a pivotal aspect in understanding how agricultural productivity, ecological stability, and climate change can be influenced. Proper management techniques can mitigate negative effects associated with increased soil temperatures. As climate change leads to more frequent instances of heated soil, these practices become increasingly essential.
Through targeted actions, farmers and land managers can create conditions that shield the soil from excessive heating. Techniques like mulching, crop rotation, and cover cropping lead to improved soil moisture retention and consequently lower soil temperatures. In this article, we explore the significance of these practices in managing heated soil and enhancing system resilience.
Conservation Techniques for Mitigating Soil Heating
Conservation techniques are critical when aiming to reduce soil heating. These practices not only contribute to more stable soil temperatures but also promote nutrient availability and enhance overall soil health. Some effective techniques include:
- Mulching: Using organic or inorganic materials to cover the soil surface. Mulch acts as an insulator, minimizing the amount of solar radiation that penetrates the soil.
- Cover cropping: Planting specific crops during off-seasons or when main crops are not growing can help protect the soil from direct sun exposure. Legumes, for example, not only maintain soil cover but also enhance nitrogen levels, providing additional benefits.
- Reduced tillage: Minimizing soil disturbance helps maintain the soil structure and supports moisture retention. Less exposed soil often equates to lower temperatures.
Implementing these techniques requires an understanding of both local conditions and crop varieties. Farmers who adopt conservation practices often see improved resilience in their crops. Improved microbial communities beneath the surface contribute positively to nutrient cycling.
Impact of Agricultural Practices on Soil Temperature
Various agricultural practices can either exacerbate or alleviate soil heating. High-intensity farming methods often expose soil to harsh conditions, enhancing its susceptibility to heat. For instance, excessive tillage can lead to soil erosion, loss of organic matter, and ultimately increased soil temperature. Important considerations include:
- Crop selection: Growing heat-resistant crop varieties can help reduce temperature stress. Farmers should consider local climate conditions when selecting their crops.
- Irrigation management: Over-irrigation can significantly increase microbial activity, resulting in elevated soil temperatures. Employing more precise irrigation methods can help regulate soil temperature effectively.
- Nutrient application: Chemical fertilizers can affect soil dynamics. A well-balanced nutrient strategy that incorporates organic amendments may help maintain a suitable temperature range for crops.
Managing these agricultural practices demands a holistic approach. It requires understanding the interplay between soil health, plant growth, and environmental factors.
In summary, the role of soil management practices is crucial. Effective conservation techniques and mindful agricultural practices can significantly mitigate the challenges posed by heated soil.
In summary, the role of soil management practices is crucial. Effective conservation techniques and mindful agricultural practices can significantly mitigate the challenges posed by heated soil.
Balancing these components will likely improve crop resilience and sustainability in the face of climate change.
Case Studies in Heated Soil Research
Research around heated soil is crucial to understanding its implications for agriculture, ecology, and climate. Through case studies, researchers gather localized data that allows them to observe soil heating effects under various conditions. These real-world studies provide essential insights that laboratory settings may not capture entirely. The benefits of examining these case studies include identifying specific heat effects on growth and yield, understanding microbial shifts in community structure, and monitoring carbon dynamics in soil. Furthermore, they help establish evidence of phenomena observed on wider scales, contributing critical knowledge to global understandings of soil heating.
Localized Studies of Soil Heating Effects
Localized studies have revealed that the effects of heated soil can vary significantly based on geographical locations and environmental factors. In agricultural regions, these studies often focus on the direct impacts of elevated temperatures on crop yield. For instance, a case study conducted in California highlighted how increased soil temperatures during critical growth periods negatively affected tomato yield, causing a reduction of up to 25%.
Additionally, localized research sheds light on how different soil management practices can mitigate these adverse effects. Studies examining various irrigation techniques in arid regions showed that applying mulch can lower soil temperature and improve moisture retention, ultimately promoting better plant growth. This localized approach allows for realistic solutions tailored to specific regions, fostering sustainable land use practices.
Global Trends in Soil Temperature Variability
The examination of global trends in soil temperature variability allows scientists to understand larger patterns and correlations. Data aggregated from multiple regions demonstrates an increasing trend in soil temperatures worldwide. One notable finding comes from research indicating that global soil temperatures have risen by approximately 0.5 degrees Celsius since the early 1980s. This upward trend has significant implications for ecosystems and agriculture.
In regions like the Arctic, increased soil temperatures have been linked to the thawing of permafrost, releasing methane and carbon dioxide, which exacerbates climate change. On the other hand, agricultural systems across Europe have shown shifts in planting dates and crop types due to warming soils. Studying these patterns helps identify how heated soil interacts with climatic changes, guiding future agricultural practices and policy decisions.
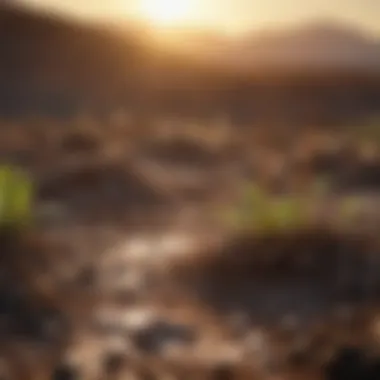
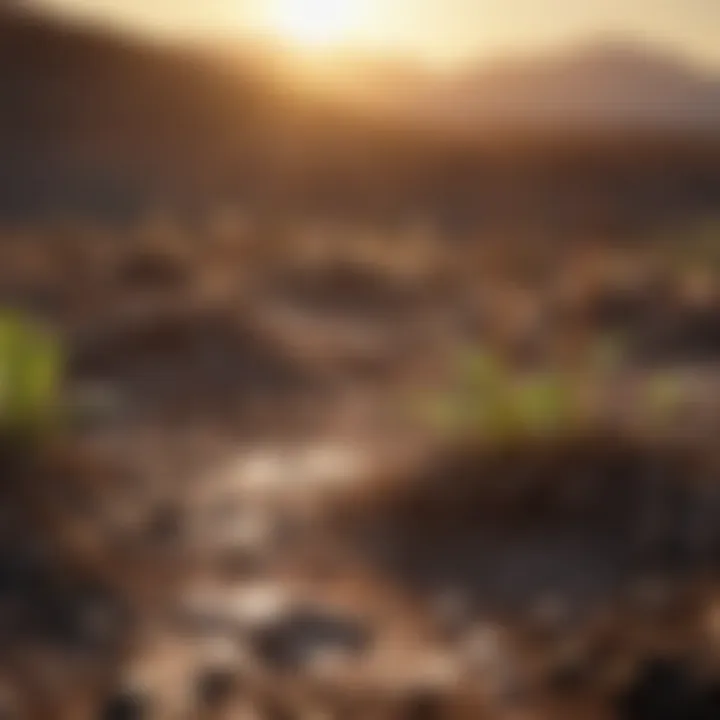
"Understanding localized and global trends in soil heating is essential for developing strategies that address food security and climate resilience."
"Understanding localized and global trends in soil heating is essential for developing strategies that address food security and climate resilience."
Through comprehensive case studies, researchers can create predictive models and recommendations that improve agricultural practices and contribute to ecological sustainability. The knowledge gained from these studies emphasizes the critical nature of ongoing research in heated soil and its far-reaching implications.
Predictive Models for Future Soil Heating Scenarios
Predictive models for future soil heating scenarios are essential for understanding how soil temperature changes may influence various sectors, particularly agriculture and ecology. These models help researchers anticipate shifts in soil health and productivity as climate conditions evolve. By employing both historical data and contemporary trends, scientists can create frameworks that project potential future changes.
As the unpredictability of climate change grows, the relevance of accurate predictive models cannot be overstated. Such models provide valuable insights for decision-makers in policy and land management. They also allow for better planning regarding crop selection and soil management practices, ensuring sustainable agricultural productivity.
Simulation of Climate Impacts on Soil Temperature
Simulating climate impacts on soil temperature typically involves complex algorithms and climate scenarios. These simulations assess many factors, including atmospheric CO2 levels, precipitation patterns, and temperature fluctuations. The data generated can reveal how these factors interact with soil composition and microbial activity.
Key elements of these simulations include:
- Data Collection: Accurate historical climate data form the backbone of predictive modeling. Information on past temperatures, rainfall, and other climatic factors are integrated into the models.
- Modeling Techniques: Various techniques such as cellular automata and machine learning are used to create sophisticated models that can simulate potential future scenarios.
- Sensitivity Analysis: This helps identify which variables most significantly impact soil temperature, allowing researchers to pinpoint areas of concern.
Simulated climate impacts highlight critical vulnerabilities in soil ecosystems, prompting proactive management.
Simulated climate impacts highlight critical vulnerabilities in soil ecosystems, prompting proactive management.
Potential Outcomes for Ecosystems and Agriculture
The outcomes derived from predictive models can have far-reaching implications. In agriculture, adjusting to elevated soil temperatures is crucial for maintaining crop yields. Understanding potential temperature increases can guide planting schedules and species selection.
Consider the following potential outcomes:
- Altered Crop Growth: Some crops may thrive in warmer conditions, while others could struggle or fail outright.
- Nutrient Cycling Changes: Elevated temperatures can enhance or inhibit microbial activity, directly influencing nutrient availability.
- Pest Dynamics: Changes in soil temperature may lead to shifts in pest populations, affecting crop resilience and yield.
In terms of ecosystems, alterations in soil temperature can impact plant diversity and overall biodiversity. Ecosystem balance may be disrupted, leading to an increased vulnerability to diseases and pests.
Epilogue
The conclusion of this article emphasizes the significance of heated soil as an interdisciplinary concern that influences agriculture, ecology, and climate. Understanding the effects of soil temperature is paramount for developing effective agricultural strategies and ecological preservation methods. The observations discussed in the previous sections highlight how heated soil alters plant growth, microbial interactions, and nutrient dynamics. Furthermore, they underline the critical relationship between soil temperature and climate change.
Specifically, the implications for agricultural productivity are profound. Heated soils can disrupt the balance of nutrient availability, potentially leading to reduced crop yields. Adaptive management practices must be adopted to mitigate these effects, ensuring that agriculture remains viable under changing climatic conditions.
In addition, recognizing the ecological impacts of heated soil is important for conserving biodiversity and maintaining ecosystem services. The shifts in microbial community structure and function can lead to broader ecosystem disruptions. Thus, studying these changes contributes valuable insights into ecological resilience.
Moreover, the feedback loops between soil heating and climate change must not be overlooked. As soil temperatures increase, the carbon release from soils may further exacerbate climate change, creating a cycle that is difficult to break.
In summary, this article brings to the forefront the need for a deeper understanding and proactive measures in relation to heated soil. Researchers, agriculturalists, and policymakers must work collaboratively to address challenges and implement solutions to promote sustainability in both agriculture and ecological contexts.
Summary of Key Findings
The research presented in this article aligns on several key findings regarding heated soil phenomena:
- Heated soils may alter nutrient availability, severely impacting agricultural productivity.
- Changes in microbial communities can modify soil health and affect ecosystem functions.
- The dynamic between soil heating and climate change can create chronic issues for biodiversity and agricultural sustainability.
- Management practices must evolve to address the raising soil temperatures effectively.
These findings indicate the necessity of continued research and adaptive practices in agriculture to counteract the implications of heated soil.
Future Directions in Heated Soil Research
Looking ahead, future research in heated soil should focus on a few essential areas:
- Long-term monitoring of soil temperature effects on diverse ecosystems.
- Innovative agricultural practices that reduce soil heating while enhancing crop resilience.
- Modeling climate impacts on soil dynamics to predict future scenarios and inform strategies for adaptation.
- Interdisciplinary collaboration among ecologists, agronomists, climatologists, and policymakers to develop comprehensive plans addressing the multifaceted challenges posed by heated soils.
Continued investigation into these topics is vital for ensuring food security, preserving ecological integrity, and mitigating climate change impacts. As scientific understanding deepens, practical applications will emerge, paving the way for more resilient agricultural systems and healthier ecosystems.