Illumina Analysis in Genomic Research: A Comprehensive Overview
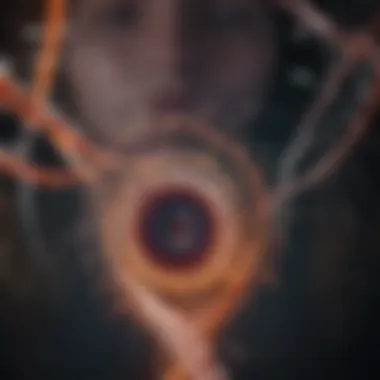
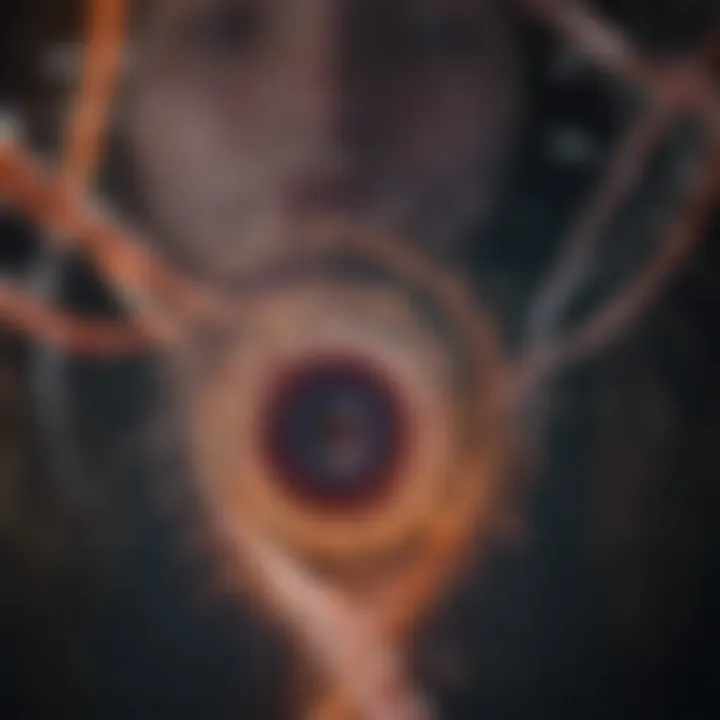
Intro
The world of genomic research has seen seismic shifts in the past couple of decades, driven predominantly by technological advancements. Among these, Illumina analysis stands out as a pivotal force, revolutionizing the ways in which we understand genetic information. By allowing researchers to unravel the complexities of DNA, RNA, and associated biological processes, Illumina technologies have become the gold standard in sequencing strategies.
When discussing genomic research, it is crucial to grasp the nuances of Illumina sequencing. This method employs a principle called sequencing by synthesis, where fluorescently labeled nucleotides are incorporated into a growing DNA strand. In simpler terms, think of it as building a LEGO structure where you need to place the right pieces at the right time. This precise methodology not only enhances accuracy but also significantly reduces the time and cost involved in sequencing tasks. As a result, Illumina analysis has enabled large-scale studies that were once deemed impractical, thus ushering in a new era of research.
In addition to its technical prowess, the implications of Illumina analysis stretch across multiple scientific disciplines. From personalized medicine and oncology to evolutionary biology and environmental genomics, its reach is broad, providing insights that can influence both public health and ecological conservation efforts.
This article will take you through the core concepts behind Illumina analysis, current research trends, and the crucial breakthroughs that keep pushing the boundaries of what’s possible in genomics.
Prelude to Illumina Analysis
The realm of genomic research has been dramatically reshaped by Illumina analysis, a pioneering methodology that has brought forth significant strides in our understanding of genetics. This section aims to underscore the centrality of Illumina analysis within the broader landscape of genomic studies, highlighting its essential components, benefits, and considerations. The advent of this technology has enabled researchers to embark on ambitious projects, paving the way for discoveries that can influence both individual health and wider public health systems.
Illumina analysis not only facilitates high-throughput sequencing but also broadens the capacity for large-scale data generation, which is crucial for a variety of studies, from basic research to applied clinical contexts. The precision and efficiency it offers make it a mainstay in many laboratories worldwide.
There are several key aspects to consider:
- High throughput: Illumina sequencing can process millions of fragments simultaneously, accelerating research timelines.
- Cost efficiency: As the technology has evolved, costs have dropped, making it more accessible for many research settings.
- Applications: The versatility of Illumina analysis spans across different fields including oncology, rare diseases, and even agricultural genetics.
Definition and Purpose
Illumina analysis is grounded in the paradigm of next-generation sequencing (NGS), which refers to a set of technologies allowing for rapid sequencing of entire genomes or targeted regions of interest. It converts the molecular architecture of DNA into readable formats that can be interpreted and analyzed by researchers. The primary purpose of Illumina analysis is to generate accurate, comprehensive genetic data that can illuminate various biological questions ranging from mutation effects in cancer to population diversity in ecology.
The significance lies in its ability to handle vast datasets and extract meaningful interpretations from them, thus facilitating a better understanding of genetic variations and their implications in health and disease.
Historical Background
The development of Illumina analysis does not spring up overnight; it's a tale woven through years of technological advancement and scientific inquiry. In the early 2000s, traditional sequencing methods were painstakingly slow and labor-intensive. Illumina emerged in a time when researchers needed more efficient tools to decode complex genomes.
The launch of the Genome Analyzer in 2006 marked a pivotal turn towards high-throughput sequencing, catapulting Illumina into prominence. Over the years, subsequent advances have led to enhanced throughput and reduced costs, making it a benchmark for sequencing technologies in research today.
"Illumina has democratized genomics, allowing countless labs to participate in and contribute to the field."
"Illumina has democratized genomics, allowing countless labs to participate in and contribute to the field."
These innovations laid the groundwork for initiatives such as the 1000 Genomes Project, which relied heavily on Illumina's technology to explore human genetic diversity. As we stand today, Illumina analysis positions itself as a cornerstone in genomics, influencing not just academic research but also clinical practices and genetic counseling.
Overview of Sequencing Technologies
Sequencing technologies have fundamentally reshaped our understanding of genetics and genomics. They allow researchers to break down complex DNA structures into manageable data, giving insights that were once thought impossible. With the rapid advancements in this field, providing a detailed overview of sequencing technologies is crucial. It helps contextualize where Illumina analysis fits within the larger picture of genomic research.
Technological advancements have brought a variety of sequencing methods to the forefront. Each technology boasts unique strengths and weaknesses, affecting both throughput and accuracy. As we dive into these different platforms, it’s essential to consider how they respond to the diverse needs within genomic research. Whether it’s studying single-nucleotide polymorphisms or conducting large-scale population studies, the right sequencing technology can make all the difference.
- High-Throughput Capabilities: Next-generation sequencing (NGS), driven primarily by platforms like Illumina, enables the sequencing of millions of fragments in parallel. This substantial throughput significantly reduces the cost per base, making it accessible for various research scopes.
- Data Depth: The amount of data generated has been transformative. With the sheer volume of genetic information, researchers can now perform comprehensive analyses that provide insights into evolutionary biology, disease mechanisms, and more.
- Technological Selection: Choosing the right sequencing platform isn’t just about cost; it’s about suitability for the specific research question. Illumina excels in short-read sequencing, while technologies like PacBio or Oxford Nanopore are advantageous for longer reads.
With these points in mind, one can appreciate the critical role that sequencing technologies play in genomic research. Each technology contributes uniquely to the understanding of genetic variations and their implications in various fields, including personalized medicine and population genomics.
Next-Generation Sequencing (NGS)
Next-Generation Sequencing (NGS) has revolutionized the genomics landscape over the past decade. Unlike traditional methods, which might take years to sequence a genome, NGS can accomplish this in a matter of days. The speed and accuracy of NGS allow researchers to delve deeper into genomic data than ever before.
The rise of NGS has unlocked numerous applications:
- Whole Genome Sequencing: This provides a complete picture of an organism’s genetic makeup, instrumental in understanding complex traits and diseases.
- Targeted Sequencing: Here, selected regions of interest are sequenced, often used in clinical diagnostics to understand specific genetic disorders.
- RNA Sequencing: This method helps in analyzing the transcriptome, providing insights into gene expression levels and alternative splicing.
Innovations in NGS technologies, particularly in Illumina, continue to broaden the scope of genomic research. The data generated is vast, necessitating robust frameworks for analysis and interpretation.
Comparative Analysis of Sequencing Platforms
When evaluating the various sequencing platforms available, one finds a rich tapestry of choices, each tailored for specific research needs. Thus, a comparative analysis gives a clearer picture of the sequencing landscape.
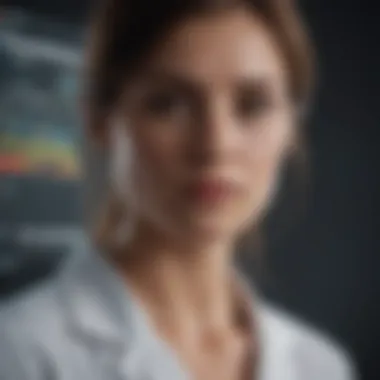
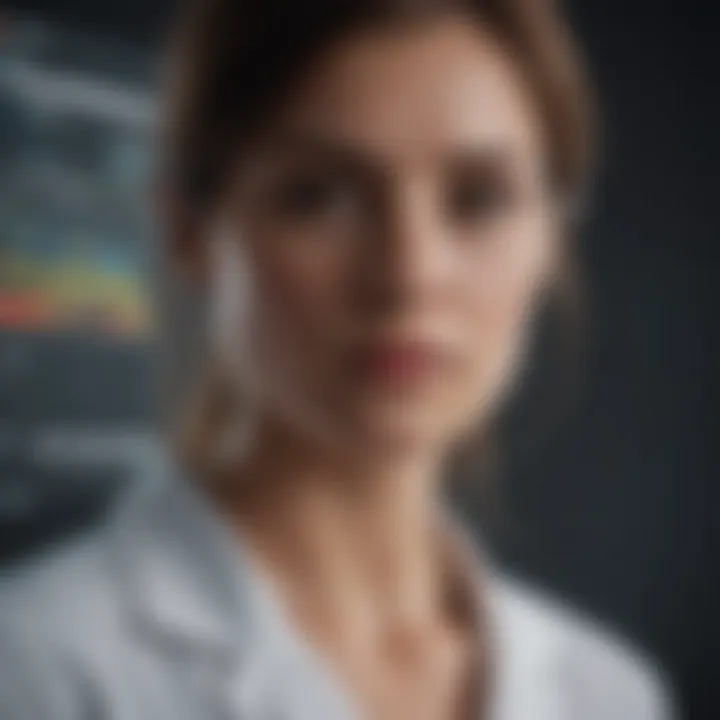
- Illumina Sequencing: Known for its high throughput and accuracy, it employs a sequencing by synthesis technology that is scalable and cost-effective.
- Ion Torrent: This platform uses semiconductor technology for sequencing and tends to have faster turnaround times and lower costs for small projects.
- PacBio: This method is noteworthy for producing very long reads, which help in resolving repetitive regions and complex structural variants, making it ideal for de novo assembly.
- Oxford Nanopore: Another technology that delivers long reads, it allows real-time data analysis, which is beneficial for certain applications, such as pathogen surveillance.
Each platform has merits and challenges, making the choice of sequencing technology a strategic decision. Factors such as project scope, budget, and desired resolution should weigh heavily in this choice.
In summary, understanding these sequencing technologies provides essential context for appreciating the role of Illumina in genomic research. It sets the stage for the more in-depth exploration of specific methodologies and their applications in the subsequent sections.
Principles of Illumina Sequencing
Understanding the principles of Illumina sequencing is crucial as it lays the groundwork for how whole genomic analysis unfolds. This technology, which has solidified its place as a leading tool in genomics, is fundamentally built on a series of meticulously designed processes. Realizing the importance of each step enhances one's appreciation for the straightforward elegance that underpins complex genomic studies. The efficiency, scalability, and accuracy of this approach have rippled across various scientific fields, making it a staple in both research and clinical environments.
Library Preparation
Library preparation is the crucial first step in any Illumina sequencing process. It involves transforming the DNA or RNA samples into a format suitable for sequencing. Imagine it as setting the stage for a grand performance; if the preparation isn’t done right, the final show might not deliver. This stage typically entails fragmenting the genome, which can be achieved through physical or enzymatic methods. The resulting fragments are then ligated to specific adapters that serve two vital functions; they initialize the sequencing process and attach unique barcodes for sample identification.
The library preparation process must be conducted meticulously, as it significantly influences the quality and efficiency of sequencing.
Some considerations include:
- Quality of starting material: High-quality, intact DNA or RNA is essential for successful library construction.
- Fragment size: The average size of the fragments determines how well they will fit into the sequencing reads.
- Adapter ligation conditions: Correct ligation conditions enhance the efficiency of subsequent sequences.
The entire library prep phase may seem rather tedious, but it undeniably sets the tone for everything that follows in the sequencing endeavor.
Cluster Generation
What happens after the library is prepared? Enter cluster generation, a fascinating stage where millions of unique DNA clusters form on a flow cell, giving rise to the bulk of the data generated during sequencing. This step ensures that each fragment of DNA is amplified, allowing the sequencing machinery to read them effectively.
Clusters are formed through a process called bridge amplification. Here’s a simplified look at it:
- Binding: The library fragments bind to the oligonucleotides on the flow cell.
- Bridge formation: Each fragment amplifies to form a bridge.
- Amplification: The bridge is then denatured, creating two identical DNA molecules. This cycle can occur thousands of times, leading to clusters that can hold hundreds of thousands of copies of the same fragment.
This high-density cluster array is vital because it becomes the canvas upon which the sequencing by synthesis will paint a vivid picture of the genetic material. The clarity of the sequencing data is directly proportional to how well the clusters have been created; thus, meticulousness here cannot be overstated.
Sequencing by Synthesis
With the clusters aligned and ready, we finally delve into the core of the Illumina sequencing technology: sequencing by synthesis. This method represents a remarkable blend of chemistry and optics, akin to fine art where the finest details come to life with precision. In this stage, nucleotides labeled with fluorescent dyes are added sequentially. As each nucleotide incorporates into the growing DNA strand, it emits a distinct fluorescent signal.
The sequencing machine captures these signals and translates them into the base calls—these sequences of "A", "T", "C", and "G" that form the backbone of genomic data.
The steps involved in sequencing by synthesis are:
- Incorporation of nucleotides: Accurate incorporation of the correct nucleotide is crucial for fidelity.
- Image Acquisition: After each incorporation, the flow cell is illuminated, exciting the fluorescent dye, and high-resolution images are taken.
- Base calling: The data from images are converted into a nucleotide sequence.
As a cornerstone for extensive genomic analysis, sequencing by synthesis increases the throughput, enabling massive amounts of data to be processed within a short time. This phase truly encapsulates the beauty of modern genomics, illustrating how sophisticated technology can efficiently unveil the building blocks of life.
The robustness of Illumina sequencing relies heavily on the synchronization of library preparation, cluster generation, and sequencing by synthesis processes. Each step is integral in creating reliable and accurate genomic data, paving the way for groundbreaking research.
The robustness of Illumina sequencing relies heavily on the synchronization of library preparation, cluster generation, and sequencing by synthesis processes. Each step is integral in creating reliable and accurate genomic data, paving the way for groundbreaking research.
Data Analysis Workflow
In the realm of genomic research, the data analysis workflow represents the backbone that supports all findings derived from sequencing techniques. A well-structured workflow is crucial for transforming raw sequencing data into meaningful biological insights. Not only does it enhance the reliability of the results, but it also streamlines the entire research process, allowing scientists to focus on interpretation rather than sifting through data inconsistencies. As Illumina analysis continues to evolve, mastering this workflow facilitates a more informed approach in various applications, from clinical diagnostics to personalized medicine.
Quality Control Measures
Before diving into the complexities of aligning and mapping strategies, it’s imperative to address quality control measures. These steps detect errors and outliers that inevitably arise during sequencing and processing phases. Think of quality control as the thorough hoovering before showcasing a tidy room; it ensures that only high-quality data enters the analysis pipeline.
Several key measures serve to uphold data integrity:
- Initial Data Assessment: This often includes checking for sequencing quality scores (like Q scores). These scores gauge the accuracy and reliability of the base calls.
- Filtering and Trimming: By removing low-quality reads or trimming off low-quality bases from the ends of reads, researchers can prevent poor-quality data from affecting calculations.
- Use of Control Samples: Incorporating control samples during sequencing helps in identifying systematic errors that may skew results.
Implementing these strategies is not merely a formality; they are essential for ensuring that subsequent analyses rest on solid ground.
Alignment and Mapping Strategies
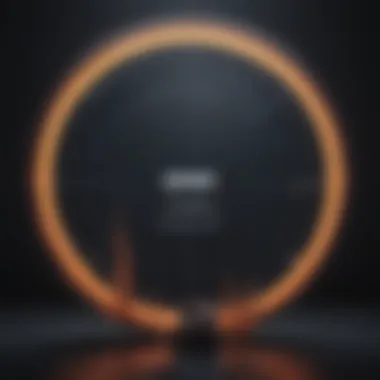
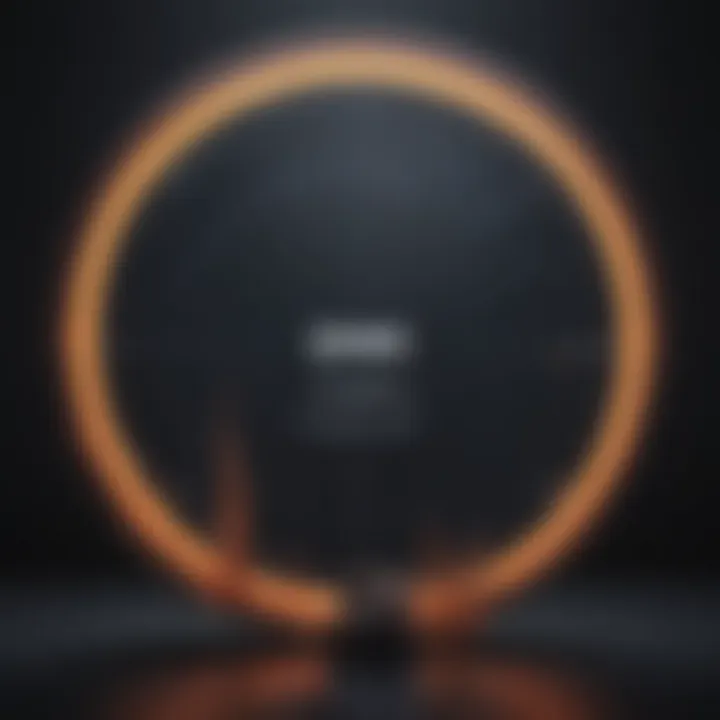
Once quality control has been firmly established, alignment and mapping strategies come into play. These steps are pivotal in situating sequencing reads onto a reference genome, thereby facilitating the identification of variations.
The most commonly utilized alignment algorithms, including BWA (Burrows-Wheeler Aligner) and Bowtie, employ sophisticated heuristics for this purpose. The choice of algorithm can drastically impact how well the reads align, affecting the accuracy of downstream analyses. Here are aspects that need consideration:
- Read Length and Complexity: Longer reads can enhance the confidence in alignment, but they may also introduce complexity in repetitive regions of the genome.
- Reference Genome Quality: It's crucial to utilize an accurate and complete reference genome, as this serves as the template for mapping.
- Utilization of Paired-end Reads: By using paired-end reads, researchers can significantly improve mapping accuracy since each read can provide positional context for its counterpart.
After alignment, researchers must also consider potential duplicates in their data, necessitating algorithms designed to mark or remove duplicate reads for accurate variant analysis.
Variant Calling Algorithms
Finally, we arrive at the stage of variant calling, where significant biological insights unfold. This portion of the workflow identifies mutations, variations, and other significant genomic changes that contribute to the understanding of biological functions.
Various algorithms exist, each with unique strengths:
- GATK (Genome Analysis Toolkit): Known for its robustness, GATK employs sophisticated statistical methods, making it a preferred choice among researchers.
- Samtools: A versatile toolkit that facilitates a range of important calculations, Samtools provides an efficient means for variant calling in an extensive genomic context.
When selecting a variant calling method, it’s important to consider:
- Sensitivity vs Specificity: Finding a balance between detecting as many variants as possible while minimizing false positives.
- Population Context: For studies involving population genomics, choosing an algorithm that incorporates demographic information may yield improved results.
In summary, the data analysis workflow is not a mere checkbox in the Illumina sequencing process; it’s a critical pathway towards extracting biology from data. Mastery of quality control, alignment, and variant calling can empower researchers to draw significant conclusions and ultimately pave the way for new scientific discoveries.
Applications of Illumina Analysis
Illumina analysis has become a cornerstone of genomic research due to its vast array of applications that span clinical, population, and personalized medicine. These applications not only facilitate groundbreaking discoveries but also enhance our understanding of complex biological systems. By diving deeper into these applications, researchers and practitioners can grasp the potential benefits and challenges tied to sequencing technologies.
Clinical Research Implications
In clinical settings, Illumina analysis plays a pivotal role in diagnosing genetic disorders and tailoring treatment protocols. It enables clinicians to identify mutations that result in specific diseases, thereby offering avenues for preventative measures and therapeutic interventions. For instance, exposing hereditary syndromes through genomic sequencing can drastically alter patient management and familial risk assessment.
A notable example is in oncology, where comprehensive genomic profiling helps identify actionable mutations. By tailoring treatment plans based on individual tumor genetics, there’s potential for improved patient outcomes. Not limited to cancer, the analysis also helps in rare disease diagnosis, shedding light on genetic variants that may be missed by traditional testing methods.
Moreover, the integration of Illumina sequencing in clinical trials allows researchers to monitor the efficacy of drugs on genetically-diverse patient populations, making biomedical research more robust and relevant.
"Genetic sequencing in clinical settings not only changes diagnosis but also revolutionizes treatment plans."
"Genetic sequencing in clinical settings not only changes diagnosis but also revolutionizes treatment plans."
Population Genomics
Population genomics is another crucial application of Illumina analysis, allowing scientists to explore genetic structures within populations and the forces that shape them. This field facilitates a greater understanding of evolutionary processes, migration patterns, and demographic histories.
By analyzing large cohorts, researchers can uncover patterns of genetic diversity and relate them to phenotypic traits. For instance, investigating single nucleotide polymorphisms (SNPs) across diverse groups can lead to insights into adaptations to environmental changes or susceptibility to diseases.
Furthermore, population genomics supports efforts in biodiversity conservation by elucidating genetic variations among species, which is critical for conservation strategies. Understanding how populations relate genetically informs management practices aimed at preserving threatened species.
Personalized Medicine Development
The application of Illumina analysis in personalized medicine signifies a shift from one-size-fits-all approaches to tailored healthcare. This transition is grounded in the belief that patients respond differently to treatments based on their genetic makeup.
Illumina analysis enables the identification of biomarkers that predict patient responses to medications, facilitating the development of individualized treatment regimens. By understanding each patient's unique genetic profile, healthcare providers can choose therapies with higher efficacy and lower risks of adverse effects.
Moreover, the rise of pharmacogenomics, which studies how genes affect a person’s response to drugs, is heavily reliant on sequencing technologies. This branch of personalized medicine helps refine medication dosages and selections, ensuring that patients receive optimal therapeutic benefits.
In summary, the applications of Illumina analysis span a wide array of fields significant to both research and healthcare. These applications not only advance our understanding of genomics but also serve to bridge the gap between complex genetic data and real-world clinical scenarios.
Challenges and Limitations
Understanding the challenges and limitations associated with Illumina analysis is crucial for researchers aiming to grasp the full spectrum of genomic research. Given that the field is rapidly evolving, comprehending these pitfalls isn't just beneficial—it's essential for making informed decisions when utilizing sequencing technologies. While Illumina has made great strides in genomic sequencing, certain hurdles remain that can affect the accuracy and reliability of results. Exploring these will help delineate the context of its application in modern research.
Data Interpretation Difficulties
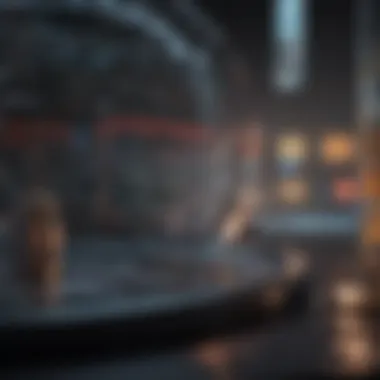
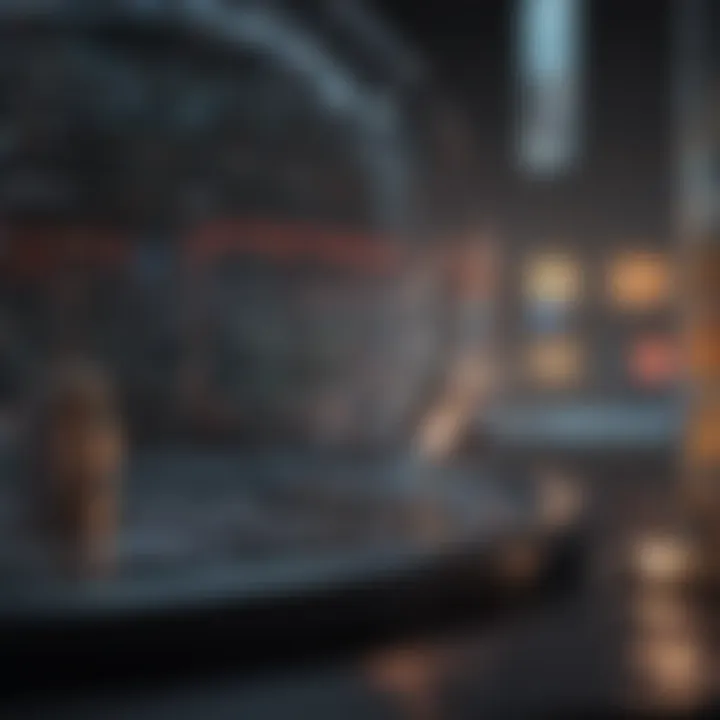
Data interpretation in Illumina analysis presents a plethora of challenges. One can't overlook that the raw data generated is enourmously complex, leading some researchers to feel like they're trying to find a needle in a haystack. The information garnered from sequencing reads must be meticulously assessed for variations, which when poorly interpreted, can lead to misleading conclusions. The sheer volume of data necessitates sophisticated computational tools and statistical expertise, which not every lab may possess.
Moreover, the ambiguity often surrounding variant effects can add another layer of complexity. The interpretation of single nucleotide polymorphisms (SNPs) or indels usually requires a comprehensive understanding of genetic pathways and population genetics. Researchers might find themselves grappling with terms like "benign," "pathogenic," or "uncertain significance." This can not only slow down the research timelines but also lead to frustration among teams trying to push forward with their work.
"The complexities of genomic data can often feel like a riddle wrapped in a mystery inside an enigma." – A common sentiment shared by researchers.
"The complexities of genomic data can often feel like a riddle wrapped in a mystery inside an enigma." – A common sentiment shared by researchers.
The reliance on reference genomes poses another barrier as well. If researchers use an outdated or poorly annotated reference genome, they might miss key variants or misinterpret their significance. The implementation of robust bioinformatics pipelines is imperative, but even then, human oversight is required to ensure that the results are reliable and applicable.
Technical Limitations of Technology
The technical limitations of Illumina technology are noteworthy, as even industry standards can falter at times. First and foremost is the issue of sequencing errors. While the overall error rate is relatively low, the presence of systematic errors means that what appears to be a significant variant may sometimes be a mere artifact of the sequencing process. Such errors can arise from various factors, including PCR amplification biases or the inherent limitations of the sequencing chemistry itself.
Another technical hurdle is the read length. Illumina sequencing typically yields shorter reads in comparison to some other platforms like Pacific Biosciences or Oxford Nanopore. This can complicate the assembly of complex regions of the genome, especially repetitive areas, which are notoriously hard to sequence accurately. An incomplete or incorrect assembly can lead to gaps in the data, drastically affecting downstream analyses.
Lastly, the high cost of instrumentation and the need for specialized training in bioinformatics present significant barriers for some labs, limiting the accessibility of cutting-edge genomic research. Moreover, as genomic technologies advance, keeping pace with updates and improvements can feel like a race against time, leaving some researchers scrambling just to catch up.
In summation, navigating the challenges and limitations associated with Illumina analysis requires both technical expertise and critical thinking. Addressing these factors head-on fosters a deeper understanding of the capabilities and constraints of this powerful sequencing technology, ultimately leading to more nuanced and accurate genomic research.
Future Perspectives
The field of genomics is witnessing dynamic transformations, largely propelled by advancements in Illumina technology. These emerging possibilities shape not only research methods but also practical applications that influence healthcare, agriculture, and beyond. A deep dive into innovations within sequencing technology and the integration of diverse omics data elucidates the crucial elements paving the way for future breakthroughs.
Innovations in Sequencing Technology
Sequencing technologies are evolving at a breakneck pace. With each iteration, we see enhancements that address prior limitations while pushing the boundary of what is possible in genetic research. For instance, the recent introduction of high-throughput sequencing solutions allows researchers to process vast datasets in significantly reduced timeframes.
Here are a few noteworthy advancements:
- Single-cell sequencing: Offers insights into cellular heterogeneity, crucial for understanding diseases like cancer at a granular level.
- Long-read sequencing: Unlike traditional methods, this enables more comprehensive genomic analyses by providing longer continuous sequences that capture structural variations more effectively.
- Real-time sequencing: Technologies such as Oxford Nanopore provide the advantage of obtaining sequencing data on-the-fly, facilitating immediate analysis and decision-making.
Such innovations are not just about speed; they play an essential role in improving accuracy and reproducibility in genomic studies. The implications of this could be monumental, giving rise to new therapies and intervention strategies across various disciplines.
"In the genomic era, while depth and speed contribute significantly to understanding the molecular underpinnings of diseases, it’s the accessibility of these advancements that holds the key for real change."
"In the genomic era, while depth and speed contribute significantly to understanding the molecular underpinnings of diseases, it’s the accessibility of these advancements that holds the key for real change."
Integration with Other Omics Data
The genomic landscape is multifaceted, comprising various layers of biological information. The integration of genetics, transcriptomics, proteomics, and metabolomics is becoming a critical aspect of modern biological research. By synthesizing these diverse data types, researchers can achieve a holistic view of biological systems, enriching their interpretations and paving the way for more comprehensive insights.
A few considerations include:
- Holistic Understanding: Combining data enables a multi-dimensional perspective, crucial for deciphering complex biological phenomena like disease pathways.
- Enhanced Precision Medicine: Integrating genomic and proteomic data can pinpoint specific biomarkers for tailored treatment strategies, advancing personalized medicine.
- Efficient Resource Allocation: Understanding how different omics interact allows for better-targeted research funding and policy decisions.
This integrative approach, while offering advantages, does come with its challenges. The complexity of data integration necessitates advanced analytical pipelines and robust data-sharing frameworks. Therefore, researchers must prioritize developing tools that facilitate smooth transitions between disparate datasets.
Epilogue
In the closing section of this article, we reflect on the essential contribution that Illumina analysis offers to the field of genomic research. It is quite clear that the methodologies and technologies discussed have fundamentally altered how scientists approach genetic inquiry. The ambitious aspirations and rigorous realities of genomic analysis are neatly sewn together, creating a textile of comprehensive understanding and practical application.
Recap of Key Points
To summarize, key elements of Illumina analysis include:
- Next-Generation Sequencing (NGS): This has revolutionized the way we sequence genetic material, bringing speed and efficiency to the forefront.
- Data Processing: From quality control measures to alignment strategies, the journey from raw data to usable information is critically detailed.
- Applications in Various Fields: Illumina technology finds its usefulness in many domains, ranging from clinical settings to population genomics and personalized medicine.
- Future Directions: Innovations and the integration of various omics data hint that the field is just at the beginning of a transformative era.
These discussions highlight how the intricate processes of Illumina sequencing, the significance of rigorous data interpretation, and their impact on various scientific disciplines collectively shape the landscape of genomics today.
Call for Continued Research
Recognizing the advancements made with Illumina analysis is crucial, but it is equally important to underscore the need for ongoing exploration in this arena. As breakthroughs in sequencing technology emerge, we must remain vigilant in integrating these new tools with existing methodologies. Encouraging interdisciplinary collaboration will further enrich understanding and mitigate challenges faced in the field.
"As the genomic landscape evolves, so must our techniques and approaches, ensuring that we harness these developments to their fullest potential for both research and practical applications."
"As the genomic landscape evolves, so must our techniques and approaches, ensuring that we harness these developments to their fullest potential for both research and practical applications."
Continued research efforts should focus not only on refining existing methodologies but also on addressing the limitations discussed within this article—such as data interpretation difficulties and technical constraints. Engaging with the scientific community, funding new studies, and fostering educational initiatives will be essential as we push the boundaries of what is possible in genomics.