Innovative Mechanisms in CO2 Electrochemical Reduction
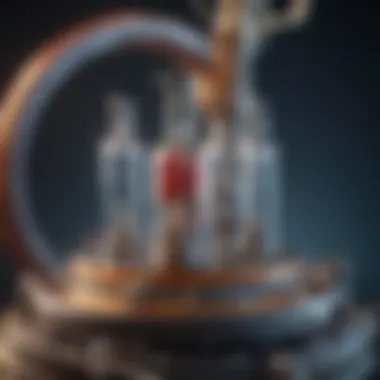
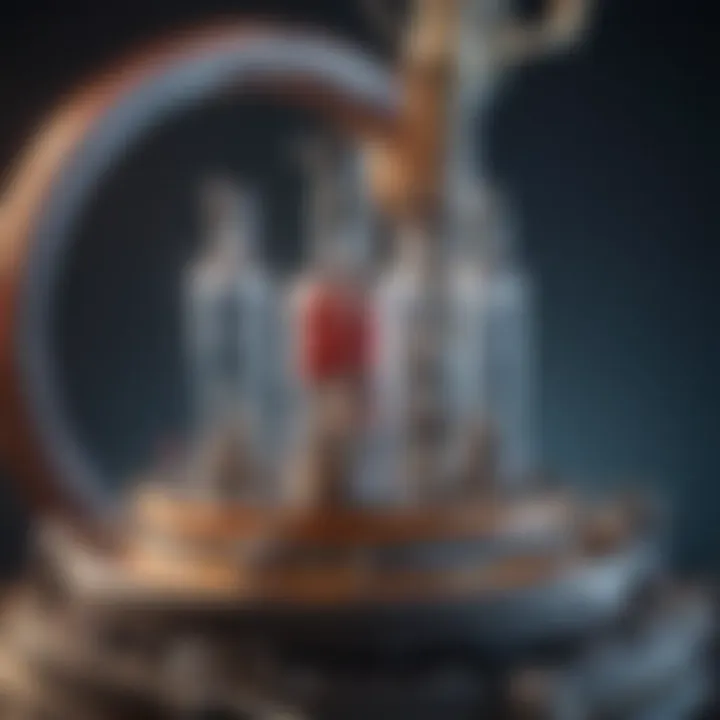
Intro
The electrochemical reduction of carbon dioxide (CO2) has come to the forefront of research aimed at addressing climate change. This process not only helps in mitigating the effects of greenhouse gas emissions but also holds promise as a sustainable energy solution. In this article, we will delve into the mechanisms behind electrochemical reduction, the catalysts involved, and the significance of product selectivity. Additionally, we will explore recent innovations that enhance efficiency and discuss future challenges that may arise in this evolving field.
Key Concepts
Definition of the Main Idea
Electrochemical reduction of CO2 refers to the conversion of carbon dioxide into usable fuels or chemicals through electrochemical reactions. This process is particularly appealing as it can utilize renewable energy sources, making it a key player in advancing sustainable energy initiatives. The aim is to produce substances like carbon monoxide, methanol, or hydrocarbons, which can then serve as energy sources or raw materials.
Overview of Scientific Principles
To fully understand electrochemical reduction, one must consider the fundamental principles governing electrochemical reactions. These reactions involve the transfer of electrons, and they occur within an electrolytic cell. The cell comprises an anode, where oxidation occurs, and a cathode, where reduction takes place. Catalysts play a crucial role in these reactions by lowering the energy barriers, thus enhancing the overall efficiency of CO2 reduction. The choice of electrolyte also affects the reaction dynamics, influencing both the rate and selectivity of the products formed.
"Electrochemical reduction of CO2 is a critical technological pathway that could significantly change energy conversion and storage methods in the coming decades."
"Electrochemical reduction of CO2 is a critical technological pathway that could significantly change energy conversion and storage methods in the coming decades."
Current Research Trends
Recent Studies and Findings
Research in the electrochemical reduction of CO2 has accelerated in recent years. Numerous studies focus on improving the efficacy of catalysts, with advanced materials being tested continuously. Recent developments include the use of transition metal compounds and organic materials that have demonstrated enhanced activity and selectivity for specific products.
Significant Breakthroughs in the Field
Significant breakthroughs in thermodynamic understanding have emerged, granting insights into optimizing reaction conditions. For instance, researchers have managed to stabilize reactive intermediates that often hinder the reduction process. Moreover, the integration of artificial intelligence in process optimization is showing potential, allowing for rapid discovery and deployment of new catalytic systems.
With the growing urgency surrounding climate change, understanding the intricacies of CO2 reduction is more important than ever. By synthesizing current knowledge and innovations, we can better prepare for the challenges that lie ahead in achieving a sustainable future.
Prelude to Electrochemical Reduction of CO2
Electrochemical reduction of carbon dioxide is an essential topic in the context of climate change mitigation and sustainable energy. This process works by converting CO2 into usable chemicals, which can directly contribute to decreasing atmospheric CO2 levels. The significance of this reduction cannot be overstated, as it aligns with global goals to achieve carbon neutrality. Understanding the mechanisms involved provides a pathway for innovative advancements in technology and energy production.
Definition and Importance
Electrochemical reduction refers to the process where an electric current drives the conversion of chemical species. In this case, it means using electricity to transform carbon dioxide into hydrocarbons or other useful chemicals. This technology offers potential advantages that distinguish it from traditional CO2 management techniques. First, it promotes a circular carbon economy by recycling CO2, thus reducing dependency on fossil fuels.
Moreover, the ability to convert CO2 into valuable products can create new economic opportunities. This can enhance the practicality of renewable energy systems, which is vital in the transition. Furthermore, electrochemical strategies, when coupled with renewable electricity sources, can significantly lower greenhouse gas emissions.
The growing importance of this field drives research, requiring a clear grasp of the underlying electrochemical principles and the role of catalysts. Scientists are continuously seeking effective materials that boost the efficiency of this conversion process, and this exploration leads to innovations that might change energy dynamics.
Historical Context
The journey of CO2 electrochemical reduction began in the late 20th century. Initially focused on fundamental electrochemistry, early research aimed to understand how CO2 behaves in electrochemical systems. However, it was not until the early 2000s that the field gained momentum. The rising awareness of climate change issues led to increased investment and scholarly attention in the mechanisms of CO2 conversion.
Key developments occurred in catalysis, where researchers identified various materials with promising efficiencies. Studies showed that certain metals, like copper, have unique properties allowing for the selective production of carbon-based compounds from CO2.
The past decade has witnessed rapid advancements and growing interest from both academia and industry, emphasizing the potential of this technology to mitigate climate change. As the world moves towards achieving the Paris Agreement targets, the historical momentum of CO2 electroreduction becomes not only an academic exercise but also a fundamental element in environmental strategy.
Fundamental Principles of Electrochemical Reactions
The field of electrochemical reactions plays a crucial role in the electrochemical reduction of carbon dioxide. Understanding the fundamental principles helps to shed light on how CO2 can be transformed into useful products. This section explores two key elements: electrolytic cells and redox reactions. The insights gained here are essential for grasping the techniques that drive the reduction process.
Electrolytic Cells
Electrolytic cells are devices that facilitate the conversion of electrical energy into chemical energy through electrolysis. In the context of CO2 reduction, these cells enable the conversion of carbon dioxide into various hydrocarbons and alcohols. An electrolytic cell consists of two electrodes: the anode and the cathode, which are submerged in an electrolyte solution.
The importance of electrolytic cells in CO2 reduction can not be overstated. They create the necessary conditions for the redox reactions to occur, allowing for efficient energy transfer. The choice of electrolyte impacts the cell's performance and the efficiency of the reduction process. For example,
- Water is often used in some systems as a solvent.
- Ionic liquids can also provide a stable environment.
Understanding the design and function of electrolytic cells is essential for researchers and practitioners working in the field of electrochemical CO2 reduction. They must consider factors like ion transport, resistance, and the stability of the components to optimize the entire setup.
Redox Reactions
Redox reactions, short for reduction-oxidation reactions, serve as the backbone for electrochemical processes, including CO2 reduction. These reactions involve the transfer of electrons between two species. During CO2 reduction, the carbon dioxide molecule is reduced while another species, often water, is oxidized.
In this reaction, electrons are transferred from the anode to the cathode. The overall efficiency of the process hinges on several factors: - The electron sources must be readily available. - The kinetics of the reaction must favor fast electron transfer. - The arrangement and choice of catalysts can significantly influence the redox pathways.
The mechanisms underlying these reactions dictate the product selectivity and yield, making it vital for ongoing research to optimize conditions for desired products.
"Redox reactions are the driving force of many electrochemical processes, including the shift from carbon dioxide to valuable chemicals and fuels."
"Redox reactions are the driving force of many electrochemical processes, including the shift from carbon dioxide to valuable chemicals and fuels."
As a whole, the principles of electrolytic cells and redox reactions form a foundation upon which the electrochemical reduction of CO2 can be analyzed and improved. Understanding these principles is crucial for anyone involved in advancing sustainable energy solutions through the manipulation of carbon dioxide.
Mechanisms of CO2 Reduction
Understanding the mechanisms of CO2 reduction is crucial for advancing the efficiency and practicality of this transformative technology. This process entails converting carbon dioxide into useful products, hence supporting efforts towards sustainable energy and emission reduction. It comprises multiple pathways which involve various reactions under electrochemical conditions. Identifying these pathways allows researchers to optimize the parameters that influence the overall performance of the reduction process.
The significance of understanding the mechanisms goes beyond just the reactions themselves. It allows for the discovery of specific catalysts and operating conditions that maximize reaction efficiency. Moreover, a deeper insight into the mechanisms can aid in predicting product selectivity, which is essential for tailoring outputs for desired applications.
"Mechanisms of CO2 reduction are foundational to the development of viable technologies for sustainable energy conversion."
"Mechanisms of CO2 reduction are foundational to the development of viable technologies for sustainable energy conversion."
Electrocatalytic Pathways
Electrocatalytic pathways describe the detailed steps that occur during the electrochemical reduction of CO2. These pathways involve the transfer of electrons and protons to CO2 molecules, which ultimately leads to the formation of a variety of products like carbon monoxide, methane, and other hydrocarbons. The nature of the cathode material significantly influences these pathways.
Different materials can lead to differing pathways, which results in variations in selectivity towards certain products. Transition metals and metal oxides are commonly utilized as electrocatalysts due to their ability to effectively facilitate electron transfer.
Factors affecting the kinetics of these pathways include:
- Choice of Electrocatalyst: The active sites on the catalyst play a pivotal role in determining the efficiency of electron transfer.
- Electrolyte Composition: The type and concentration of electrolytes can greatly influence the reaction environment, subsequently impacting the pathways.
- Potential Applied: The voltage applied during the reaction can change the pathway dynamics substantially, thus affecting product yield and selectivity.
Formation of Intermediates
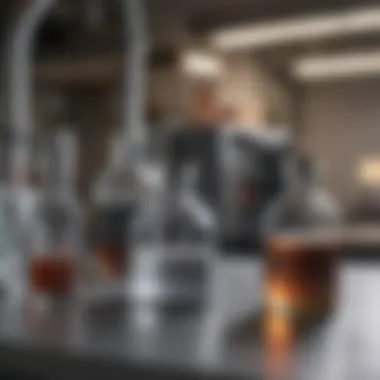
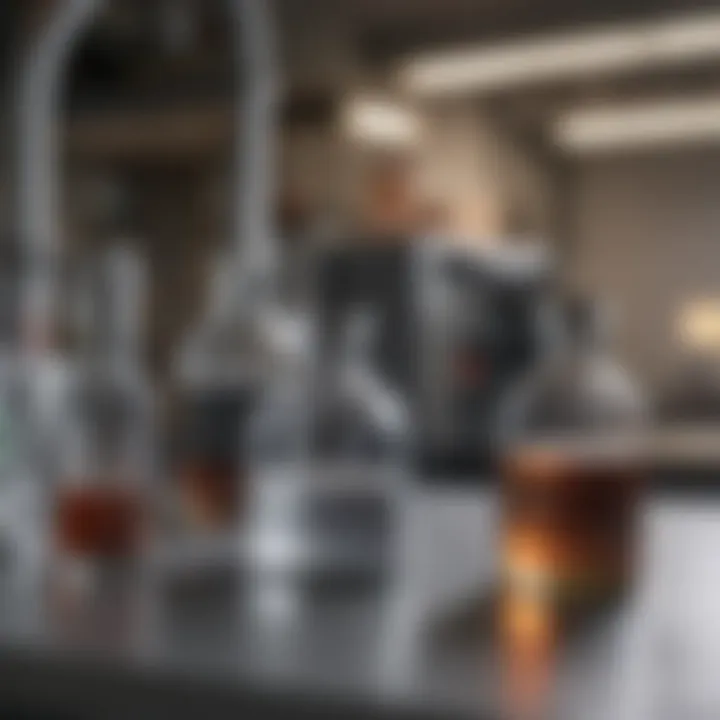
Formation of intermediates is another critical aspect of the CO2 reduction mechanisms. Intermediates are transient species formed during the conversion of CO2 into end products. Their stability and reactivity directly affect the efficiency and selectivity of the electrochemical process.
Key intermediates in CO2 reduction include:
- Formate: Often forms as an initial product and can be further converted to higher-value products like methanol.
- Carbon Monoxide: Generated from the one-electron reduction process and can diverge to form hydrocarbons.
- Hydrocarbons: Depending on the conditions, different lengths of carbon chains can form from the reduction of CO2 via various intermediates.
Understanding the stability and reactivity of these intermediates allows researchers to manipulate conditions to favor desired products. By adjusting factors such as temperature, pressure, and current density, it is possible to direct the reaction towards specific intermediates, which in turn aids in optimizing final product yields.
Role of Catalysts in CO2 Reduction
The role of catalysts in the electrochemical reduction of carbon dioxide is crucial. Catalysts increase the reaction rates without being consumed in the process. This characteristic is important for practical applications, as it allows for more efficient energy use and better product yields. In this section, we will explore the types of catalysts used in CO2 reduction, their efficiency, and stability considerations.
Types of Catalysts
There are various types of catalysts employed in CO2 reduction. Each type has its unique properties, which influence both the reaction pathways and the quality of the end products. Here are some of the most common types:
- Metallic Catalysts: Noble metals like silver or gold are often utilized in CO2 electroreduction. They enable the conversion of CO2 to hydrocarbons through different pathways.
- Transition Metal Catalysts: Copper is a notable example in this category. It selectively converts CO2 into higher-order alcohols and hydrocarbons.
- Metal Oxides: These can also serve as catalysts, often providing benefits in terms of stability and reusability. They can facilitate a range of chemical reactions.
- Molecular Catalysts: Consisting of small organometallic complexes, these catalysts can offer high selectivity for specific products.
Each catalyst comes with specific advantages and drawbacks, shaping its suitability for particular applications. The choice between these types is often a balance between cost, efficiency, and potential for long-term stability.
Catalyst Efficiency and Stability
Catalyst efficiency refers to how effectively a catalyst facilitates a given reaction. High efficiency is desired to minimize energy costs and maximize product outcomes. Stability is equally important; a stable catalyst maintains its performance over time, resulting in less frequent replacements and lower operational costs.
Key factors that affect efficiency and stability include:
- Surface Area: A larger surface area typically allows better interaction with CO2, increasing the chance of reaction.
- Structural Integrity: The catalyst structure must remain intact under reaction conditions. For instance, some materials may become inactive due to sintering or corrosion.
- Operating Conditions: Temperature and pressure play a role as well. The right conditions can enhance the activity of a catalyst.
Improved efficiency and stability will contribute to the feasibility of CO2 reduction technologies in practical applications.
Improved efficiency and stability will contribute to the feasibility of CO2 reduction technologies in practical applications.
In summary, understanding the various types of catalysts and their associated efficiencies and stabilities is essential. Catalysts are a linchpin in the efforts to make CO2 reduction not only practical but also economically viable.
Electrolytes in CO2 Electroreduction
In the context of electrochemical reduction of carbon dioxide, electrolytes serve as a crucial component. They facilitate the transport of ions, impacting the overall efficiency and effectiveness of the reduction process. Understanding electrolytes in CO2 electroreduction is essential for optimizing reaction conditions and improving product yield. Given their role, it is critical to explore the properties of the electrolytes used, as well as their influence on reaction efficiency.
Properties of Electrolytes
Electrolytes can differ significantly depending on their chemical composition and physical properties. A few key aspects include:
- Ionic Conductivity: This property determines how well the electrolyte can conduct ions. Higher ionic conductivity is generally advantageous. It can reduce energy losses and enhance overall efficiency.
- Stability: The stability of an electrolyte under various operating conditions is vital. Electrolytes that degrade or react undesirably may lead to reduced performance and poor product selectivity.
- Solvent Compatibility: The interaction between the electrolyte and the solvent can influence the solubility of reactants and products. Some electrolytes work well with specific solvents, maximizing efficiency.
Many studies explore various solvent-electrolyte combinations. For instance, aqueous electrolytes are often favored for their ability to promote proton transfer, while organic solvents can provide different advantages, such as higher solubility for certain products. Thus, choosing the right electrolyte requires careful consideration of these properties to achieve optimal performance.
Impact on Reaction Efficiency
The selection of electrolytes has a significant impact on the overall reaction efficiency during CO2 reduction. The efficiency can be influenced by several factors, including:
- pH Levels: The acidity or alkalinity of the electrolyte affects the reduction potential. This, in turn, influences the kinetics of the reaction and can enhance or hinder specific pathways.
- Ionic Strength: A higher ionic strength can improve the electrode kinetics, aiding in the rapid transfer of electrons. This can lead to improved product yields.
- Product Solubility: Some electrolytes increase the solubility of reaction products. This is particularly important for selective production of desired chemicals from CO2, as some products may rapidly precipitate out otherwise.
"The right electrolyte can transform the performance of CO2 electroreduction, optimizing yield while minimizing energy input."
"The right electrolyte can transform the performance of CO2 electroreduction, optimizing yield while minimizing energy input."
Efforts to optimize these factors are ongoing. Researchers are engaged in trial-and-error across numerous configurations, assessing how variations in electrolyte composition can help achieve greater efficiency in the CO2 reduction pathways. Overall, the appropriate electrolyte choices profoundly shape the landscape of electrochemical CO2 reduction.
Products of CO2 Electroreduction
The products of CO2 electroreduction are critical in understanding the efficiency and effectiveness of this technology in combatting climate change. They are not only essential for evaluating the success of electrochemical reactions but also have significant implications for sustainable energy practices. The field aims to transform carbon dioxide, a waste product, into valuable chemicals and fuels, thus closing the carbon loop and providing solutions that blend environmental responsibility with economic viability.
The significance of product selectivity cannot be overstated. Different electrochemical conditions and catalysts can lead to a variety of products. Methanol, formic acid, and carbon monoxide are notable examples, each having distinct applications and market potential. Understanding how to manipulate reaction conditions to favor one product over another can lead to advancements in both reaction efficiency and product utilization.
Product Selectivity
Product selectivity refers to the ability of the electrochemical process to favor the formation of specific products over others. This is vital as market demand often dictates the need for certain chemicals and fuels. Factors influencing selectivity include the type of catalyst used, the applied potential, and the electrolyte composition.
In many cases, improving selectivity leads to higher overall efficiency. Therefore, research focuses on optimizing parameters like catalyst surface area and reaction kinetics to control which product is formed. This often involves exploring novel catalysts or modifying existing ones to enhance their selectivity through techniques like doping or structural modification.
One challenge faced in this area is the trade-off between selectivity and activity. While it may be possible to enhance selectivity for one product, it can sometimes reduce the overall reaction rate, complicating the balance between achieving both high yield and fast production rates.
Applications of Reduction Products
The applications of the products generated from CO2 electroreduction are diverse, presenting a myriad of opportunities to enhance sustainability. For instance, methanol produced from CO2 can serve as a fuel or a feedstock for various chemicals.
- Chemical Manufacturing: Methanol is used in the production of formaldehyde, acetic acid, and other important compounds. Given the versatility of this alcohol, its production through renewable means presents an avenue for a reduced carbon footprint in chemical manufacture.
- Fuel Production: The conversion of CO2 to hydrocarbons can also lead to fuels that can directly replace fossil fuels without requiring significant changes to infrastructure. These fuels can thus play a role in decarbonizing the transportation sector.
The integration of these products into existing industrial processes further underscores the economic advantages of CO2 electroreduction.
The electrochemical reduction of CO2 presents not only environmental benefits but also economic potential by converting a liability into a valuable resource.
The electrochemical reduction of CO2 presents not only environmental benefits but also economic potential by converting a liability into a valuable resource.
Additionally, the market demand for sustainable solutions enhances the significance of developing these reduction products. As the global economy shifts towards greener alternatives, the business case for CO2-derived products becomes stronger, thereby encouraging further investment in research and development.
Advancements in Material Science
Advancements in material science are instrumental in enhancing the electrochemical reduction of carbon dioxide (CO2). This process is pivotal for addressing the urgent need for carbon management in the context of climate change. Improved materials play a crucial role in optimizing catalytic activity, efficiency, and sustainability of CO2 reduction methods. The exploration of new materials can lead to significant breakthroughs in performance and cost-effectiveness.
The integration of innovative materials like nanomaterials and two-dimensional (2D) materials has demonstrated transformative potential. These materials typically offer a high surface area to volume ratio, facilitating more active sites for reactions. Furthermore, advancements in synthesis techniques enable the development of materials tailored to specific electrochemical processes, maximizing their effectiveness.
Nanomaterials and Their Applications
Nanomaterials have emerged as a vital category in the advancement of catalysts for CO2 reduction. Their unique properties arise from their small size, which can lead to enhanced electronic and catalytic behavior. For example, nanoparticles can achieve high catalytic activity with minimal amounts, which is essential for reducing the overall cost of the process. Various types of nanomaterials, including metal nanoparticles and carbon-based nanostructures, have shown prowess in selective CO2 reduction.
Some notable applications include:
- Silver Nanoparticles: Known for their ability to selectively convert CO2 into ethanol, silver nanoparticles exhibit high activity under mild conditions.
- Graphene Oxide: Functions as a support for active sites within catalysts, enhancing the flow of electrons during the electrochemical reaction.
- Carbon Nanotubes: These exhibit excellent electrical properties and stability, improving the performance of composite catalysts.
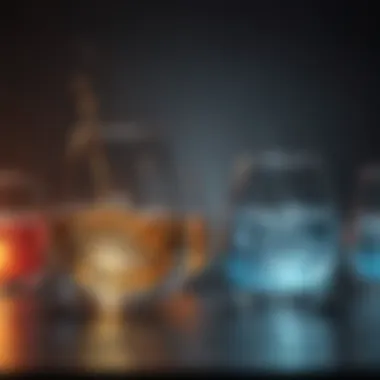
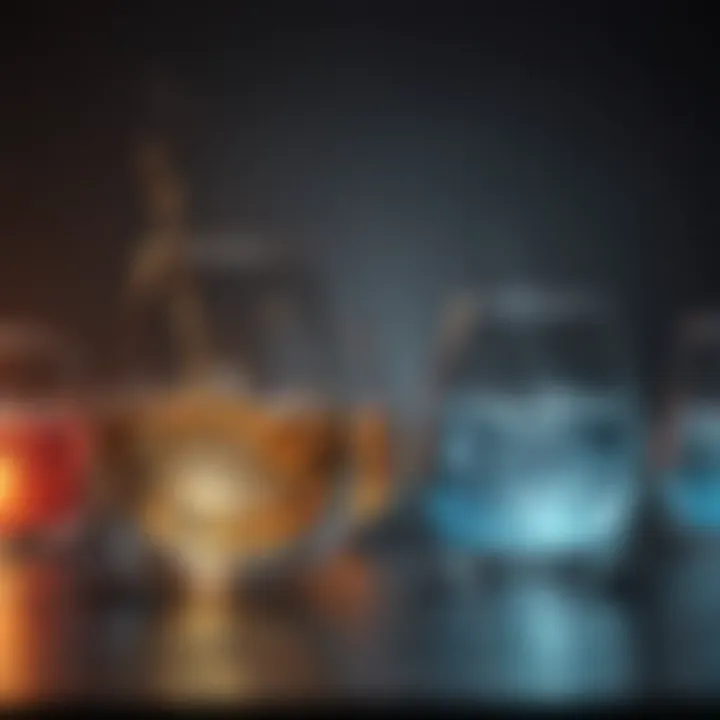
Nanomaterials can provide tailored solutions to specific challenges in CO2 reduction, including product selectivity and reaction kinetics. The ongoing research into their properties indicates great promise in scaling up these innovations for real-world applications.
2D Materials in CO2 Reduction
Two-dimensional materials, such as graphene and transition metal dichalcogenides, have gained attention due to their unique properties. These materials possess exceptional electrical conductivity and mechanical strength, making them suitable for enhancing catalytic activity in electrochemical reduction processes. For instance, the large aspect ratio of 2D materials allows for more surface area available for reaction, thus improving efficiency.
Recent studies have reported that the incorporation of 2D materials into catalyst designs can significantly improve selectivity toward desired products while minimizing by-products.
- Graphene: When used as a conductive support, graphene enhances electron transfer, which is vital in electrochemical reactions.
- Molybdenum disulfide (MoS2): This material has shown promise due to its unique catalytic properties, allowing for efficient CO2 conversion to fuels like methane and methanol.
Ongoing research aims to better understand the mechanisms of 2D materials in CO2 reduction processes, which could lead to further optimization in terms of performance and scalability. The potential of these materials to integrate with existing technologies presents an exciting frontier in the field of electrochemical reduction.
Innovative Approaches to CO2 Reduction
Innovative approaches to the electrochemical reduction of carbon dioxide represent a crucial area in developing effective strategies for climate change mitigation. These approaches showcase how creative methodologies can enhance CO2 reduction processes, influencing both efficiency and scalability. Novel techniques in this domain allow researchers and engineers to address the technical challenges that accompany traditional methods.
Hybrid Systems
Hybrid systems combine different technologies or materials to optimize the reduction of CO2. By integrating various processes, such systems can enhance the yield of desired products while minimizing energy consumption. For instance, combining electrochemical cells with biological systems can lead to more effective CO2 utilization and conversion. In such setups, microorganisms are employed alongside electrocatalysts to convert CO2 into valuable chemicals. This synergy can amplify the overall efficiency of the process, potentially making it more commercially viable.
Moreover, hybrid systems facilitate the use of waste streams, which can be significant sources of CO2. Utilizing these feeds not only reduces overall emissions but can also minimize costs. The versatility of hybrid systems allows for adaptability in various industrial contexts, increasing their attractiveness as a solution for CO2 reduction.
Integration with Renewable Energy Sources
Integrating renewable energy sources with electrochemical CO2 reduction is another innovative approach capturing attention. This method aims to power the reduction process with energy derived from renewable resources, such as solar or wind energy. By coupling electrochemical systems with solar panels, researchers can directly convert sunlight into chemical energy during CO2 reduction processes.
The benefits are evident. First, it minimizes the dependency on non-renewable energy sources, effectively lowering the carbon footprint of the entire process. Second, it increases the sustainability of CO2 reduction technologies by using clean energy. This combination is often referred to as solar-driven CO2 electroreduction.
Furthermore, utilizing renewable energy can lead to improved economics for carbon capture and conversion technologies, making them more accessible for widespread application. This alignment with global clean energy goals makes the integration of renewable energy sources a vital facet of contemporary CO2 reduction innovation.
Innovative approaches to CO2 reduction have the potential to transform how we tackle climate change, merging technology and sustainability for a more viable future.
Innovative approaches to CO2 reduction have the potential to transform how we tackle climate change, merging technology and sustainability for a more viable future.
Challenges in Electrochemical CO2 Reduction
The electrochemical reduction of carbon dioxide faces various significant challenges that hinder its widespread adoption and efficiency. Understanding these challenges is essential for advancing research and development in this field. Among the primary considerations are technical barriers and economic viability, both of which influence the feasibility of implementing CO2 reduction technologies in practical applications.
Technical Barriers
The technical barriers in CO2 electroreduction stem from a range of factors that affect efficiency and effectiveness in the conversion process. One of the main challenges is the need for high selectivity towards desired products. The formation of unwanted byproducts can significantly reduce the overall yield and complicate downstream processing. Complex reaction pathways lead to difficulty in optimizing reaction conditions, which ultimately impacts overall performance.
Moreover, the stability of electrocatalysts is a critical concern. Many catalysts exhibit declining activity over time, necessitating frequent replacement or regeneration. This instability complicates the continuous operation of electrochemical cells, which is crucial for scalability in industrial settings. Additionally, maintaining suitable operating conditions, such as temperature and pressure, adds to the complexity of the system design.
"The development of robust and stable electrocatalysts is crucial for the efficiency of electrochemical CO2 reduction."
"The development of robust and stable electrocatalysts is crucial for the efficiency of electrochemical CO2 reduction."
Another barrier involves the electrolytes used in the process. The choice of electrolyte can have significant implications for the kinetics of the reaction, impacting both the efficiency and overall energy consumption. Finding the right blend of electrolytic properties to facilitate CO2 reduction while preventing side reactions remains a challenge for researchers.
Economic Viability
Economic viability is another crucial factor to consider in the adoption of electrochemical CO2 reduction technology. The initial capital investment for setting up electrochemical systems can be substantial. Equipment costs, including the development of advanced materials for electrodes and electrolytes, may escalate the financial burden. Furthermore, ongoing operational costs, such as energy consumption and maintenance, can impact the overall profitability of such systems.
The market for CO2 reduction products must also be economically attractive. While the potential applications of reduced CO2 products are many, their market readiness is often limited due to factors such as price competitiveness compared to traditional fossil fuel products. Policymakers and industries must align on creating a favorable environment that encourages investments in this technology.
Additionally, establishing a supportive regulatory framework is essential for stimulating growth in this sector. Policies that favor sustainable practices can help mitigate the risks involved in developing and deploying CO2 reduction technologies.
In summary, the challenges in electrochemical CO2 reduction encompass a wide array of technical and economic factors. Addressing these issues is vital for enabling this technology's transition from research to practical application, promoting the role of CO2 electroreduction in achieving global sustainability goals.
Environmental Impacts of CO2 Reduction
The environmental impacts of CO2 reduction are essential to understanding the broader implications of this technology in combating climate change. As society pushes towards greater sustainability, the methods developed for the electrochemical reduction of carbon dioxide are increasingly under scrutiny. Evaluating these impacts is critical not just from a scientific perspective, but also for informing policy decisions and public perception on climate action.
One significant aspect to consider is the carbon footprint associated with the electrochemical processes. While reducing CO2 levels is a primary goal, the energy sources used for these reductions can offset some of these benefits. If the energy required comes from fossil fuels, it can potentially negate the advantages of capturing CO2. Conversely, if renewable energy sources such as solar or wind power are employed, the overall carbon footprint can be significantly lowered.
- Key considerations include:
- Type of energy sources utilized
- Efficiency of the electrochemical systems
- Potential emissions generated during manufacturing of materials used
Thus, a thorough analysis must be performed to grasp how these systems contribute to greenhouse gas emissions beyond the immediate reduction of CO2. This analysis is crucial in ensuring sustainable practices are integral to research and application.
Carbon Footprint Analysis
The carbon footprint analysis of CO2 reduction technologies provides a clear picture of environmental viability. This analysis involves assessing all stages of the process from energy input to operational emissions. For instance, if a new catalyst allows for more efficient energy use, it reduces the total emissions further downstream.
Moreover, a quantitative assessment must include direct and indirect emissions:
- Direct emissions:
- Indirect emissions:
- Emissions from energy consumption during CO2 reduction
- Emissions from raw material extraction
- Transport emissions for inputs and outputs
- Emissions from production processes
Evaluating these factors allows researchers to make informed decisions about which technologies to pursue further. Understanding how to minimize the carbon footprint while maximizing CO2 reduction efficiency remains a significant challenge and goal for researchers in this field.
Lifecycle Assessment
Lifecycle assessment (LCA) is critical in comprehensively evaluating the environmental impacts of CO2 reduction technologies. LCA encompasses all stages of a product's life, from production through use and eventual disposal or recycling.
- Stages to consider in LCA:
- Raw material extraction
- Manufacturing processes
- Use phase
- End-of-life options
Conducting an LCA ensures that all impacts are accounted for, providing a holistic overview. With this assessment, it becomes easier to identify areas for improvement. It may highlight that certain materials or processes need redesigning to enhance sustainability.
Furthermore, an accurate LCA can guide future research efforts and regulatory frameworks to prioritize technologies with lower environmental impacts. Emphasizing this detailed examination throughout the research and implementation phases is essential for achieving significant long-term climate goals.
"A thorough lifecycle assessment can reveal hidden environmental costs and benefits, guiding better decision-making in CO2 reduction strategies."
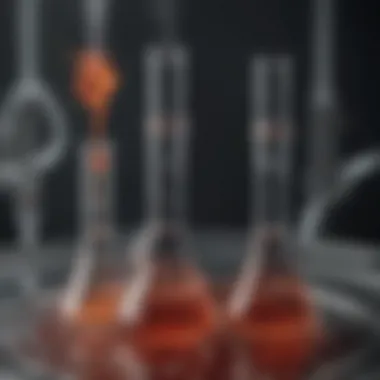
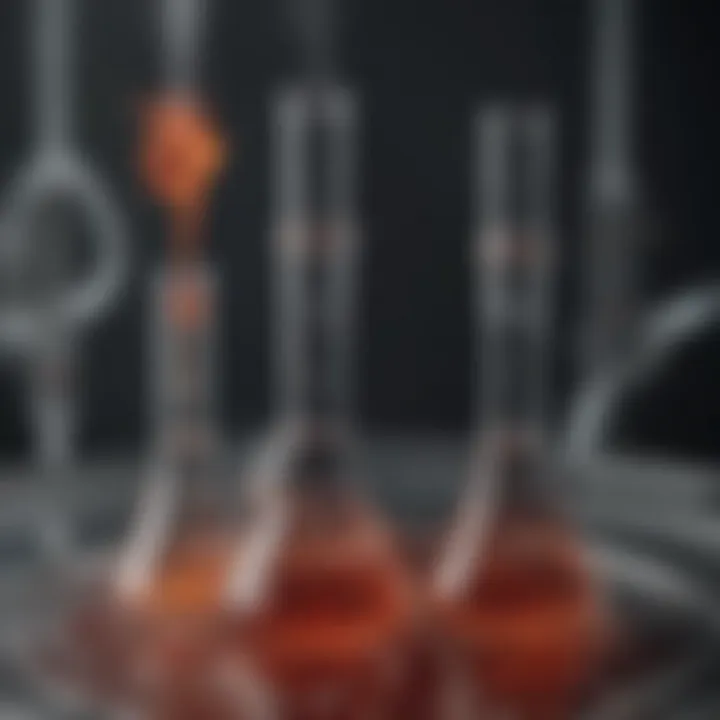
"A thorough lifecycle assessment can reveal hidden environmental costs and benefits, guiding better decision-making in CO2 reduction strategies."
Regulatory and Policy Considerations
The regulatory and policy landscape surrounding the electrochemical reduction of CO2 is of paramount importance. As this technology evolves, effective governance can significantly influence its adoption and success. This section aims to elucidate critical elements, benefits, and considerations relevant to regulatory frameworks affecting CO2 electroreduction. Recent initiatives underscore the need for a structured approach to harnessing this technology for sustainable energy solutions.
Current Regulations
Regulatory measures currently in place aim to foster research and development in carbon reduction technologies while ensuring environmental protection and public safety. In the United States, regulatory bodies such as the Environmental Protection Agency (EPA) set standards that impact carbon capture and utilization. These regulations often focus on emissions limits and the environmental impact of various technologies. Internationally, agreements like the Paris Agreement motivate countries to set emission reduction targets, indirectly encouraging research in electrochemical reduction technologies.
Regulations can vary significantly among regions. For instance, the European Union has implemented stringent guidelines that promote green technologies. The EU's "Green Deal" is a framework enhancing support for innovations, aiming to achieve carbon neutrality by 2050. In this context, policies that support funding for research and incentives for industries adopting sustainable practices are prevalent. Furthermore, intellectual property rights associated with innovative processes also fall under regulatory scrutiny, impacting how new technologies are commercialized.
Future Policy Directions
As the field progresses, future policy directions will be critical in shaping the evolution of CO2 electroreduction technologies. Policymakers must ensure that new regulations foster innovation while maintaining environmental standards. One potential direction involves integrating carbon pricing mechanisms that incentivize emissions reductions. By making carbon emissions more costly, stakeholders in both the public and private sectors may be more likely to invest in CO2 reduction technologies.
Another aspect to consider is the development of more flexible regulatory frameworks. These updated policies should accommodate the rapid pace of technological advancement. Such flexibility would also allow researchers and companies to pivot quickly to new methodologies based on emerging findings. Collaboration among governments, academia, and industry is essential in crafting effective policies that support technical progress while maximizing societal benefits.
Additionally, expanding public-private partnerships can accelerate the practical implementation of CO2 electroreduction technologies. Incentives for companies that engage in sustainable practices within the regulatory framework can enhance the transition to greener processes. In summary, as we look toward the future, cohesive policy frameworks and innovative regulatory strategies will be instrumental in driving the progress and adoption of electrochemical CO2 reduction.
Global Perspectives on CO2 Reduction
The topic of global perspectives on CO2 reduction is vital in understanding how different regions approach the challenge of mitigating climate change through electrochemical methods. Each country brings its unique experiences, policies, and technological advancements to the table. By examining these diverse strategies, we can identify best practices and foster collaborative international efforts that enhance the efficacy of CO2 reduction.
The significance of this topic lies in its ability to showcase not only the varying levels of commitment and innovation but also how local economies and regulatory environments shape the adoption of electrochemical reduction techniques. Countries with robust research institutions, for example, may be better positioned to lead advancements in electrochemical technologies.
Moreover, exploring global case studies fosters an understanding of shared challenges, such as funding limitations and technological hurdles. This awareness can encourage nations to work together, promoting shared knowledge and pooled resources to break down existing barriers.
Case Studies from Various Countries
Case studies from various countries illustrate the myriad approaches to CO2 reduction. For instance, Germany has emerged as a leader in integrating renewable energy with CO2 electrocatalysis. German researchers focus heavily on combining solar power with CO2 reduction technologies, capitalizing on both environmental and economic benefits. The nation’s commitment to sustainability is evident in initiatives that encourage funding for innovative CO2 reduction projects.
In contrast, China is investing significantly in scaling up existing technologies. As the largest emitter of CO2, China's focus has been on implementing electrochemical systems on an industrial scale. Chinese firms are actively improving the efficiency of CO2 reduction methods, reflecting a dual goal of reducing emissions while promoting economic growth through the development of green technologies.
The United States provides another noteworthy example. Through various governmental and private initiatives, the U.S. is pushing to advance electrochemical CO2 reduction technologies. Collaborative projects between universities and industries have led to breakthroughs in catalyst efficiency, showcasing how combined efforts can yield substantial advancements.
These cases underscore that each country, regardless of its economic conditions, can contribute valuable insights and methods to the global goal of CO2 reduction.
Collaborative Efforts in Research
Collaborative efforts in research are essential in tackling CO2 reduction at a global scale. The intricate and technical nature of electrochemical reduction demands cross-disciplinary cooperation. Research institutions, industry leaders, and governmental bodies must align their interests and objectives for effective outcomes.
Global collaborations have been established, such as the International Energy Agency’s Carbon Capture, Utilization, and Storage program, which brings together experts from multiple countries. This platform encourages shared research efforts, facilitating feedback and peer review that ultimately leads to improved outcomes.
Additionally, partnerships between academia and industry foster innovation. For instance, institutions like Stanford University and Tesla work on developing efficient electrochemical processes for CO2 reduction. Such collaborations often lead to practical applications and scale-up processes that might otherwise take much longer if pursued independently.
Furthermore, funding plays a crucial role in these collaborations. By pooling resources from different nations and sectors, researchers can access advanced technologies and materials. This cooperative approach allows for shared risks and rewards, ultimately promoting a faster pathway to viable CO2 solutions.
Through global collaboration, we can overcome technical barriers and accelerate progress in reducing atmospheric CO2 concentrations.
Through global collaboration, we can overcome technical barriers and accelerate progress in reducing atmospheric CO2 concentrations.
In summary, global perspectives on CO2 reduction highlight the importance of shared knowledge and cooperative efforts. By learning from the experiences of different countries, we can devise more effective strategies that contribute to a sustainable future.
Future Directions of CO2 Electrochemical Reduction Research
Future research in the electrochemical reduction of CO2 is essential to unlocking its full potential as a viable pathway for carbon mitigation. As global efforts intensify to address climate change, the significance of this field cannot be overstated. Scientists are compelled to devise innovative strategies that not only enhance reaction efficiencies but also integrate these technologies into existing frameworks. A multifaceted approach can yield benefits across environmental, economic, and societal dimensions.
Emerging Technologies
Emerging technologies represent a cornerstone of the future direction in CO2 electrochemical reduction. Advances in nanotechnology have cultivated a range of novel materials with tailored properties that promote accelerated CO2 conversion. For example, the introduction of atomic layer deposition techniques has enabled the production of highly ordered nanostructures. These structures exhibit improved electrocatalytic activity and selectivity toward desired products.
Research is also increasingly looking at hybrid electrochemical systems that couple with biocatalysis. These systems could exploit both biological and electrochemical pathways to optimize yield and efficiency. Notable trends include:
- Utilization of machine learning to predict and facilitate new catalyst designs.
- Integration of organic frameworks for enhanced selectivity in electroreduction processes.
- Exploration of new electrolyte formulations that promote greater ionic conductance under varied operational conditions.
Batch production methods are evolving as well, with an emphasis on continuous flow systems that promise improved mass transport and energy efficiency. The exploration of these technologies presents a fertile ground for innovation and can lead to outcomes that significantly improve the overall feasibility of CO2 reduction processes.
Scalability Considerations
Scalability is another critical element that researchers must address when considering future directions in CO2 electrochemical reduction. The transition from laboratory-scale processes to commercial applications involves numerous challenges. It is paramount that the methods developed are not only effective on a small scale but are also adaptable for large-scale production. Here are some key considerations:
- Cost-effectiveness of materials: The economic viability of using rare or expensive materials must be carefully evaluated against their performance benefits.
- System design: Engineering efficient large-scale reactors that can operate reliably over extended periods remains crucial.
- Energy consumption: Research must focus on minimizing the energy input required for electrochemical processes.
- Integration into industrial processes: Future technologies should aim at alignign with existing industrial infrastructure to enhance acceptability and reduce startup costs.
Ultimately, addressing scalability will determine whether innovative technologies can become commercially viable solutions to CO2 emissions. The synthesis of these advancements in technology and consideration of scalability holds promise for transforming the dynamics of CO2 reduction and its impact on global sustainability efforts.
"The future of electrochemical CO2 reduction lies in our ability to innovate and scale efficiently, addressing both technical and economic challenges."
"The future of electrochemical CO2 reduction lies in our ability to innovate and scale efficiently, addressing both technical and economic challenges."
Finale
The conclusion of this article highlights the crucial role of electrochemical reduction of CO2 in addressing the pressing climate challenges we face today. The processes discussed are not merely theoretical but hold substantial implications for both technology and policy. A comprehensive understanding of these mechanisms can lead to real-world applications that might significantly lower atmospheric CO2 levels.
In our exploration, it became clear that advancements in materials science and electrochemical technologies form a backbone for efficient CO2 reduction. The ongoing research into innovative catalysts, nanomaterials, and electrolytes is not just an academic pursuit; it is a race against time to devise solutions that could redefine our energy landscape.
Moreover, the successful integration of these technologies with renewable energy sources presents a unique opportunity to create a sustainable energy ecosystem. As seen in various case studies globally, collaborations across borders highlight the importance of a collective effort in research and implementation.
"The electrochemical reduction processes examined are a vital component of our future energy strategy, potentially paving the way for significant reductions in greenhouse emissions."
"The electrochemical reduction processes examined are a vital component of our future energy strategy, potentially paving the way for significant reductions in greenhouse emissions."
Summary of Key Findings
Several key findings emerged from this investigation into CO2 electroreduction:
- Mechanistic Insights: Understanding the electrocatalytic pathways involved in CO2 reduction is essential for enhancing the efficiency of the conversion processes.
- Catalyst Variability: Different types of catalysts demonstrate varying levels of efficiency and stability, indicating the need for continuous development in this area.
- Electrolyte Impact: The choice of electrolytes significantly influences reaction efficiency; thus, optimizing these components is critical for future advancements.
- Product Utilization: The selectivity of reduction products is directly linked to their applicability in various industries, emphasizing the importance of identifying valuable end products.
These findings underscore the multifaceted nature of CO2 reduction technology and its implications for energy sustainability.
Implications for the Future
The future of CO2 electrochemical reduction research appears promising yet challenging. Continued advancements can lead to:
- Enhanced Efficiency: Research is likely to further improve the efficiency of this process, making it more viable for industrial applications.
- Broader Adoption: As technologies mature, we can expect broader adoption across various sectors, helping mitigate climate change effectively.
- Policy Development: There will be a need for well-structured policies that support innovation in this field while ensuring compliance with environmental goals.
- Global Collaborations: Increased collaborative efforts between academic institutions, industry leaders, and governments will be essential in driving innovations that are both sustainable and economically feasible.