PEM Electrolysers: Insights into Hydrogen Production
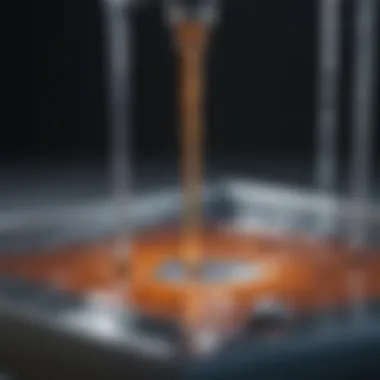
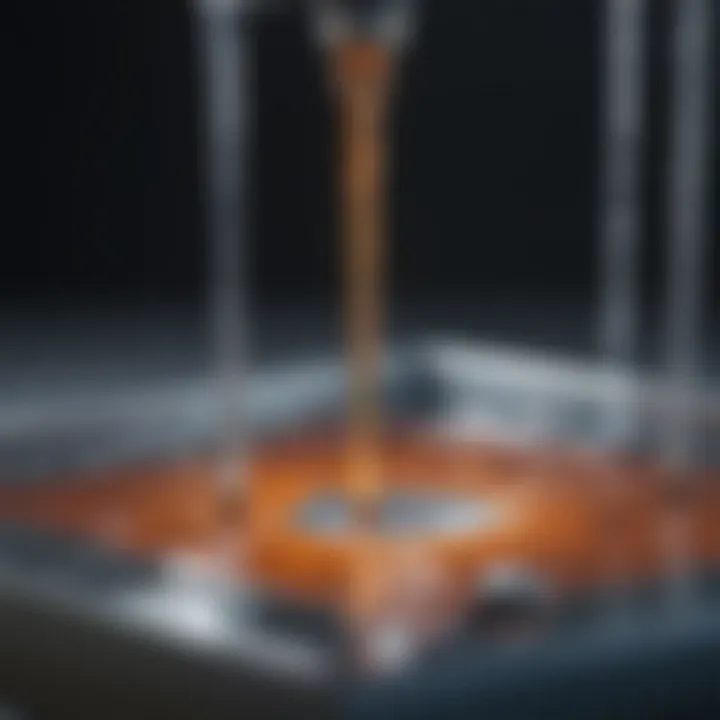
Intro
The quest for sustainable energy has pushed various technologies into the limelight, but few have garnered as much attention as proton exchange membrane (PEM) electrolysers. These devices turn water into hydrogen gas, an essential fuel with an abundance of applications—ranging from energy production to transportation. As the world seeks to curb carbon emissions and transition towards renewable energy, PEM electrolysers stand at the forefront, showcasing both promise and potential hurdles.
Hydrogen itself isn’t a new player on the energy stage; however, the efficient and eco-friendly production of hydrogen through electrolysis is where PEM technology shines. The inherent mechanisms at play within these electrolysers, coupled with their advantages and limitations, underscore the relevance of diving deeper into this field. This examination will illuminate their role in various sectors, highlighting not just the current state of the technology, but also its trajectory into the future.
"In a world increasingly dependent on green energy, PEM electrolysers symbolize a beacon of hope in hydrogen production, presenting a viable pathway to sustainability."
"In a world increasingly dependent on green energy, PEM electrolysers symbolize a beacon of hope in hydrogen production, presenting a viable pathway to sustainability."
Let’s break down the fundamental concepts and explore the latest advancements in this rapidly evolving area.
Prelude to PEM Electrolysers
In an era where the quest for sustainable energy solutions has become paramount, Proton Exchange Membrane (PEM) electrolysers are at the forefront of hydrogen production technology. This section serves to dive into the pivotal role these electrolysers play in generating hydrogen through the electrolysis of water, a process that not only aids in the transition toward cleaner energy but also aligns with global goals of reducing carbon emissions. Their importance cannot be overstated, as they provide a viable pathway to harness renewable energy sources, turning excess electricity into hydrogen fuel, which can be stored and utilized when needed.
Understanding Electrolysis
Electrolysis is a process that involves splitting water into hydrogen and oxygen using electric current. At its core, it is a basic reaction — water molecules are decomposed into their elemental constituents. This occurs through two electrodes: the anode and the cathode.
- At the anode, water is oxidized, producing oxygen gas, protons, and electrons.
- At the cathode, protons from the electrolyte combine with electrons from the external circuit to produce hydrogen gas.
What makes PEM electrolysis particularly significant is how it streamlines this process. Unlike traditional electrolysers, PEM technology uses a solid polymer membrane as the electrolyte, which improves efficiency and reduces the complexity of the system. This is not just a minor innovation; it means faster response times and excellent performance even under varying conditions — essentials for modern energy systems.
What is PEM Technology?
PEM technology is an advanced methodology in hydrogen production that relies on the principles of electrolysis while leveraging the unique characteristics of Proton Exchange Membranes. These membranes are crucial components that facilitate the selective transport of protons while blocking gases like hydrogen and oxygen from passing through, thus driving the reactions efficiently without crossover losses.
The use of PEMs contributes to several notable benefits:
- High Efficiency: A significant increase in efficiency compared to other methods, particularly under fluctuating load conditions.
- Compactness: PEM electrolysers are generally smaller and lighter, making them easier to integrate into various applications.
- Scalability: Their design allows for scaling up production, making them suitable for both small-scale and larger industrial applications.
This combination of efficiency and adaptability positions PEM electrolysers as a game changer in the growing hydrogen economy.
"The role of PEM electrolysers in hydrogen production is akin to the foundation of a new energy paradigm, one that places sustainability and efficiency at its heart."
"The role of PEM electrolysers in hydrogen production is akin to the foundation of a new energy paradigm, one that places sustainability and efficiency at its heart."
In summary, PEM technology not only enhances the effectiveness of hydrogen production through electrolysis but also supports broader goals of transitioning to clean energy sources. As we explore this topic in greater depth, the subsequent sections will illuminate the operational principles, advantages, limitations, and future outlook of PEM electrolysers, offering readers a comprehensive understanding of their significance in renewable energy landscapes.
Operational Principles of PEM Electrolysers
Understanding the operational principles of Proton Exchange Membrane (PEM) electrolysers is central to grasping their role in hydrogen production. Electrolysis, the process by which water is split into hydrogen and oxygen, relies on the seamless cooperation of various components and mechanisms within these systems. Essentially, this section will unpack how these principles not only underscore efficiency and effectiveness in hydrogen generation, but also highlight the broader implications for renewable energy applications and technologies.
Electrochemical Reactions
The performance of PEM electrolysers hinges on electrochemical reactions that occur at the electrodes. These reactions are the lifeblood of the electrolyser, determining its efficiency and overall effectiveness in producing hydrogen.
Anode and Cathode Reactions
When discussing Anode and Cathode Reactions, it’s crucial to realize they represent the starting point for the electrolysis process. At the anode, water is oxidized. This decomposition produces protons and oxygen gas while releasing electrons into the circuit. The equation can be simply represented as:
[ 2 H_2O ightarrow O_2 + 4 H^+ + 4 e^- ]
On the opposite side, at the cathode, those released protons recombine with electrons to form hydrogen gas. The reaction can be summed up as follows:
[ 4 H^+ + 4 e^- ightarrow 2 H_2 ]
One of the key characteristics of these reactions is their ability to operate at competitive standards to traditional water electrolysis methods. The ability to produce hydrogen at lower temperatures and pressures can be extremely advantageous. Furthermore, it minimizes energy loss, which is a hallmark of PEM technology.
Despite these advantages, it’s worth noting that the requirement for precious metals such as platinum for the electrodes can be a point of concern. High costs associated with these materials may restrict deployment in large-scale scenarios, but research is ongoing to develop alternatives that could reduce these expenses over time.
Overall Reaction Mechanism
The Overall Reaction Mechanism in PEM electrolysis is a sum of the anode and cathode reactions we just outlined, but has broader implications. Understanding this mechanism helps illustrate how the electrolysers transform electrical energy from renewable sources into usable hydrogen fuel. It effectively bridges the gap between different energy systems.
The unique feature of PEM technology lies in its swift response to load changes. The overall mechanism can adapt rapidly to fluctuations in electricity supply, making PEM electrolysers a prime candidate for pairing with variable renewable energy sources like solar and wind. This adaptability is a significant asset in today's energy landscape where demand for flexibility is paramount.
However, despite these strengths, the need for smart integration with energy management systems cannot be overstated. The efficiency of the overall process can greatly diminish if not carefully managed, leading to wasted energy—a scenario that’s far from ideal, especially when striving for sustainability.
Membrane Functionality
Diving deeper, Membrane Functionality in PEM electrolysers cannot be overstated. The polymer electrolyte membrane plays a central role in maintaining ionic conductivity while preventing electrical short-circuiting. Its performance is crucial for the efficiency and durability of the system.
The membrane allows only protons to pass through, creating a segregated environment for the production of hydrogen and oxygen, which is vital for safety and efficiency. The need for innovation in membrane materials is pressing. Enhancements in durability and conducting properties could greatly extend the lifespan of PEM electrolysers, making them even more economically viable for various applications.
In summary, the operational principles of PEM electrolysers provide valuable insight into hydrogen production mechanics. From intricate electrochemical reactions to the pivotal role of the membrane, a clearer understanding unfolds, revealing both the potential and the challenges faced in the quest for sustainable energy solutions.
Components of PEM Electrolysers
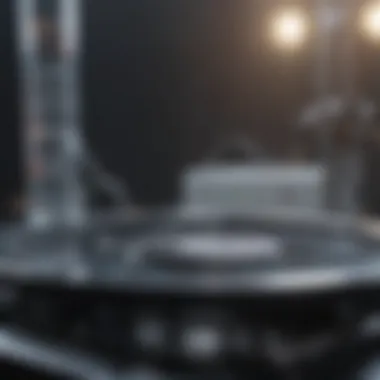
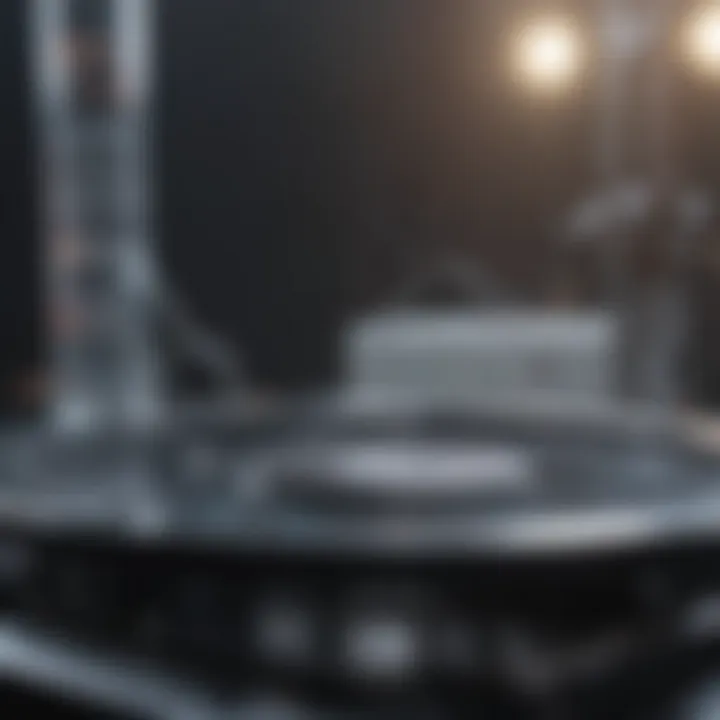
The importance of understanding the components of PEM electrolysers cannot be overstated. Each part plays an integral role in the overall efficiency and functionality of hydrogen production through electrolysis. A solid grasp of these components not only informs design improvements but also guides operational practices and innovations. Let's break down the key elements that make PEM electrolysers work like a well-oiled machine.
Membranes
Membranes are the heart of PEM electrolysis. They serve as selective barriers, allowing protons to pass while blocking electrons and gases. These membranes are usually made from a polymer material like Nafion, which offers excellent proton conductivity and mechanical stability. They hold the power to influence efficiency directly, as the conductivity of the membrane will determine how much energy is wasted in the process.
Moreover, durability is a critical consideration; over time, membranes can degrade due to high temperatures and environmental stressors. Therefore, ongoing research aims to enhance membrane resilience and reduce costs associated with providing high-performance materials. Investing in the right membrane can substantially optimize the overall efficiency of the electrolyser, making it a focal point of continued innovation.
Electrodes
Electrodes are the catalysts for the electrochemical reactions taking place within PEM electrolysers. Each cell typically consists of an anode and a cathode, with each electrode requiring specialized materials to facilitate optimal reactions. For instance, platinum and iridium are often used due to their superior catalytic properties. However, the high cost of these materials can be a double-edged sword. Researchers are actively pursuing alternative catalysts that are less expensive but still efficient. Transition metals, for example, are showing promise in some studies.
- Anode: In the anode, water is oxidized, releasing oxygen gas and protons.
- Cathode: In contrast, the cathode's role is to reduce the protons, yielding hydrogen gas as a product.
It’s crucial that both electrodes have good surface area and conductivity to ensure effective electrochemical reactions. Optimizing these materials leads to an increase in hydrogen yield while lowering energy consumption.
Flow Fields and Gaskets
Flow fields and gaskets may not get as much spotlight but are critical to the process. Flow fields guide the distribution of reactants to the electrodes while ensuring efficient gas removal during operation. This design feature helps maintain consistent operation and ensures no stagnant zones develop.
Moreover, gaskets provide vital sealing between the cells to prevent gas mixing – an essential safety measure. These components need to be made from resilient yet flexible materials to withstand pressures and temperatures during operation.
Consider this: The efficiency of the entire electrolyser can hinge on how well these components are designed and integrated. A small leak or a poorly distributed flow channel can lead to significant energy losses.
Consider this: The efficiency of the entire electrolyser can hinge on how well these components are designed and integrated. A small leak or a poorly distributed flow channel can lead to significant energy losses.
Advantages of PEM Electrolysers
PEM electrolysers stand out in the arena of hydrogen production technology, primarily due to their unique advantages that cater to both emerging and established markets. Understanding these benefits is crucial for those engaged in energy solutions, be it in academia, industry, or research sectors. Let's delve into some of the most remarkable advantages offered by PEM electrolysis.
High Efficiency
One of the foremost benefits of PEM electrolysers is their high efficiency. Unlike traditional alkaline electrolysis, PEM systems often claim efficiencies in the range of 60 to 80%. This is largely attributed to their ability to sustain higher current densities, which translates to a greater hydrogen production rate per unit of input energy.
- Electrolyte Properties: The proton exchange membrane (PEM) serves as a robust conductor of protons, allowing them to travel from the anode to the cathode efficiently.
- Minimal Energy Loss: With a sleek setup and materials like iridium and platinum in the catalysts, PEM electrolysers minimize energy loss, which often plagues other electrolytic methods.
This high efficiency not only enhances the productivity of hydrogen generation but also contributes to the overall viability of hydrogen as a clean energy carrier.
Compact Design
Another striking appeal of PEM electrolysers is their compact design. The architecture is often less bulky compared to other technologies, making these systems more adaptable to a variety of settings. This compactness brings several advantages:
- Space Saving: In an age where efficiency is key, devices that take up less real estate are increasingly valuable, particularly in urban environments.
- Modular Potential: Smaller footprint designs allow for modular installations, enabling users to scale up hydrogen production without a complete overhaul of existing systems.
- Enhanced Integration: The compact nature facilitates easier integration within renewable energy setups or industrial applications where space is limited.
Essentially, PEM electrolysers provide flexibility that larger systems cannot offer, making them a pragmatic choice for urban developers and energy planners.
Rapid Response to Load Changes
PEM electrolysers shine when it comes to dynamic operational capabilities. The ability to respond swiftly to load changes is invaluable, especially when integrating with intermittent renewable energy sources like solar and wind.
- Real-Time Adjustment: These systems can ramp production up or down almost instantly, adapting to fluctuations in energy supply or demand.
- Operational Resilience: Such responsiveness minimizes downtime and enhances overall system reliability, making it ideal for grid stability.
This characteristic positions PEM electrolysers as a crucial technology not only for hydrogen production but also for creating resilient energy systems that can cope with the challenges of modern energy landscapes.
"Harnessing the potential of PEM technology involves acknowledging both its nuanced advantages and the specific needs of its applications."
"Harnessing the potential of PEM technology involves acknowledging both its nuanced advantages and the specific needs of its applications."
In summary, the advantages of PEM electrolysers are manifold, showcasing high efficiency, compact design, and quick adaptability. These traits underscore their critical role in the advancement of hydrogen production technology and the broader goals of sustainable energy implementation.
Limitations and Challenges
While proton exchange membrane (PEM) electrolysers present numerous benefits, it is equally important to scrutinize their limitations and challenges. Understanding these aspects is crucial not only for potential investors but also for researchers dedicated to pushing the boundaries of this technology. Recognizing where PEM electrolysers falter can provide insights for future advancements, ultimately steering the industry toward more effective solutions. In this section, we will explore the main challenges, notably focusing on the cost of materials, durability and lifespan, and scalability issues.
Cost of Materials
The cost of materials used in PEM electrolysis is a prominent area of concern. High-performance membranes, electrodes, and catalysts are often based on expensive materials, such as platinum and iridium. These elements can inflate production costs significantly, making it difficult for PEM technology to compete with other hydrogen production methods, like alkaline electrolysis.
Moreover, as the global demand for hydrogen increases, the competition for these precious materials is expected to tighten, potentially exacerbating pricing issues.
- Factors to Consider:
- The price volatility of precious metals can drastically impact the operational viability of PEM electrolysers.
- Research into alternative materials that are both cost-effective and efficient remains a priority in the field.
"Only by investing in research on alternative materials can the industry hope to bring down costs while maximizing efficiency."
"Only by investing in research on alternative materials can the industry hope to bring down costs while maximizing efficiency."
Despite these costs, researchers are experimenting with new catalyst structures and materials derived from more abundant sources. These innovations can potentially change the game but remain in development stages for wide-scale deployment.
Durability and Lifespan
Another point of contention lies in the durability and lifespan of PEM electrolysers. Though designed for efficiency, the lifespan of membranes is often significantly shorter compared to traditional technologies. Factors such as temperature fluctuations, PID (proportional-integral-derivative) control issues, and the corrosive nature of the electrolytic environment can lead to degradation over time.
- Implications:
- A shorter lifespan necessitates more frequent replacements, translating into higher long-term costs.
- This not only affects economic viability but also raises questions about the environmental consequences of disposing of worn-out membranes.
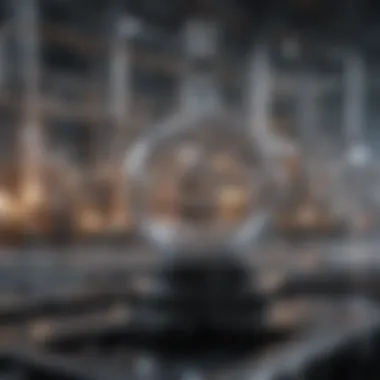
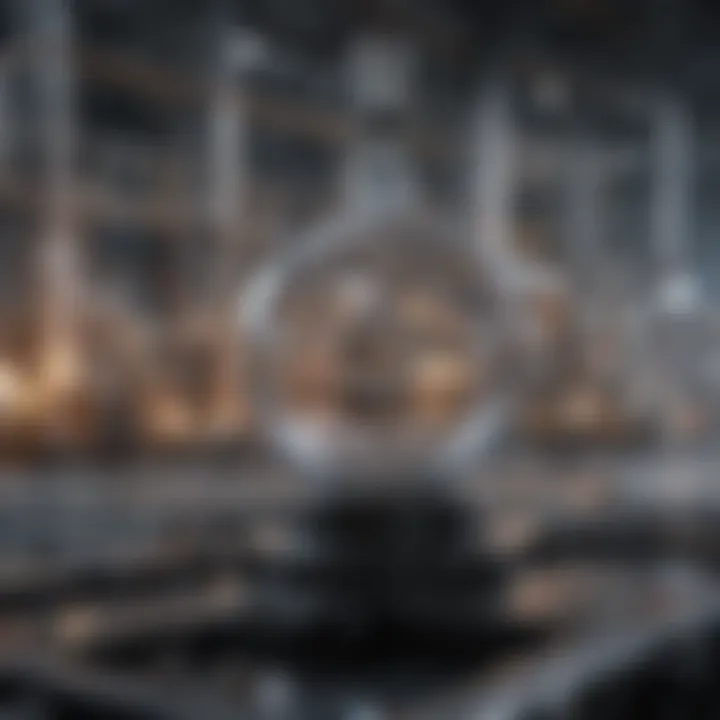
Efforts are underway to enhance durability. For example, advancements in polymer chemistry could yield membranes that withstand harsher conditions, thus prolonging their operational lifespan.
Scalability Issues
Scalability poses its own set of challenges for PEM electrolysis technology. While small-scale systems can be constructed fairly easily, scaling up to meet industrial demands requires careful planning and robust infrastructure.
- Main Challenges:
- High capital costs for larger systems can deter companies from investing, especially in a market that is still developing.
- The integration of larger units into existing energy frameworks often demands extensive modifications, which can be cost-prohibitive.
As the market matures, strategizing for scalable designs will be essential. Companies must engage in developing modular systems that can grow as demand increases, allowing for flexibility and reduced initial investment.
In summary, while PEM electrolysis is a promising avenue for green hydrogen production, it faces significant limitations. Addressing the cost of materials, durability and lifespan, and scalability issues will be critical for its widespread acceptance and implementation in the energy landscape.
Recent Advances in PEM Electrolysers
The domain of hydrogen production has seen promising strides thanks to developments in PEM (Proton Exchange Membrane) electrolysers. These advancements are not just a flash in the pan; they represent a roadmap for future energy solutions, playing a crucial role in steering both industry and technology towards more sustainable practices. Understanding these recent breakthroughs gives us an edge when evaluating not just the technology itself, but also its relevance in combating climate change and improving energy security.
Novel Membrane Materials
In the realm of PEM electrolysers, the membrane is a vital component, acting as a gateway for protons while serving as a barrier for gases. Recent innovations in membrane materials have focused on enhancing conductivity and durability. Traditional membranes, typically made from Nafion, have limitations in terms of temperature tolerance and moisture retention, impacting efficiency rates. Researchers have explored alternatives, including
- Composite Membranes: Blending several substances provides better mechanical stability while maintaining high ion-conductivity. A promising example includes the combination of polymer with ceramic materials.
- Ionic Liquids: These have emerged as potential candidates due to their negligible vapor pressures and excellent ionic conductivity, even under varied temperatures.
Such advancements are paramount—they not only improve efficiency but also extend the overall lifespan of electrolysers, thereby reducing operation and maintenance costs.
Catalyst Innovations
Catalyst development is essential in ensuring the efficiency of electrochemical reactions during hydrogen production. A notable recent advance has been the focus on non-noble metal catalysts, which proffer both cost-effectiveness and comparable performance to their platinum-based counterparts. Researchers have made headway in developing:
- Transition Metal Catalysts: Using metals like nickel, cobalt, and iron—less expensive and more abundant—has led to more sustainable production processes.
- Nanostructured Catalysts: Enhancements in particle size and surface area can lead to improved activity and resilience, offering superior performance at lower loadings.
These developments can significantly reduce costs associated with hydrogen production, making it more accessible for various industries.
Integrated Systems with Renewable Energy
The coupling of PEM electrolysers with renewable energy sources is a game-changer in the quest for sustainable hydrogen production. By integrating systems such as:
- Solar Power: Utilizing photovoltaics to supply electricity during peak sunlight hours can create a synergy that maximizes both hydrogen yield, while effectively leveling off energy supply and demand.
- Wind Energy: Wind generation can be harnessed in tandem, especially in regions with abundant wind. This compatibility allows for the generation of hydrogen during high production periods, offering an immediate use for excess energy.
This integration not only optimizes the efficiencies of both the electrolysers and the energy sources but also aligns with global efforts to utilize clean energy, positioning PEM electrolysis at the forefront of sustainable energy solutions.
These advancements in PEM technology not only usher in a new wave of efficiency and cost-effectiveness but also lay down the groundwork for a robust hydrogen economy, which plays a crucial part in reducing global carbon emissions.
These advancements in PEM technology not only usher in a new wave of efficiency and cost-effectiveness but also lay down the groundwork for a robust hydrogen economy, which plays a crucial part in reducing global carbon emissions.
Applications of PEM Electrolysers
PEM electrolysers are more than just a technological curiosity; they stand at the heart of the hydrogen economy's current and future applications. Understanding their versatility in various sectors is crucial for grasping their role in sustainable energy systems. The growth of hydrogen production technology, particularly through electrolysis, is driving momentum in numerous industries, making PEM electrolysers an essential focal point in today's energy landscape.
Hydrogen Production for Fuel Cells
One of the primary applications of PEM electrolysers is in the production of hydrogen that serves as a fuel source for cells. Fuel cells operate on the principle of converting hydrogen and oxygen into electricity, with water as the only byproduct. This clean energy transfer adds invaluable benefits to transportation and portable power generation. By utilizing PEM technology, hydrogen production from water is efficient and scalable, meeting the dynamic demands of fuel cell technologies.
For instance, companies like Toyota are innovating in the automotive realm by integrating hydrogen fuel cells into their vehicles, which greatly reduces greenhouse emissions compared to conventional combustion engines. Notably, hydrogen fuel cell vehicles, such as the Toyota Mirai, have demonstrated efficacy in long-range travel, offering a sustainable alternative to battery electric cars.
Industrial Chemical Production
Beyond transportation, PEM electrolysers are pivotal in industrial chemical processes. Hydrogen produced via these systems feeds numerous chemical reactions for producing ammonia, methanol, and other synthetic fuels. Ammonia synthesis, for example, is traditionally reliant on fossil fuels, but PEM electrolysis shifts the paradigm towards greener chemical production. The Haber-Bosch process, which combines hydrogen with nitrogen, could dramatically lessen its carbon footprint if powered by renewable energy.
Moreover, companies engaged in industrial ammonia production are recognizing the shift towards decarbonization. Emerging technologies are experimenting with PEM electrolysers to enhance efficiency and reduce operational costs while following stricter environmental regulations. Businesses that adopt such innovations balance economic growth with environmental responsibility.
Energy Storage Solutions
Energy storage presents an evolving challenge in the shift to renewable energy. PEM electrolysers offer a feasible solution by converting excess electricity, particularly from intermittent sources like solar or wind, into hydrogen gas. This stored hydrogen can later be converted back into electricity using fuel cells, effectively functioning as a form of energy storage.
In regions where production exceeds consumption, hydrogen storage could mitigate the negative effects of energy waste. For instance, excess electricity generated during high solar output hours can be transformed into hydrogen, which can then be utilized during periods of low generation or peak demand.
"The shift to using PEM electrolysers for energy storage is not just smart but necessary for balancing our energy systems."
"The shift to using PEM electrolysers for energy storage is not just smart but necessary for balancing our energy systems."
This unique approach creates a bridge between energy production and demand, enabling a more flexible and sustainable energy grid.
Through the utilization of PEM electrolysers in these applications, industries leverage cleaner energy solutions while pushing forward towards a sustainable future. The importance of these electrolysers cannot be overstated, as they play a critical role in enhancing operational efficiency and promoting environmentally-friendly practices across various sectors.
Market Trends and Economic Viability
The landscape of hydrogen production is rapidly evolving, and understanding the market trends and economic viability of PEM electrolysers is pivotal for stakeholders in this sector. As we shift towards cleaner energy solutions, the importance of analyzing these trends cannot be overstated. It not only sheds light on the current state of technology but also helps predict its future trajectory, ensuring strategic investments and innovations are made in the right areas.
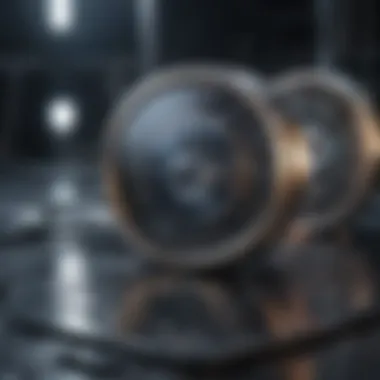
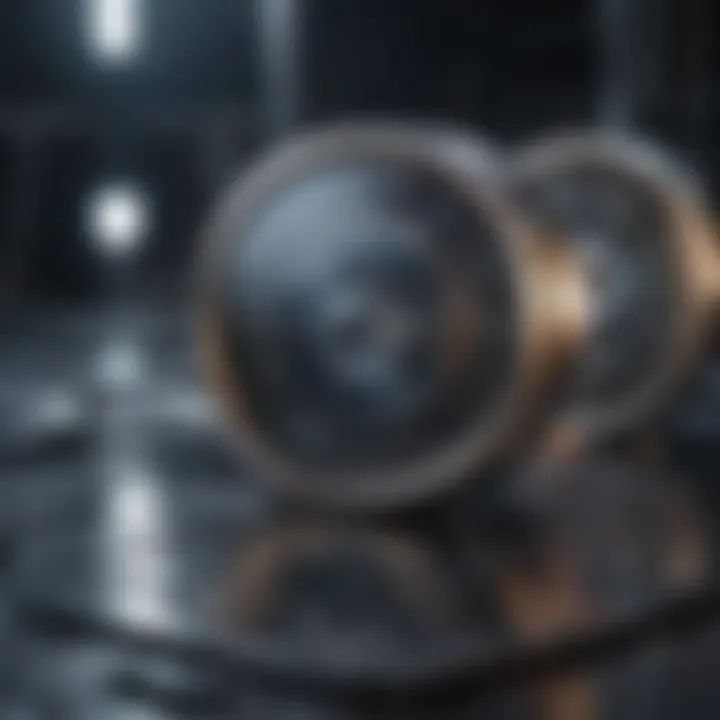
Global Market Insights
The global hydrogen market is witnessing a seismic shift, driven by the increasing demand for clean and sustainable energy sources. Recent research indicates that the hydrogen market is projected to reach over $200 billion by the end of the decade, driven largely by advancements in PEM technology. The rise of green hydrogen—produced via electrolysis powered by renewable energy—has garnered significant attention, as countries aim to reduce their carbon footprints while meeting energy demands.
Some key market insights include:
- Government Policy Support: Various governments are ramping up support for hydrogen initiatives, offering incentives and funding for research and development. For instance, the European Union's Hydrogen Strategy outlines plans to scale up production and market development solutions.
- Technological Partnerships: Collaboration between tech companies and energy firms is on the rise, facilitating the sharing of expertise and resources. This trend helps in driving down the costs associated with PEM electrolysers, making them more commercially viable.
- Investment Surge: Institutional and private investments are attracting attention. Sectors such as automotive, chemical production, and energy storage are expanding their portfolios to include hydrogen solutions, thus driving demand for electrolysers.
"The direction of the hydrogen economy is inexorably linked to advancements in PEM technology. The ability to produce hydrogen at scale and economically is what will define its success in the coming years."
"The direction of the hydrogen economy is inexorably linked to advancements in PEM technology. The ability to produce hydrogen at scale and economically is what will define its success in the coming years."
Future Cost Projections
Cost remains a critical factor when evaluating the economic viability of PEM electrolysers. Historically, the high upfront costs associated with these systems have posed barriers to entry for many companies. However, projections indicate a positive trend toward decreasing costs.
Several elements contribute to the anticipated reduction in costs:
- Material Innovations: Advances in the materials used for membranes and catalysts are expected to lower expenses. Companies are investing in alternative materials that maintain efficiency while being cheaper than traditional options like platinum catalysts.
- Economies of Scale: As the market matures and demand for hydrogen grows, production scales will increase. Larger-scale manufacturing of PEM electrolysers will lead to economies of scale, resulting in lower costs per unit.
- Technological Advancements: Continuous research is leading to more efficient designs and better operational parameters. Efficiency improvements directly correlate with output, thus optimizing cost-effectiveness in hydrogen production.
- Market Competition: An influx of new players entering the market increases competition. This competitive pressure encourages innovation and price reductions as companies strive to gain market share.
In summary, as the landscape of PEM electrolysers evolves in response to market trends and economic considerations, stakeholders must stay informed and agile. The interplay between technological innovation, market demand, and regulatory support will be key determinants in shaping the future of hydrogen production and its underlying economic viability.
Environmental Impact of PEM Electrolysis
The environmental influence of any technology, especially one connected to energy production, is a crucial subject that demands scrutiny. This holds particularly true for PEM electrolysis, which plays a significant role in hydrogen production. As the world contemplates the shift toward sustainable energy, the integration of PEM electrolysis into this framework becomes a pressing topic. There are several dimensions to consider when evaluating its environmental impact.
Carbon Footprint Analysis
The notion of carbon emissions is front and center in discussions about energy technology. PEM electrolysis presents a dual-edged sword in this regard. On one hand, the process itself is generally low in carbon output, principally due to its reliance on electricity to split water into hydrogen and oxygen. If this electricity comes from renewable sources, the carbon footprint can be minimal, showcasing PEM's potential to support decarbonization efforts. Conversely, if fossil fuels are the predominant source of electricity, then the environmental benefits become muddled.
A thoughtful analysis reveals that:
- Renewable Energy Synergy: When coupled with wind or solar, PEM electrolysis can achieve nearly zero emissions.
- Lifecycle Assessments: Understanding the carbon footprint isn’t just about direct emissions; it also incorporates manufacturing, transportation, and disposal of electrolyser components.
- Mitigation Strategies: Innovations in energy sourcing and technological improvements may make it possible to lower the overall emissions related to PEM systems, even when powered by conventional methods.
"The most effective strategy for reducing the carbon footprint of PEM systems hinges on the decarbonization of the grid from which they draw power."
"The most effective strategy for reducing the carbon footprint of PEM systems hinges on the decarbonization of the grid from which they draw power."
Sustainability Aspects
When considering PEM electrolysis in the sustainability equation, several aspects warrant attention. It is not solely about output; it's about a broader ecological impact that extends through various stages of deployment and operation.
- Resource Efficiency: PEM electrolysers utilize precious materials, such as platinum or iridium, in their catalysts. Sourcing these metals responsibly can pose a significant environmental challenge. However, researchers are striving to develop more efficient catalysts that minimize or even eliminate the need for these materials.
- Water Use: Water—the input for electrolysis—is abundant but should be managed wisely. Ensuring that the water extracted for hydrogen production does not compromise local ecosystems is essential.
- Long-term Viability: Another critical aspect includes durability and maintenance. The longevity of PEM technology reduces waste and resource input over time. Enhancing durability through improved materials and design can therefore promote sustainability.
In summary, the path forward for PEM electrolysis is not just about production methods but integrating intelligent design and resource management strategies that can foster an environmentally friendly legacy for this promising technology.
Future Outlook for PEM Electrolysers Technology
The future of PEM electrolysers poses significant implications for the hydrogen economy and the broader landscape of renewable energy. With global emphasis on decreasing carbon emissions and turning toward sustainable energy sources, this technology stands at the forefront of innovation. The journey from traditional hydrocarbon-based energy systems to renewable alternatives is being accelerated by advancements in PEM electrolyser technology. The projections are optimistic, focusing on enhancing efficiency, cost-effectiveness, and overall performance, all while addressing existing challenges.
Research Directions
As researchers delve into improving the performance of PEM electrolysers, numerous pathways emerge that are both promising and practical. Several aspects are worth considering:
- Material Innovation: Developing advanced membrane materials that offer higher proton conductivity and better durability can significantly enhance performance. Researchers are exploring polymers and composites that can withstand harsh operational environments.
- Catalyst Development: Improving catalysts, especially in terms of reducing reliance on precious metals like platinum, is crucial. The search is on for alternative materials that are both effective and economical.
- System Integration: Understanding how PEM electrolysers can seamlessly integrate with various renewable energy sources is vital. Simulating systems that can operate efficiently with fluctuating energies from solar or wind can revolutionize the market.
- Scalability Studies: New methodologies for scaling up production without losing efficiency or increasing costs are in demand, focusing on modular designs that allow for one-size-fits-all solutions.
- Pilot Projects and Demonstrations:
Real-world applications must be tested through pilot projects to analyze the effectiveness and practicality of emerging technologies.
These research trends indicate that the future will likely see a range of transformative changes and improvements, significantly affecting the hydrogen production landscape.
Integration into Energy Systems
Integrating PEM electrolysers into existing energy frameworks isn’t merely a trend; it’s a necessary evolution. Several elements must be considered to achieve effective integration:
- Renewable Energy Synergy: PEM electrolysers should work hand in glove with renewable energy sources. This calls for innovative strategies that ensure optimal energy use even when renewable inputs fluctuate. For instance, using surplus wind energy during off-peak hours could maximize efficiency.
- Grid Support and Stability: By acting as flexible loads, PEM systems can provide grid stability, consuming excess energy when available while deferring production during peak demand.
- Hydrogen as a Storage Medium: Hydrogen produced through electrolysis can serve as an energy storage medium. This ties into broader energy systems, representing a cleaner alternative to battery storage and offering prolonged energy sustainability.
- Collaborative Models: Working alongside industrial entities, utilities, and research institutions will create diversified ecosystems that enhance the performance and applicability of PEM electrolysers.
In summary, the future of PEM electrolysis looks promising and multifaceted. Research directions are exploring innovative materials, catalysts, and integration approaches that can align this technology with energy systems globally. As developments unfold, PEM electrolysers may well become a cornerstone in achieving a sustainable energy future.
Culmination
The discussion surrounding PEM electrolysers highlights their critical role in the broader hydrogen economy. Recognizing the need for sustainable energy solutions has only intensified interest in these technologies. The examination of their operational principles, advantages, and challenges paints a vivid picture of why PEM electrolysers are not just another technological novelty, but rather a cornerstone of future energy systems.
Summary of Key Points
- Operational Mechanics: The basic workings of PEC electrolysers rely on straightforward yet effective electrochemical reactions to yield hydrogen from water. When electrical current is applied, water splits into oxygen and hydrogen, emphasizing efficiency and a sustainable process.
- Advantages over Conventional Methods: Among the notable benefits, the compact design, rapid response times to load changes, and overall higher efficiency set PEM technology apart from traditional electrolyser models. The ability to quickly adjust output makes them suited for integration with renewable energy sources.
- Challenges and Innovations: Despite their evident advantages, cost of materials and durability issues cannot be overlooked. This article also anticipates the path forward, spotlighting research into new materials and integrated systems that promise to ameliorate current limitations.
- Market Dynamics and Future Prospects: Understanding the market trends leads to significant insights about potential growth areas. As the demand for hydrogen production soars, the viability of PEM electrolysers looks promising, particularly with projections suggesting a reduction in future costs.
- Environmental Considerations: Lastly, assessing the environmental impact speaks volumes about the role these electrolysers play in sustainable development. As society pushes towards less carbon-intensive energy systems, PEM electrolysis offers a route to achieve those goals.
Implications for Future Energy Landscapes
The implications of this technology in future energy systems extend far beyond mere hydrogen production. As energy demands evolve and pressure mounts to transition away from fossil fuels, PEM electrolysers present a viable solution.
- Energy Storage Potential: PEM electrolysis provides a method for balancing intermittent renewable energy sources. By storing energy in the form of hydrogen, it helps to manage supply and demand effectively.
- Fuel Cell Applications: The hydrogen generated is a vital component for fuel cells, essential for transportation and stationary power applications. This ties deeply into broader strategies for decarbonizing sectors traditionally dependent on fossil fuels.
- Public Policy and Economic Incentives: Achieving a stable energy future requires not just technological advances but robust policy frameworks that support these innovations. Policymakers will need to create incentives that foster research, development, and eventual deployment of PEM electrolysis technology across various industries.
- Collaborative Efforts: Partnerships between private and public sectors can catalyze advancements. Engaging in collaborative projects appears essential to accelerate progress, driving down costs and enhancing efficiency.
- Role in a Circular Economy: Lastly, as we shift towards sustainability, PEM electrolysers can help establish a circular economy approach in energy usage, contributing to resource efficiency and environmental preservation.
The horizon of our energy landscape is shifting, and PEM electrolysis stands at the forefront, paving the way for a transitional phase to greener methods. This intricate dance between technology, policy, and society will shape not only how we generate energy but also how we consume and view resources in the future.
"The future of energy isn't just about new technologies; it's about integrating them into our existing frameworks to inspire real change."
"The future of energy isn't just about new technologies; it's about integrating them into our existing frameworks to inspire real change."
By grasping the nuances of PEM electrolysis, we arm ourselves with understanding necessary to navigate future challenges. Whether through academic inquiry, industrial application, or policy-making, knowledge is our key asset in this rapidly evolving field.