Innovations in High Energy Density Batteries of 2021
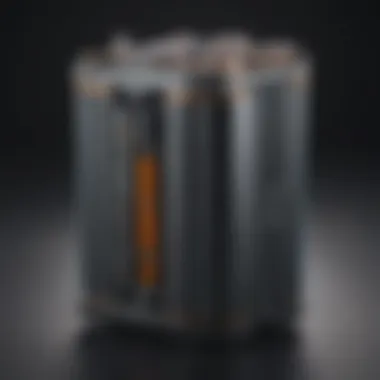
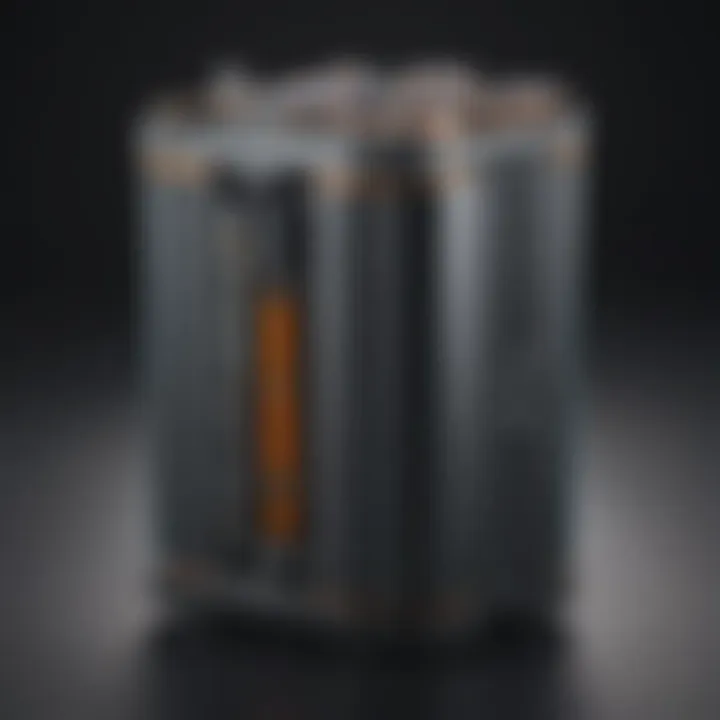
Intro
The evolution of battery technology is crucial for the future of many sectors, particularly renewable energy and electric transportation. In 2021, the focus on energy density was more pronounced than ever. As global demand for efficient energy storage solutions grows, innovations have emerged that promise to reshape how we think about energy. This article seeks to delve into the significant advancements in battery technologies during this period, exploring their fundamental principles and applications.
Understanding energy density is not merely an exercise in technical jargon; it has real-world implications. It affects everything from the range of electric vehicles to the efficiency of renewable energy storage systems. By analyzing recent breakthroughs, we hope to highlight not just the potential these batteries hold, but also the challenges and considerations that come with their use.
Key Concepts
Definition of the Main Idea
Energy density refers to the amount of energy stored in a given system or region of space per unit volume or mass. It is a critical factor influencing the performance of batteries. Higher energy density typically translates into smaller, lighter batteries that can store more electricity. This has profound implications for applications like electric cars and renewable energy integration.
Overview of Scientific Principles
Several scientific principles underpin energy density.
- Chemical Battery Reactions: These reactions define how energy is stored and released. Different materials yield different performance characteristics.
- Materials Science: The choice of components, such as cathodes and anodes, directly impacts energy density. Lithium-ion technology, for instance, has been dominant due to its relatively high energy density.
- Thermodynamics: This plays a part in understanding energy losses during charging and discharging cycles.
By grasping these concepts, we can better appreciate the advancements made in 2021.
Current Research Trends
Recent Studies and Findings
Numerous research projects have focused on enhancing energy density. Scientists have explored alternative materials, such as solid-state batteries, which promise greater energy density and safety. Notably, research has pointed towards lithium-sulfur and lithium-air batteries as candidates for the future, boasting potential energy densities much higher than traditional lithium-ion systems.
Significant Breakthroughs in the Field
Some standout breakthroughs in 2021 include:
- Solid-State Batteries: These batteries use solid electrolytes, contributing to safety and potentially higher density. Companies like QuantumScape are at the forefront of this technology.
- Silicon Anodes: Replacing traditional graphite with silicon has shown promise in enhancing energy capacity.
- Hybrid Systems: Innovations integrating multiple technologies to optimize performance and energy storage.
"Advancements in battery technology are not just about performance; they are about enabling a sustainable future."
"Advancements in battery technology are not just about performance; they are about enabling a sustainable future."
Understanding Energy Density
Energy density is a crucial aspect of battery technology that directly affects the performance and applicability of various devices. This section aims to dissect the concept of energy density and how it underpins advancements in battery innovations of 2021. Understanding energy density involves recognition of its types, definitions and the significant role it plays in enhancing the efficiency of energy storage systems. As the demand for longer-lasting batteries escalates, having a solid grasp of energy density becomes essential for both researchers and consumers. Not only does it influence battery design, but it also impacts industry goals related to sustainability and energy consumption.
Definition of Energy Density
Energy density refers to the amount of energy stored per unit of volume or mass. This metric determines how much energy a battery can deliver, and it is essential in defining the overall performance and capabilities of batteries. The higher the energy density, the more energy a battery can hold, which directly affects how long it can power a device. Understanding this definition allows one to assess how energy density evolves in different battery technologies.
Types of Energy Density
In battery technology, energy density can be evaluated in two main ways: gravimetric energy density and volumetric energy density. Each has its distinct implications and benefits.
Gravimetric Energy Density
Gravimetric energy density is defined as the amount of energy stored per unit mass. It is a critical characteristic because it affects the weight of the batteries, which is especially important for portable applications. A key advantage of high gravimetric energy density is that it can lead to lighter and more efficient energy storage solutions, making it a popular choice in fields like electric vehicles and consumer electronics. However, it may trade off some volume efficiency, which is crucial in devices where space is limited. Thus, optimizing gravimetric energy density is often a balancing act with volumetric considerations.
Volumetric Energy Density
On the other hand, volumetric energy density measures the amount of energy stored per unit volume. This aspect of energy density is vital for applications where space constraints are predominant, such as in mobile devices or spacecraft. A notable characteristic of volumetric energy density is that it often allows for compact battery designs, enhancing overall design flexibility. One unique feature is that while volumetric energy density can improve energy storage in smaller units, it can also create challenges when aiming for weight reductions. This can be a trade-off, especially when considering future designs aimed at higher performance.
Importance in Battery Technology
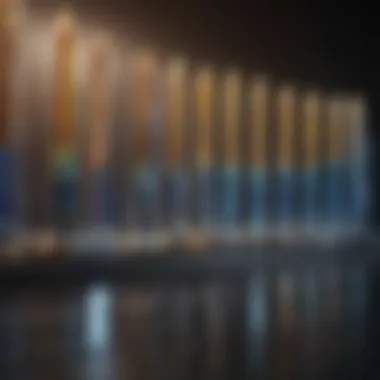
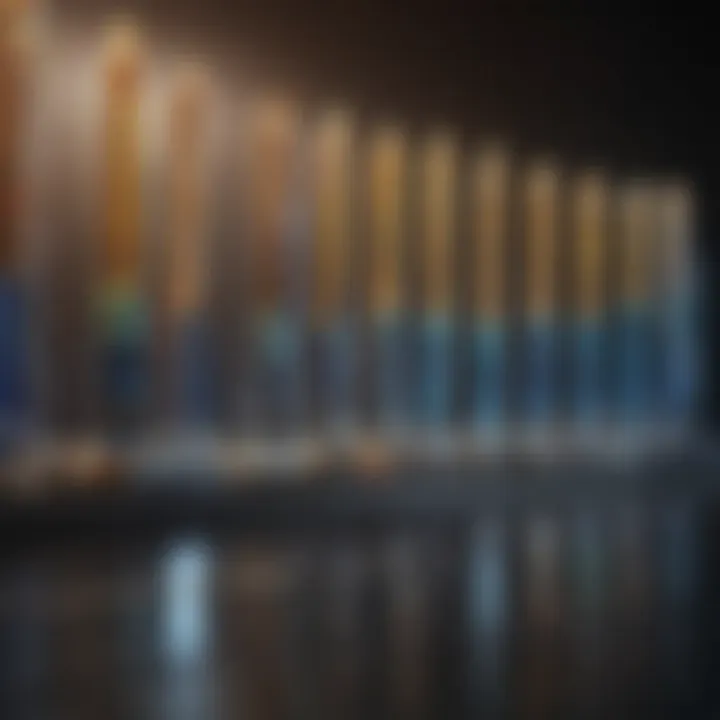
The significance of energy density in battery technology cannot be overstated. It not only influences battery performance but also dictates the feasibility of integrating new technologies into mainstream applications. High energy density batteries can facilitate significant improvements in various sectors, including renewable energy, autonomous systems, and electric mobility. Stakeholders must consider energy density when developing novel battery technologies to ensure that they meet the performance demands of a rapidly evolving market. In summary, understanding energy density provides valuable insights into the dynamics of battery performance and application possibilities.
Current Landscape of Battery Technologies
The current landscape of battery technologies is crucial for understanding the advancements made in energy density within a competitive market. The evolution of battery types affects not only performance but also cost, safety, and environmental impacts. As the demand for high-energy and efficient storage solutions increases across various sectors, the importance of recognizing the different technologies becomes apparent. This section will provide a comprehensive overview of the most prominent battery types currently in use while also considering their energy densities and applications.
Overview of Battery Types
Lithium-ion Batteries
Lithium-ion batteries are perhaps the most prevalent type of battery used today. Their significance lies in their high energy density, which is typically around 150-250 Wh/kg, making them suitable for portable electronics and electric vehicles. Their key characteristic is the ability to charge quickly and hold a charge for an extended period. This efficiency is beneficial, especially in applications where space and weight are critical considerations.
A unique feature of lithium-ion batteries is their electrochemical cell design, which allows them to be lightweight and compact. However, they do come with drawbacks, such as limited lifespan and potential safety risks under certain conditions. Nonetheless, they remain a cornerstone in the battery technology landscape due to their balance of performance and cost-effectiveness.
Solid-state Batteries
Solid-state batteries represent a significant innovation in battery technology. Their contribution to the landscape lies in their supposed advantages over traditional lithium-ion systems, particularly in safety and energy density. The solid electrolytes used in these batteries provide better thermal stability and a reduced risk of leakage, making them a promising option for future applications in electric vehicles and other high-performance equipment.
A key characteristic of solid-state batteries is their potential for much higher energy densities, estimated to reach up to 500 Wh/kg. This makes them advantageous for applications needing long ranges and quick charging times. However, manufacturing challenges and high production costs remain obstacles that must be addressed before widespread adoption occurs.
Flow Batteries
Flow batteries offer a different approach to energy storage that emphasizes scalability and longevity. These batteries store energy in liquid electrolytes that flow through a cell. Their distinct structure allows for the easy scaling of energy capacity by increasing the size of the storage tanks. This makes flow batteries particularly suitable for renewable energy storage applications, such as solar and wind energy integration.
The primary attribute of flow batteries is their ability to discharge continuously, providing power for extended periods, which is a significant benefit for grid energy storage. However, they tend to have lower energy densities compared to lithium-ion and solid-state alternatives, which can limit their immediate applications. Understanding these differences is essential for evaluating energy storage systems in various scenarios.
Comparative Analysis of Energy Densities
The comparative analysis of energy densities among various battery types highlights the ongoing evolution in the quest to enhance battery performance. By evaluating how lithium-ion, solid-state, and flow batteries measure up against each other, a clearer picture emerges that outlines their relative advantages and limitations in practical applications.
Significant Innovations in
The year 2021 has marked significant leaps in battery technology, especially concerning high energy density batteries. These innovations play a crucial role in shaping the future of various applications. From electric vehicles to consumer electronics, the advancements made can potentially redefine performance standards and establish new benchmarks. Companies and researchers have focused on enhancing materials and technologies in the quest for improving energy storage efficiency. This section dives into key advancements that have emerged, showcasing unique contributions to the field of battery innovation.
Advancements in Lithium-ion Batteries
New Cathode Materials
New cathode materials have gained considerable attention for their impact on the energy capabilities of lithium-ion batteries. Innovations such as nickel-rich materials and lithium iron phosphate are at the forefront. These cathodes can offer improved energy density and cycle stability.
A key characteristic is their ability to enhance the total energy storage per cell. This is particularly beneficial for applications requiring long-lasting power. The unique feature of these materials can often result in increased energy retention. However, they may pose challenges like higher costs and resource availability.
Enhanced Anode Designs
Enhanced anode designs are also important in maximizing battery performance. Researchers have been exploring silicon-based anodes as a replacement for traditional graphite. Silicon anodes provide several benefits, such as increased specific capacity and improved efficiency.
The primary characteristic is their ability to store more lithium ions, resulting in higher energy density. Although promising, these designs face issues such as volume expansion during charge cycles, which can affect battery longevity. Finding ways to mitigate these challenges is key for manufacturing and market adoption.
Emergence of Solid-state Technologies
Electrolyte Innovations
Solid-state technology centers around the development of new electrolytes. These innovations help transition from liquid electrolytes in conventional batteries to solid forms, enhancing safety and performance. New materials, like sulfide-based electrolytes, show considerable promise.
A critical aspect is their high ionic conductivity, which facilitates better energy transfer. This leads to higher efficiency in energy use, allowing devices to run longer. However, production scalability remains a concern. Achieving a balance between performance and manufacturability is crucial for future applications.
Manufacturing Techniques
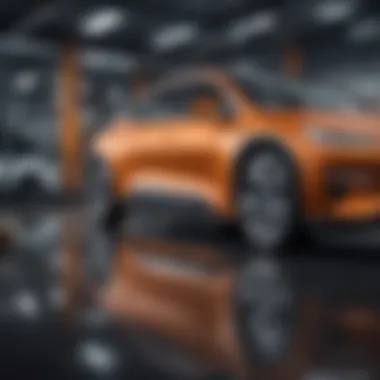
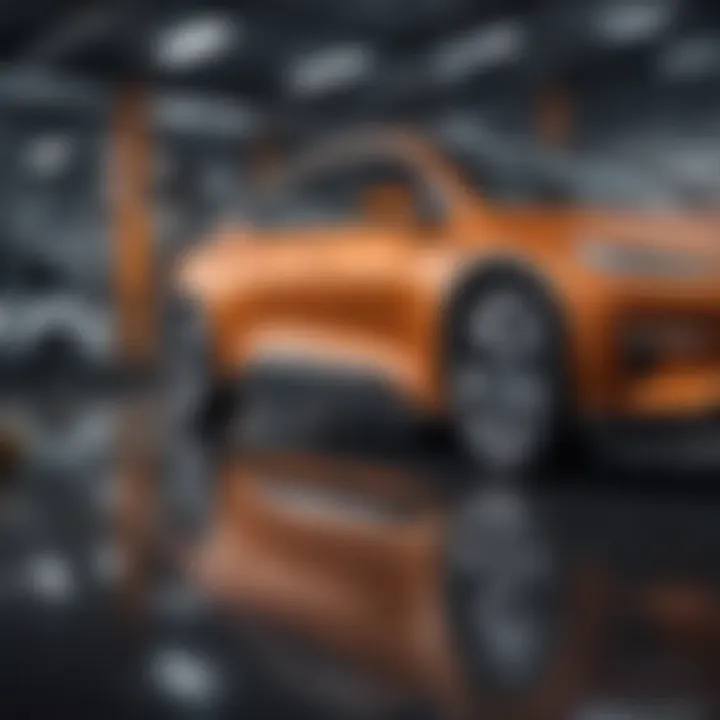
The manufacturing techniques for solid-state batteries have also advanced. Processes like additive manufacturing and roll-to-roll production are gaining traction. These methods can lower production costs while maintaining battery performance.
The ability to mass-produce solid-state batteries effectively makes them appealing for large-scale applications. Yet, the scalability and integration into the existing supply chain will need ongoing attention.
Breakthroughs in Alternative Chemistries
Sodium-ion Batteries
Sodium-ion batteries present a compelling alternative to traditional lithium-ion batteries. They utilize sodium as a charge carrier, making them potentially cheaper due to the abundance of sodium resources. A key characteristic is their ability to perform under different temperature conditions, which opens the door for diverse applications.
While they offer advantages in terms of material availability, they generally exhibit lower energy density compared to lithium-ion counterparts. This may limit their effectiveness in some high-performance applications.
Lithium-Sulfur Batteries
Lithium-sulfur batteries have gained traction for their high theoretical energy density. This chemistry allows for the integration of sulfur as the cathode material. A prominent feature is the potential to significantly increase the capacity compared to conventional lithium-based systems.
The attractiveness lies in their capability to be more environmentally friendly. Nevertheless, technical challenges persist related to cycle stability and energy retention. Finding solutions to these issues continues to be the focus of research efforts in 2021.
"Innovations in battery technology could potentially reshape the fields of renewable energy and electric vehicles, offering a glimpse into a more sustainable future."
"Innovations in battery technology could potentially reshape the fields of renewable energy and electric vehicles, offering a glimpse into a more sustainable future."
The advancements discussed are central to understanding the evolution of energy storage technologies. As these innovations continue to develop, they hold the potential to transform how we interact with energy in both daily life and industrial applications.
Applications of High Energy Density Batteries
The importance of high energy density batteries extends across several sectors, each influenced by unique requirements and specifications. These batteries provide distinct advantages, such as improved performance and efficiency. Their applications range from electric vehicles to renewable energy storage solutions and consumer electronics. Understanding these applications is crucial for grasping the benefits and considerations involved in implementing these battery technologies.
Electric Vehicles
Electric vehicles (EVs) have gained significant traction in recent years, driven by the pursuit of sustainable transportation. High energy density batteries play a critical role in enhancing the effectiveness of EVs.
Range Improvements
Range improvements are a fundamental focus in electric vehicle design. High energy density batteries allow vehicles to travel further on a single charge. This feature is essential for reducing range anxiety among users. Enhanced range not only makes electric vehicles more appealing to consumers but also contributes to their market growth. With improvements, vehicles can cover distances comparable to traditional fossil-fuel cars, making them more viable. However, the cost associated with producing batteries that achieve this level of range remains a challenge.
Performance Metrics
Performance metrics in electric vehicles include acceleration, efficiency, and charging speed. High energy density batteries contribute to these metrics significantly. By providing increased power output, they enable quicker acceleration and thus, more enjoyable driving experiences. Moreover, these batteries tend to support faster charging times, which is appealing for consumers. Yet, while they enhance performance, maintaining a balance between energy density and safety can complicate battery design and development.
Renewable Energy Storage
Renewable energy sources like solar and wind have unique requirements for efficient energy use. High energy density batteries present opportunities for better energy storage.
Solar Energy Applications
Solar energy applications are integral to many renewable energy solutions. High energy density batteries allow for storing excess energy generated during peak sunlight hours. This stored energy can then be used during low production periods, such as nighttime. The ability to harness solar energy effectively reduces dependency on non-renewable resources. However, the initial investment and installation costs can deter some users from fully embracing these technologies.
Wind Energy Integration
Wind energy integration benefits from high energy density batteries, which can store energy generated during high wind periods. This consistent storage capability helps balance supply and demand, effectively integrating wind energy into the broader power grid. The characteristic of flexibility allows utilities to use energy when itβs most needed. Yet, the variability in wind energy production necessitates careful planning in battery capacity and placement to maximize efficiency.
Consumer Electronics
The rise of portable electronic devices highlights the importance of high energy density batteries. Devices such as smartphones, tablets, and laptops can greatly benefit from advancements in battery technology.
High energy density batteries enhance the usability of these devices by allowing longer usage times with shorter charging periods. Consumers today expect devices that not only perform well but also last throughout the day without needing frequent charges. The pursuit of these expectations has led to increased investments in battery technologies that meet these requirements but may drive up device costs as a byproduct.
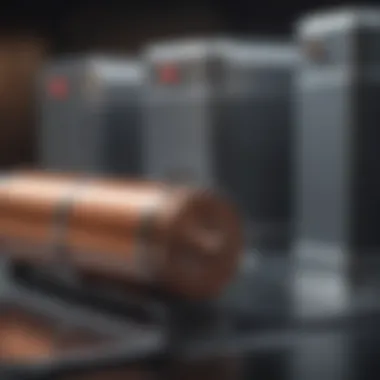
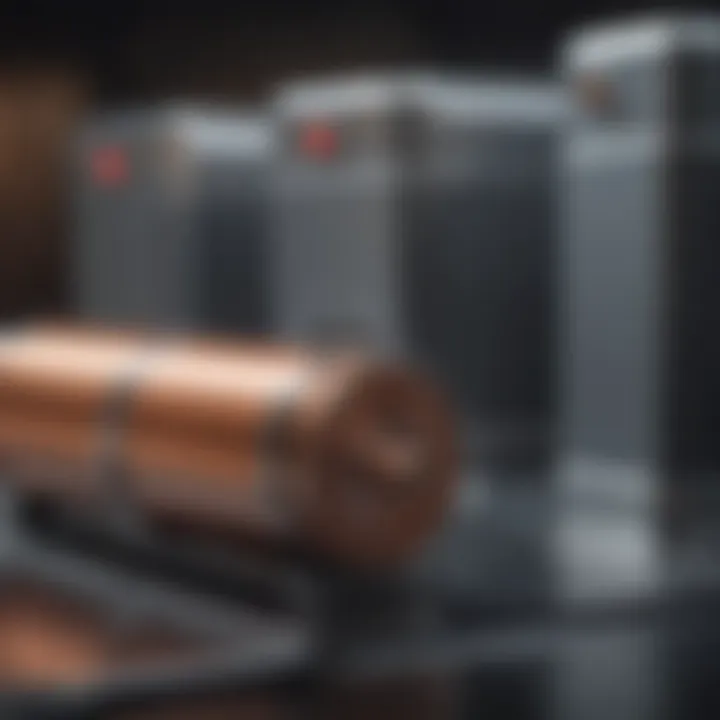
High energy density batteries form the backbone of many advancements in technology, shaping how we think about energy, efficiency, and sustainability.
High energy density batteries form the backbone of many advancements in technology, shaping how we think about energy, efficiency, and sustainability.
Challenges Facing New Battery Technologies
The rapid advancements in battery technology have not come without significant challenges. As we observe innovations in high energy density batteries, it is essential to acknowledge the hurdles that researchers and manufacturers need to overcome. These challenges affect not only the feasibility of these technologies in commercial applications but also their long-term viability in meeting global energy needs.
Cost Implications
One of the most pressing challenges facing new battery technologies is the cost associated with their development and production. High energy density batteries often rely on rare materials that are expensive to source. For instance, manufacturers of lithium-sulfur batteries need specialized materials for their production. The cost of these materials can limit market adoption. Moreover, the overall manufacturing process can be resource-intensive, further driving up prices.
Reducing these costs is critical if we want high energy density batteries to compete with more traditional options. The economic viability of innovations hinges on finding alternative components that are both effective and affordable.
Scalability Issues
Scalability remains a significant concern as well. While a small-scale laboratory can successfully produce cutting-edge batteries, translating that success into mass production presents complexities. The transition from laboratory to industrial scale often reveals weaknesses in design or operational logistics.
Another aspect of scalability is the infrastructure needed for production. Companies may find that existing manufacturing setups are not suited for new battery technologies. This lack of readiness can slow the deployment of promising advancements, preventing them from meeting the growing energy needs, especially in sectors like electric vehicles and renewable energy storage.
Safety Concerns
Safety is perhaps the most critical challenge facing new battery technologies. High energy density batteries, like lithium-ion cells, have been known to pose fire risks under specific conditions. As manufacturers develop batteries with higher capacities and different chemistries, the potential for safety issues must be addressed.
The safety considerations include thermal stability, chemical reactivity, and failure modes of batteries under stress. Any new technology must undergo rigorous testing to ensure it can operate safely in all expected conditions. Failure to adequately address safety can lead to devastating consequences that stifle public confidence in these innovations.
"As energy storage continues to evolve, addressing cost, scalability, and safety challenges will determine the successful integration of new battery technologies into mainstream applications."
"As energy storage continues to evolve, addressing cost, scalability, and safety challenges will determine the successful integration of new battery technologies into mainstream applications."
Future Directions in Battery Research
The field of battery research is in a state of rapid evolution. Understanding future directions in battery research is crucial for advancing energy density technologies. Innovations not only address current limitations but also pave the way for sustainable energy solutions. This section will discuss potential materials for next-generation batteries, advancements in recycling technologies, and regulatory and environmental considerations. Each element is vital for ensuring that these products are both effective and safe in their integrated systems.
Potential Materials for Next-gen Batteries
Research in battery technologies often revolves around finding better materials that enhance properties like energy density, cycle life, and safety.
Materials such as lithium-sulfur or sodium-ion are gaining attention. Lithium-sulfur batteries can theoretically achieve much higher energy density than traditional lithium-ion counterparts. Sulfur is abundant and inexpensive, making it an attractive option. Sodium-ion batteries share this advantage, utilizing sodium, which is more plentiful than lithium.
Additionally, materials like graphene and solid electrolytes show promise. Graphene offers high conductivity and can enable faster charge times. Solid electrolytes can improve the safety profile of batteries, reducing risks like leakage or fires that are often associated with liquid electrolytes.
Researching these new materials allows for a broader application in sectors such as electric vehicles and portable electronics.
Advancements in Recycling Technologies
As the production and disposal of batteries become increasingly scrutinized, advancements in recycling technologies play a critical role. Efficient recycling can mitigate environmental impacts by recovering precious materials, thus lessening the demand for new resources.
Methods such as hydrometallurgical processes are being developed to extract valuable metals from used batteries. These processes use aqueous solutions to leach metals even from complex compounds without significant environmental harm. Similarly, pyrometallurgical approaches, which involve high-temperature treatment, can be used alongside newer technologies to maximize material recovery.
Innovations in recycling are crucial, especially as electric vehicle adoption rises, leading to a surge in end-of-life vehicle batteries. Establishing effective recycling processes is essential to ensure sustainability and reduce waste.
Regulatory and Environmental Considerations
With innovations in battery technologies, regulatory frameworks become necessary to ensure safety and viability. Environmental considerations are significant too. Regulatory bodies are starting to implement stricter guidelines around battery production, usage, and disposal.
Policies must reflect the full lifecycle of batteries. This includes manufacturing processes, transportation, and end-of-life management. Understanding the regulatory landscape helps organizations align with best practices. It can also drive investments in cleaner production methods.
Furthermore, there is growing emphasis on the use of environmentally friendly materials and processes. Companies that invest in eco-friendly technologies may gain a competitive edge in markets increasingly focused on sustainability.
"The future of battery technology lies in innovation coupled with sustainability. This perspective can determine the direction and success of energy storage solutions in the coming years."
"The future of battery technology lies in innovation coupled with sustainability. This perspective can determine the direction and success of energy storage solutions in the coming years."
In summary, the future directions in battery research point towards materials that promise higher energy densities alongside responsible recycling practices and regulatory compliance. These elements are essential for fostering a sustainable transition to renewable energy and efficient electric transportation.
Their proper integration can allow societies to harness the full potential of battery innovations as they continue to evolve.