Understanding the Hidex Liquid Scintillation Counter
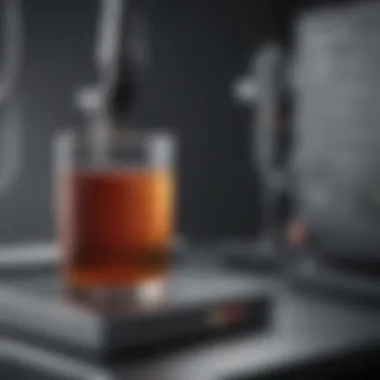
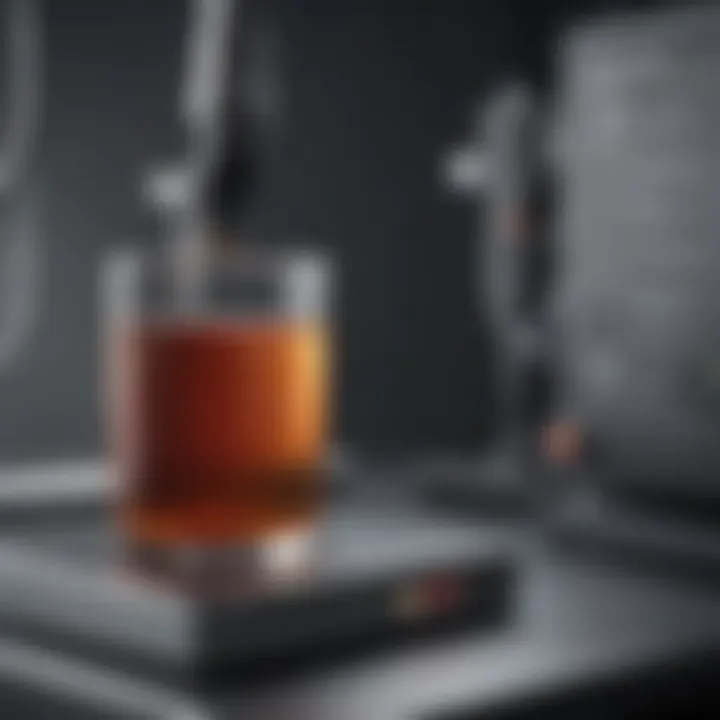
Intro
Liquid scintillation counting is a method widely used in various scientific fields to measure ionizing radiation. Among the devices that facilitate this measurement, the Hidex Liquid Scintillation Counter stands out for its precision and operational effectiveness. With numerous applications in fields like biology, chemistry, and environmental science, this instrument represents a critical tool for researchers aiming to uncover the mysteries of radioactive materials.
In this article, we will explore the functionalities of the Hidex Liquid Scintillation Counter, integrating its underlying principles with its significant applications. Understanding this technology not only illuminates its role in scientific endeavors but also helps demystify complex processes for a broader audience, from students to seasoned professionals.
Key Concepts
Definition of the Main Idea
At its core, the Hidex Liquid Scintillation Counter is designed to measure low levels of radioactivity in liquid samples. This device captures the scintillation light produced when ionizing radiation interacts with specific chemical compounds in a liquid, consequently allowing for the quantification of radioactive isotopes present in that sample. What sets the Hidex apart is its ability to do this with heightened efficiency and accuracy compared to older, more traditional measuring techniques.
Overview of Scientific Principles
The operational principle relies heavily on the interaction between radiation and liquid scintillators. When a radioactive particle passes through a scintillator, it excites the molecules within the liquid, causing them to emit photons of light. This emitted light is then detected and counted by the Hidex, which translates these signals into quantifiable data on radiation levels. Key components involved include:
- Scintillation Cocktail: A mixture of solvents and fluorescent compounds in which the radioactive sample is placed.
- Photomultiplier Tube: A device that detects the photons emitted by the scintillator and amplifies the signal to usable levels.
- Data Acquisition System: A computerized system that processes the detection events and generates meaningful results.
Understanding these components provides context to the significance of the Hidex counter within the landscape of radiation measurement.
Current Research Trends
Recent Studies and Findings
As research continues to evolve, so do the methodologies applied in radiation detection. Articles published in current scientific journals highlight the increasing reliance on liquid scintillation counting for studying environmental pollutants, such as radioactive isotopes in water and soil samples. These studies not only enhance our understanding of environmental health but also underscore the utility of the Hidex counter in conducting breadth and depth in data analysis.
Significant Breakthroughs in the Field
One notable advancement is the development of enhanced scintillation cocktails that yield greater light yield and improved discrimination between different types of radiation. With such breakthroughs, researchers can expect greater sensitivity and specificity when utilizing the Hidex Liquid Scintillation Counter for diverse experimental requirements. Furthermore, ongoing improvements in digital technology are optimizing data processing speeds, allowing scientists to handle larger datasets efficiently.
"The power of the Hidex Liquid Scintillation Counter lies not just in its ability to measure radioactivity; it's in how it continues to adapt and evolve alongside scientific demands and technological advancements."
"The power of the Hidex Liquid Scintillation Counter lies not just in its ability to measure radioactivity; it's in how it continues to adapt and evolve alongside scientific demands and technological advancements."
As we delve deeper into this article, we will uncover more about how the Hidex counter is revolutionizing research methodologies across various fields.
Intro to Liquid Scintillation Counting
Liquid scintillation counting stands as a cornerstone in the realm of radiation detection and measurement. This technique leverages the unique capabilities of scintillation materials to identify and quantify radioactive substances, playing a crucial role across various scientific domains such as biology, chemistry, and environmental science. Adopting this method not only enhances the accuracy of measurements but also widens the scope of applications, from tracing metabolic pathways in biological research to monitoring environmental levels of radioactive pollutants.
Historical Context
The inception of liquid scintillation counting can be traced back to the early to mid-20th century. In the wake of nuclear physics advancements post-World War II, researchers sought more refined methods to detect radiation. Traditional methods like Geiger counters, while effective, had limitations in sensitivity and the ability to differentiate between various isotopes. The introduction of scintillation counters marked a significant upgrade. Scientists discovered that certain liquids, when excited by radiation, emitted flashes of light, which could be counted and analyzed.
Fast forward to today, liquid scintillation counting has undergone tremendous evolution, adapting to the needs of modern science. The emergence of sophisticated instruments such as the Hidex Liquid Scintillation Counter embodies these advancements, allowing for enhanced precision and versatility in radiation detection.
Basics of Scintillation Counting
At the core of scintillation counting is a straightforward yet elegant principle: when ionizing radiation interacts with certain materials, it produces scintillation light. Here’s how it works:
- Interaction: When a radioactive particle travels through the scintillation liquid, it interacts with the molecules in that liquid.
- Photon Emission: This interaction causes the molecules to become excited, and, as they return to their stable state, they emit photons (light).
- Detection: The emitted light is then detected by photomultiplier tubes, which convert the light signals into electrical signals. These signals are counted and analyzed, ultimately providing quantifiable data about the radioactivity present in the sample.
The versatility of liquid scintillation counting lies in its capability to measure a wide range of isotopes, including beta and gamma-emitting radionuclides. This feature proves particularly advantageous in biological studies, environmental assessments, and nuclear chemistry, where distinct measurement requirements arise.
As we delve deeper into the functionalities and significance of the Hidex Liquid Scintillation Counter, it's worth noting how its sophisticated design intertwines with the foundational principles discussed here, facilitating advancements in various research fields.
Overview of the Hidex Liquid Scintillation Counter
The Hidex Liquid Scintillation Counter stands out as a crucial instrument in contemporary radiation measurement. More than just a piece of equipment, it embodies the convergence of design, function, and technological advances that facilitate precise detection of radioactive isotopes in various applications. This section highlights the intricacies of its design and the technical specifications that underpin its functionality, which ultimately positions the Hidex system at the forefront of scintillation counting.
Design and Functionality
The design of the Hidex Liquid Scintillation Counter is a blend of innovation and practicality. The sleek structure allows for easy operation, minimizing the learning curve for new researchers while providing seasoned professionals with the sophistication they need. The counter employs a liquid scintillation technique that involves the use of special solvents mixed with scintillation cocktails. When a radioactive particle interacts with these solvents, it generates light, which is subsequently detected by photomultiplier tubes.
A key aspect of its functionality lies in the automation features embedded within the system. This automation not only streamlines sample processing but also enhances reproducibility, a critical factor for ensuring accurate research results. Users can preset various parameters, such as counting time and sample type, facilitating a user-friendly experience that enhances productivity in fast-paced research environments.
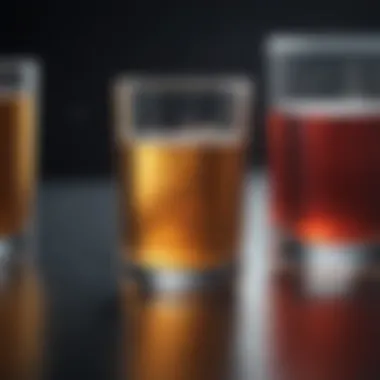
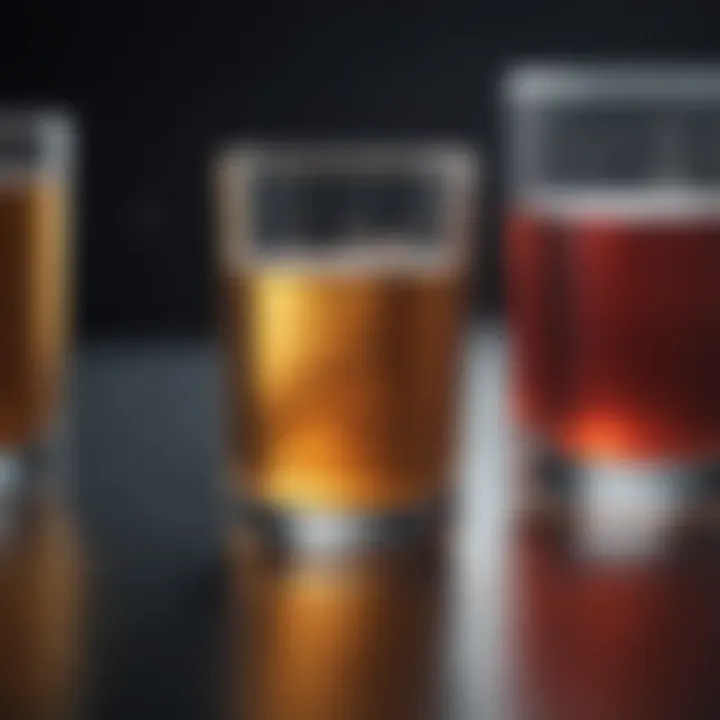
"The Hidex Counter is designed to simplify complexity, making it a reliable partner in any research laboratory."
"The Hidex Counter is designed to simplify complexity, making it a reliable partner in any research laboratory."
Moreover, the compact footprint of the Hidex system is notable. It allows the counter to fit seamlessly into different laboratory setups, whether in academic institutions or industrial environments. The clever engineering minimizes noise and interference, thereby heightening sensitivity and precision in counting applications.
Key Technical Specifications
Understanding the specifications of the Hidex Liquid Scintillation Counter provides insight into its capabilities. Here are some key details that stand out:
- Counting Efficiency: The device is designed to have high counting efficiencies for both low-energy beta particles and gamma rays, making it versatile for various isotopes in different studies.
- Energy Range: It operates efficiently across a broad energy range, accommodating a variety of isotopes like Carbon-14, Tritium, and Iodine-125.
- Sample Capacity: Depending on the model, the Hidex counter can hold multiple samples simultaneously, reducing the time required for batch processing.
- User Interface: It features an intuitive interface with touchscreen technology, enhancing accessibility and ease of use for people of varying technical backgrounds.
- Output Formats: The instrument can export data in several formats, making it easy to integrate with other software tools and facilitating data analysis.
In summary, the design and specifications of the Hidex Liquid Scintillation Counter are engineered to meet the evolving needs of radiation measurement. It is not just about capturing data; it is about the reliability, efficiency, and adaptability that this equipment brings to the table, propelling advancements in biological, chemical, and environmental research.
How Liquid Scintillation Counters Work
Understanding how liquid scintillation counters operate is essential for grasping the significance of these instruments, particularly the Hidex Liquid Scintillation Counter. This section focuses on the underlying principles and detection processes that enable these devices to measure radiation effectively. The workings of these counters can be compared to a finely-tuned orchestra, where each component plays a crucial role in achieving an accurate output. By breaking down these components, we can appreciate their synergy, enhancing our comprehension of their applications and benefits in various research domains.
Principle of Operation
In essence, liquid scintillation counting relies on the principle of light emission when radiation interacts with specific scintillation materials dissolved in a liquid. The process initiates when radioactive particles or photons pass through the liquid scintillator, causing certain molecules within to transition to an excited state, much like a balloon rising higher and higher until it can no longer contain the energy within. Once these scintillation molecules return to their ground state, they emit light—typically in the form of photons—which can be thought of as the final pop of that balloon.
This emitted light is then transferred to a photomultiplier tube (PMT), an incredibly sensitive device that converts light into a measurable electrical signal. The PMT amplifies this signal, allowing for detection of even the faintest bursts of light induced by low-energy beta particles or gamma radiation. Consequently, the number of photons translated into electrical impulses corresponds to the amount of radioactive decay occurring in the scintillator, creating a direct link between radiation and quantifiable output.
Several factors influence the efficiency of this scintillation process, including the choice of solvent and scintillator material. Moreover, when using the Hidex Liquid Scintillation Counter, the system emphasizes optimizing these factors for maximum sensitivity. Using high-quality scintillation cocktails, often composed of organic solvents mixed with specific solutes, can significantly enhance light output and detection efficiency.
Detection Process
The detection process of a liquid scintillation counter combines several technological marvels. Initially, when light from the scintillation event is emitted, it travels through the liquid medium, bouncing and scattering until it reaches the photomultiplier tube. Think of it as a game of pinball where the photons ricochet off various components until they ultimately trigger a successful score.
Once the PMT receives the light, it starts to convert it into an amplified electrical pulse. This process involves several stages, including:
- Photoelectric Effect: The emitted photons strike the surface of the PMT, causing the release of photoelectrons.
- Electron Multiplication: These released electrons move through a series of dynodes, which are metallic surfaces that create further electrons upon impact. Essentially, each electron that strikes a dynode produces more electrons.
- Signal Output: By the time these electrons reach the anode, the original single photon can generate a cascade effect, resulting in thousands of electrons being released, creating a significant electrical signal.
This amplified signal is then processed by sophisticated software designed to analyze and present the data. The readout provides researchers with critical information about the sample being analyzed, including the energy of the detected radiation and its intensity.
"The effectiveness of liquid scintillation counters, particularly the Hidex model, lies in their ability to detect low levels of radiation with high precision, making them indispensable in research from biology to nuclear physics."
"The effectiveness of liquid scintillation counters, particularly the Hidex model, lies in their ability to detect low levels of radiation with high precision, making them indispensable in research from biology to nuclear physics."
By uncovering the intricacies of how liquid scintillation counters work, particularly focusing on the principles of operation and detection processes, we set a solid foundation for understanding their applications and advantages in research endeavors.
Applications of Hidex Liquid Scintillation Counter
Liquid scintillation counting (LSC) has carved a niche in several scientific fields, showcasing its adaptability and robustness as a radiation measurement tool. The Hidex Liquid Scintillation Counter is a notable player in this arena, meeting the diverse demands of researchers across biological, environmental, and nuclear disciplines. The significance of understanding these applications not only highlights the counter's versatility but also its pivotal role in advancing scientific knowledge.
Biological and Medical Research
In the realm of biological and medical research, the Hidex counter is indispensable. It is primarily used to track radioactive isotopes in various samples, allowing researchers to analyze metabolic pathways, drug distribution, and cellular reactions with high precision. For instance, when studying pharmacokinetics, scientists often label drugs with radioisotopes. By using the Hidex counter, they can monitor the concentration of the drug in biological systems over time. This is crucial for understanding how drugs are absorbed, distributed, metabolized, and ultimately excreted by the body.
Moreover, the counter supports radioimmunoassays, which are vital for measuring hormone levels or detecting substances in blood. These assays capitalize on the sensitivity of liquid scintillation counting to low activity concentrations, leading to more accurate diagnoses and research outcomes.
- Key Benefits:
- High Sensitivity: Detects minute levels of radioactivity.
- Rapid Analysis: Provides quick results, essential for time-sensitive studies.
Overall, the Hidex Liquid Scintillation Counter serves as a cornerstone in biological research, facilitating groundbreaking findings and enhancing our understanding of health and disease.
Environmental Science
Environmental science also stands to benefit significantly from the functionalities of the Hidex counter. One of the main goals in this field is accurate assessment of radioactive contamination in ecosystems. Liquid scintillation counters like Hidex excel in measuring low levels of radioactivity in environmental samples, such as soil, water, and air.
For example, after a nuclear incident, researchers need to assess the extent of contamination. The Hidex counter enables them to measure the levels of isotopes like cesium-137 or strontium-90 in various samples effectively. This not only helps in understanding the immediate impact of radiation on the environment but also aids in developing remediation strategies to mitigate harmful effects.
- Considerations:
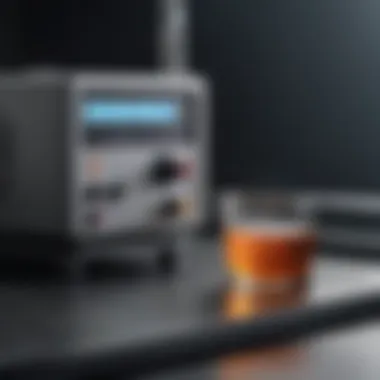
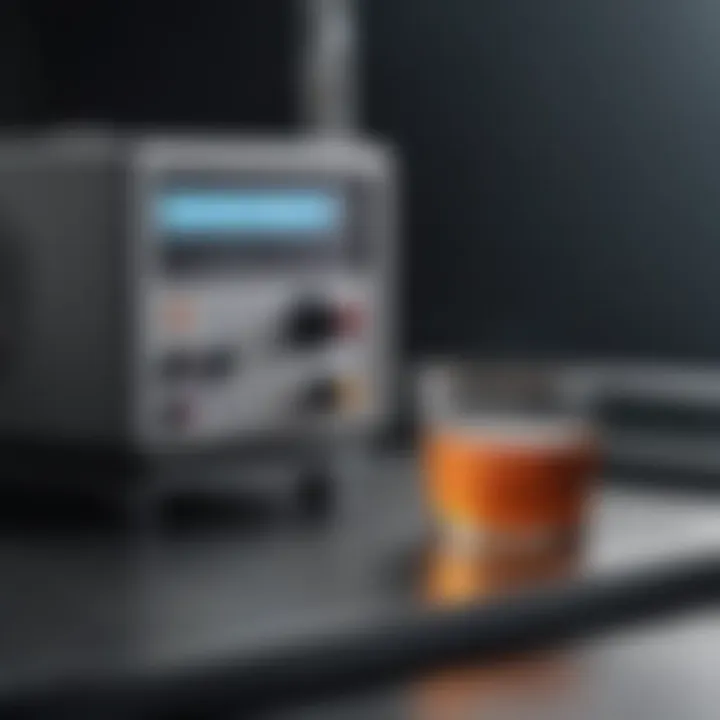
- Adaptability: Works with diverse sample types and detection needs.
- Regulatory Compliance: Meets standards for environmental monitoring.
In summary, by employing the Hidex Liquid Scintillation Counter, environmental scientists can gain insights into radioactive pollutants and their ecological implications, making strides towards healthier ecosystems and informed policy decisions.
Nuclear Chemistry
Nuclear chemistry involves the study of radioactivity and its chemical properties. The Hidex counter plays a vital role in facilitating this type of research, especially in the realms of radioactive decay and the synthesis of new isotopes. The precision of this instrument allows chemists to quantify radioisotopes in various chemical reactions, providing critical data about reaction pathways and kinetics.
A prime application is in the field of radiopharmaceutical development. These compounds, used in diagnostic imaging and therapy, often incorporate radioisotopes. The Hidex counter allows researchers to measure the yield and purity of these compounds with high sensitivity. Additionally, it helps in assessing the stability of radioactive materials over time, which is crucial for ensuring safety and efficacy.
- Important Aspects:
- Quantitative Analysis: Enables detailed studies on radioisotope behavior.
- Long-Term Stability Checks: Critical for storage and application of radiopharmaceuticals.
Advantages of Using Hidex Liquid Scintillation Counter
The Hidex Liquid Scintillation Counter stands out as a valuable tool in the realm of radiation measurement, offering a mix of benefits that make it indispensable in various scientific fields. Understanding its positive aspects can shine a light on why many researchers and labs opt for this specific counter. The importance of this section lies in appreciating how its design enhances performance and output, making it a key player in advancing scientific research.
Sensitivity and Precision
One of the most compelling advantages of the Hidex Liquid Scintillation Counter is its unparalleled sensitivity and precision. This tool boasts a remarkable ability to detect low levels of radiation, capturing data that other counters might miss. High sensitivity is crucial, especially in fields like biological research where minute amounts of radioactive material are often used.
- Accuracy in Results: The precise nature of measurements enables researchers to make informed decisions based on reliable data. This is not just about getting a number; it’s about understanding the implications that number has in a broader context.
- Lower Detection Threshold: The counter can record even the faintest signals, which significantly enhances the reliability of the results obtained. When dealing with radioactive tracers in biological assays, such precision can lead to major breakthroughs in understanding complex processes.
Furthermore, the accuracy of the Hidex counter minimizes the risk of false positives and negatives, which can be detrimental in experimental settings. Thus, it ensures that every result contributes effectively to the body of knowledge being built by researchers.
Versatility in Applications
The versatility of the Hidex Liquid Scintillation Counter cannot be overstated. It's not confined to just one area; its applications span across a diverse array of fields, including biological research, environmental monitoring, and nuclear chemistry. This wide-ranging utility is what makes it attractive for many laboratories.
- Biological and Medical Research: The counter is adept at analyzing different types of samples, whether they are tissues, fluids, or cell cultures. Research involving the metabolism of radiolabeled compounds benefits immensely from its capabilities, as it allows for a detailed investigation of biological pathways.
- Environmental Applications: In environmental science, the analysis of radioactive contaminants in soil and water sources showcases how this counter aids in safeguarding ecological health. It helps in monitoring radiation levels that could pose risks to public health and safety.
- Nuclear Chemistry: The system’s application extends to the study of radiochemical processes, where precision is paramount in understanding nuclear reactions and decay chains.
Even beyond these examples, the adaptability of this counter allows researchers to customize setups for specific experiments, ultimately enhancing workflow efficiency.
"The Hidex Liquid Scintillation Counter is a bridge between the intricate dance of atoms and the clarity of understanding that drives scientific discovery."
"The Hidex Liquid Scintillation Counter is a bridge between the intricate dance of atoms and the clarity of understanding that drives scientific discovery."
Overall, the sensitivity and precision of the Hidex Liquid Scintillation Counter, coupled with its versatile application across numerous scientific disciplines, underline its significance in advancing our understanding of radiation in various contexts. This counter doesn’t just measure; it contributes to a greater dialogue in science.
Comparison with Other Scintillation Technologies
In the realm of scintillation counting, understanding the differences and advantages between various technologies is essential for a comprehensive grasp of radiation detection methods. Scintillation counters serve as critical tools in diverse fields like medical research, environmental studies, and nuclear energy. The Hidex Liquid Scintillation Counter stands out, yet it is vital to compare it with solid-state scintillation technologies and other modern innovations. These comparisons reveal insights that inform choices in practical applications, supporting both researchers and industry professionals in optimizing their techniques.
Solid-State vs. Liquid Scintillation Counting
When delving into the specifics of scintillation technology, one must examine the contrast between solid-state and liquid scintillation counting. Solid-state detectors employ materials like silicon or germanium to register radiation. These detectors tend to offer better energy resolution compared to their liquid counterparts. However, they often come with higher costs and require stringent operational conditions.
On the flip side, liquid scintillation counters, such as those from Hidex, use liquid scintillation cocktails to facilitate the detection process. This offers several noteworthy benefits:
- Higher Sensitivity: Liquid scintillation counters can detect lower levels of radiation, which is crucial in many biological and environmental applications.
- Broader Range of Applications: They are able to analyze different types of isotopes, including beta-emitting isotopes, which sometimes can be missed by solid-state detectors.
- Cost-Effectiveness: Generally, liquid scintillation counters are more affordable for substantial counts, making them more accessible for various laboratories.
While both technologies have their merits, the choice often swings based on the specific type of experiment or measurement required. The versatility of liquid scintillation counters makes them a preferred choice in many laboratories.
Modern Innovations in Scintillation Detection
Technological advancements have sparked a notable evolution in scintillation detection methods. From improving the materials used in scintillation cocktails to enhancing the spectral sensitivity of detectors, these innovations significantly impact the efficacy of radiation measurement.
Recent developments that stand out include:
- Nano-Scintillators: These are being researched as next-gen materials that promise heightened sensitivity and rapid response times.
- Integrated Detection Systems: Systems that combine liquid scintillation counting with other detection technologies allow for multipurpose functionality in one device, delivering comprehensive data in varied contexts.
- Software Integration: Advancements in data analysis software have transformed how scientists interpret scintillation data. Enhanced algorithms lead to quicker results and better accuracy, benefiting diverse research fields.
As these modern innovations continue to unfold, they pose compelling arguments for revisiting and re-evaluating existing practices in radiation measurement. Researchers are keen to not only adapt to these changes but also leverage them towards groundbreaking discoveries.
"The evolution in scintillation technology reflects the innovative spirit of scientific inquiry itself, where continuous improvement and adaptation are the norms rather than the exceptions."
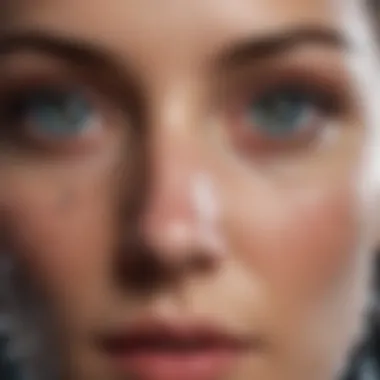
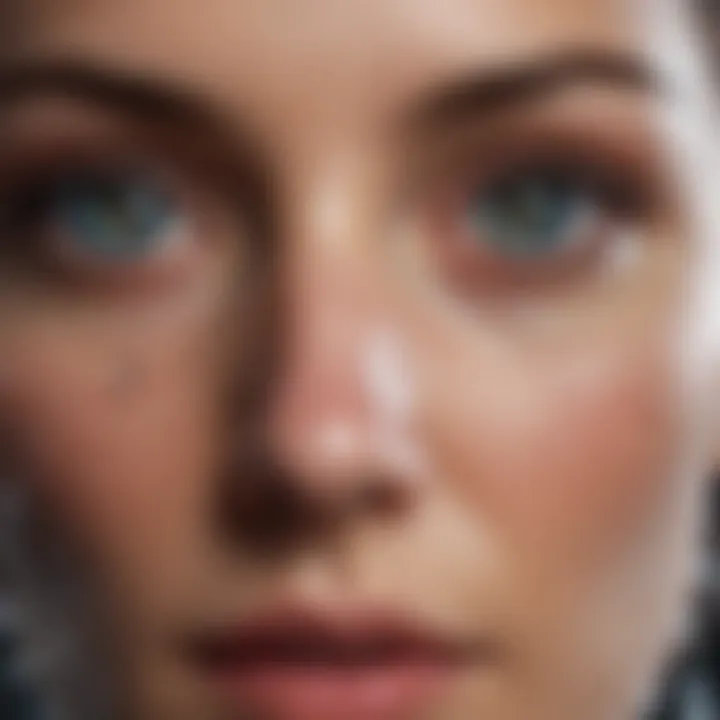
"The evolution in scintillation technology reflects the innovative spirit of scientific inquiry itself, where continuous improvement and adaptation are the norms rather than the exceptions."
Ultimately, comparing scintillation technologies is not merely about identifying the best tool; it’s about understanding which tool serves the specific context most effectively. The Hidex Liquid Scintillation Counter, with its unique functionalities, embodies the ongoing shift toward precision and versatility in scientific inquiry.
Limitations and Challenges
In the realm of scientific instrumentation, no device is without its limitations. The Hidex Liquid Scintillation Counter, despite its sophisticated capabilities, faces several hurdles that researchers must navigate. Understanding these challenges is vital for anyone utilizing this technology, as it not only shapes the interpretation of results but also influences the direction of future innovations. By recognizing its limitations, users can better prepare for the realities of radiation measurement in their respective fields.
Chemical Quenching Effects
One of the most significant hurdles faced when using a liquid scintillation counter is the phenomenon known as chemical quenching. This term refers to the reduction in light output that occurs when certain substances interact with the scintillation medium. For example, if a sample contains high concentrations of certain solutes or solvents, it may absorb some emitted light, leading to inaccurate readings. This can be especially problematic in biological studies where complex mixtures are present.
In practical terms, these effects can muddy the waters quite a bit. High levels of quenchers can lead to a necessity for calibration, which often depends on the specific sample matrix. Many researchers have to work through a series of calibration steps, adjusting for the presence of these interfering substances.
What's the bottom line? To ensure accurate measurements, it’s crucial to implement routine checks for quenching effects. This may involve using standards to gauge the scintillation efficiency before delving into sample analysis. While this adds time and complexity, it is an unavoidable aspect of achieving reliability in results.
Regulatory and Safety Considerations
The utilization of the Hidex Liquid Scintillation Counter engages a web of regulatory guidelines and safety protocols that must be adhered to. Understanding these regulations is not just advisable; it's often a legal necessity, especially in academic and industrial labs that deal with radioactive materials.
The handling of radioactive samples requires strict compliance with organizations like the Environmental Protection Agency and the National Nuclear Security Administration. These entities dictate how radiation is managed, which extends to the operation of scintillation counters. Issues can include not just the management of waste but also personnel training, equipment maintenance, and incident reporting.
Moreover, laboratories must ensure proper shielding and containment measures are in place to minimize exposure risks. This involves regular safety audits and potentially even environmental monitoring, which incur additional costs and time. Hence, researchers must also factor in their institutional regulatory environment and stay updated on any changes that may affect operational procedures.
"Safety begins with good laboratory practices and an understanding of the regulatory environment; ignoring either can lead to choices that have dire consequences."
"Safety begins with good laboratory practices and an understanding of the regulatory environment; ignoring either can lead to choices that have dire consequences."
Future Prospects of Liquid Scintillation Counting
The future of liquid scintillation counting holds considerable promise, and its continued evolution serves as a beacon of innovation in radiation measurement technologies. As the scientific community pushes boundaries in research—be it in environmental science, nuclear chemistry, or medical diagnostics—it's essential to consider how advancements in liquid scintillation counting can facilitate such endeavors.
Advancements in Detectors
Recent developments in detector technology are shaping the landscape of liquid scintillation counting. These advancements vary from improved detection efficiencies to enhanced energy resolution. For instance, integrating materials like silicon photomultipliers (SiPMs) into these systems allows for greater sensitivity, significantly reducing the minimum detectable activity of samples.
One notable progress is the incorporation of automated calibration systems. This transition not only improves the reliability of the data but also streamlines the time researchers invest in these processes. With such enhancements, the accuracy of measurements taken during time-sensitive experiments can increase, allowing for more precise detection of low-energy beta emitters in biological samples.
Moreover, scientists are also looking at miniaturization. Smaller, more compact detector designs can be made portable, expanding their usability in field studies where quick assessments are critical. This mobility opens new doors for applications previously limited by bulkiness and size constraints of traditional systems.
Ultimately, focusing on advancements in detectors is not just about improving performance; it's about creating opportunities for more intricate and diverse research pathways.
Integration with Emerging Technologies
As we gaze into the crystal ball of technology, the integration of liquid scintillation counting with emerging technologies stands out prominently. For example, the collaboration between scintillation counters and machine learning algorithms presents a fascinating avenue of exploration. The ability of these algorithms to analyze patterns in vast datasets collected can dramatically enhance interpretation and application in experimental settings.
Artificial intelligence can optimize counting protocols, such as adjusting settings based on real-time feedback from samples. This real-time adaptation can lead to reduced errors and improved throughput in laboratories where time and accuracy are paramount.
Additionally, the push towards automation in laboratories means that liquid scintillation counters might soon be part of larger networks of interconnected devices. This could allow for not only automated readings but also seamless integration of results with other analytical instruments, positioning liquid scintillation counting as a central piece in a multifaceted scientific puzzle.
When considering these integrations, we must also think about data management and sharing. With the adoption of cloud technologies, researchers can now store and access their results remotely, enabling collaborations that were once logistically challenging.
Ending
The conclusion serves as the linchpin of our exploration into the Hidex Liquid Scintillation Counter. It synthesizes a wealth of information, drawing upon the intricate details discussed throughout the article. One of the first considerations is the sheer accuracy and reliability offered by the Hidex counter in measuring various types of radiation. This precision is not merely a technical feature; it has substantial implications for a wide array of scientific fields, from biomedicine to environmental monitoring.
Summary of Key Insights
This segment lays out the critical points highlighted during our examination:
- Operational Principles: The Hidex Liquid Scintillation Counter employs unique scintillation processes to detect and quantify radiation levels. Understanding the device’s mechanics can significantly enhance the interpretation of experimental results.
- Multifaceted Applications: Its utility spans various domains, making it indispensable in biological research, environmental assessments, and nuclear chemistry. This versatility demonstrates its relevance in modern scientific inquiry.
- Advantages Over Traditional Methods: The sensitivity and reliability offered by the Hidex instrument are paramount. Compared to older methods, it can efficiently handle complex samples and deliver prompt results, saving time and resources.
The culmination of these key insights presents a cohesive picture of the Hidex counter not only as a tool but also as a pivot around which numerous research paths revolve.
The Importance of Continued Research
The scientific community must recognize the ongoing relevance of research into tools like the Hidex Liquid Scintillation Counter. While current innovations are impressive, continuous advancements are essential to meet the growing demands for accuracy in radiation measurement.
Moreover, as environmental concerns gain more traction, the integration of new technologies with existing counter systems could lead to breakthroughs in detecting low-level radiation in various contexts. Adaptations in software and detector materials may also emerge in response to the challenges posed by modern-day analyses.
Thus, it’s clear that supporting research initiatives and fostering collaborations among scientists, universities, and industries can catalyze significant advancements in this field, ensuring that the tools we rely on for safety and understanding continue to evolve.