Understanding Restriction Enzyme Recognition Sites
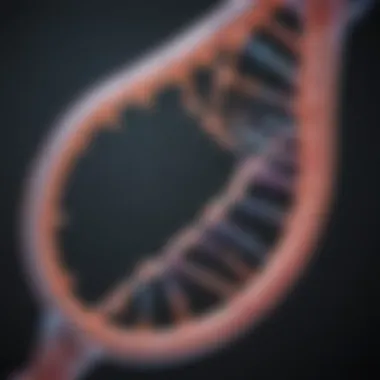
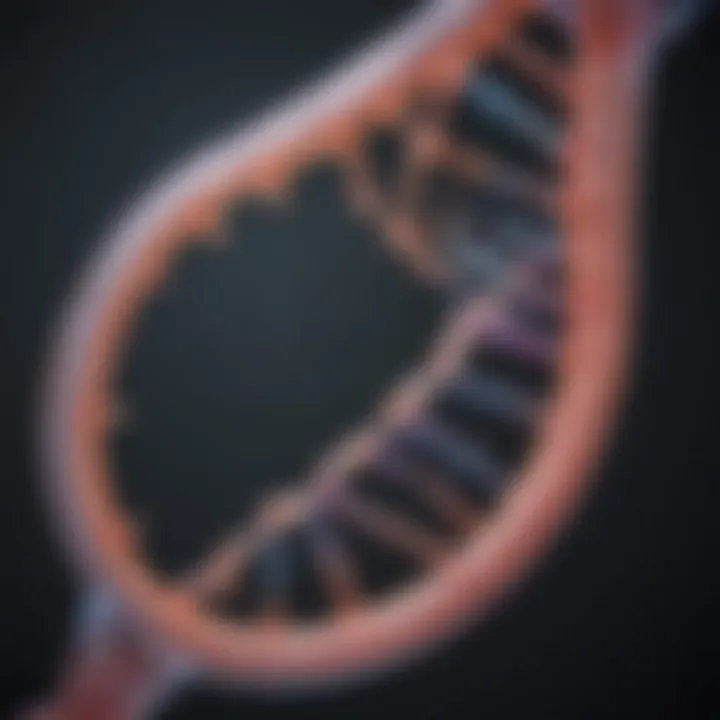
Intro
Restriction enzymes, also known as restriction endonucleases, are essential tools in molecular biology and biogenetics. They are proteins that identify and bind to specific sequences within a DNA molecule, causing cleavage at or near these sites. The focus of this article is to delve into the intrinsic qualities of restriction enzyme recognition sites, their structural characteristics, and their significance in contemporary genetics and biotechnology.
These enzymes play a crucial role in molecular cloning, genetic engineering, and gene editing. A deeper understanding of these recognition sites is vital, as it informs researchers about how these enzymes can be applied effectively in laboratory and clinical settings. This knowledge also opens up pathways to advance genetic research and therapeutic developments.
Key Concepts
Definition of the Main Idea
The concept of restriction enzyme recognition sites can be simplified as defined segments of DNA where specific enzymes bind and subsequently act. Each restriction enzyme recognizes a unique sequence, typically ranging from four to eight base pairs. This specificity is what makes these enzymes valuable in various applications, such as DNA analysis, cloning, and gene manipulation.
Overview of Scientific Principles
When a restriction enzyme encounters its target sequence in DNA, it binds and cleaves the DNA at specific points. This cleavage results in two fragments with either sticky ends or blunt ends, depending on the enzyme used. Sticky ends have complementary overhangs, while blunt ends do not. The ability to cut DNA at precise locations is what allows scientists to insert new genetic material, delete unwanted sequences, or create specific alterations within a genome. Understanding the underlying principles of these enzymes enriches the knowledge base that molecular biologists and geneticists rely upon.
Current Research Trends
Recent Studies and Findings
Recent research highlights strides in understanding the efficiency and specificity of different restriction enzymes. Studies have employed various methods, such as next-generation sequencing, to analyze the outcomes of DNA manipulations. Researchers continually assess new enzymes derived from diverse organisms, leading to the discovery of more effective tools for targeted genomic interventions.
Significant Breakthroughs in the Field
One breakthrough includes the discovery of CRISPR-Cas9, a revolutionary genome-editing tool that works along similar principles to restriction enzymes. Despite being different in mechanism, the analogies drawn between CRISPR and restriction enzymes showcase how historical understanding of sequence recognition has paved ways for modern innovations in genetic engineering. Additionally, advancements in enzyme engineering have allowed scientists to tailor restriction enzymes to recognize novel sequences, thus expanding their applicability in various biotechnological therapies.
Prologue to Restriction Enzymes
Restriction enzymes, also known as restriction endonucleases, are significant tools in molecular biology. Their ability to accurately target specific DNA sequences makes them invaluable in genetic engineering. The importance of this topic is multifaceted, focusing on the precision they bring to DNA manipulation. Understanding how these enzymes function allows researchers to innovate in fields such as cloning, gene therapy, and synthetic biology.
The recognition sites within DNA that restriction enzymes target are of utmost relevance. These sites dictate the effectiveness of the enzyme's action, influencing how genetic material can be modified or edited. Furthermore, recognizing the structure and characteristics of these sites aids in predicting the behavior of different enzymes during experiments.
Moreover, the development of these enzymes over time highlights the ongoing advancements in biotechnology. Early discoveries paved the way for improved techniques that have diverse applications in modern science. For educators, students, and professionals in biology, comprehending the intricacies of restriction enzymes enhances critical thinking and fosters innovation in experimental designs.
Understanding restriction enzymes establishes a fundamental basis for deeper exploration into genetic manipulation and biotechnological advancements, which shape the future of research.
Definition and Function
Restriction enzymes have a clear definition and an essential function in molecular biology. They are proteins produced by bacteria that can recognize short, specific sequences of DNA. Upon binding to these recognition sites, they catalyze the cleavage of the DNA strand. This process effectively separates DNA into fragments, enabling further manipulation for research or therapeutic purposes.
Enzymes such as EcoRI and BamHI are examples that recognize certain sequences and cut the DNA at specific locations. This selective action allows scientists to splice desired genes into plasmids or remove genetic material in precise ways. Therefore, their function goes beyond mere cutting; it fundamentally influences gene expression and protein synthesis.
Historical Development
The historical development of restriction enzymes outlines a fascinating journey in biotechnology. Their discovery in the 1970s brought about a significant revolution in genetics. The first major restriction enzyme identified was HindII, isolated from the bacterium Haemophilus influenzae. This initial discovery opened new avenues for genetic research and laid the groundwork for recombinant DNA technology.
Over the next decades, a diverse range of restriction enzymes were discovered. Researchers identified enzymes that cut at different sequences and with varying precision. This diversification has led to the establishment of a wide toolkit for genetic engineering. Consequently, the historical trajectory of these enzymes reflects the curiosity and ingenuity of scientists aiming to manipulate life's genetic blueprint.
Mechanism of Action
The Mechanism of Action of restriction enzymes is a fundamental aspect of their function and utility in molecular biology. Understanding how these enzymes bind to specific DNA sequences and cleave them is crucial. This knowledge not only helps in manipulating genetic material but also aids in the development of various applications in biotechnology. Each step in the mechanism provides insights into how to effectively use these enzymes in laboratory settings. Moreover, recognizing the factors that influence their activity can significantly optimize experimental conditions and outcomes.
Binding to Recognition Sites
Binding is the first step in the action of restriction enzymes. These enzymes recognize specific sequences within the DNA, known as recognition sites. The recognition sites are generally short, ranging from four to eight nucleotides in length, and can vary widely among different restriction enzymes.
Once a restriction enzyme encounters its target sequence, it binds to this site with high specificity. This specificity is what makes restriction enzymes such powerful tools in molecular biology. Their ability to identify precise sequences means that they can be used to cut DNA at exact locations, enabling a variety of genetic manipulations. The binding affinity also ensures that the enzyme does not mistakenly act on other regions of the DNA, minimizing potential errors in experiments.
Cleavage Activity
Following successful binding, the restriction enzyme performs its primary function: cleavage. The cleavage activity involves cutting the DNA backbone, resulting in the synthesis of free DNA ends. Depending on the enzyme, the cuts may create either blunt ends or sticky ends.
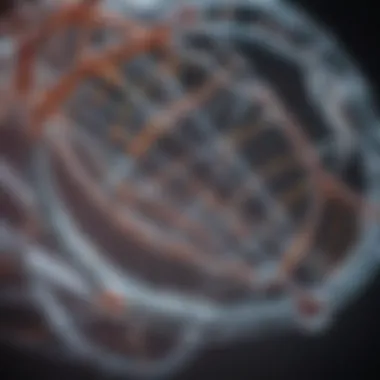
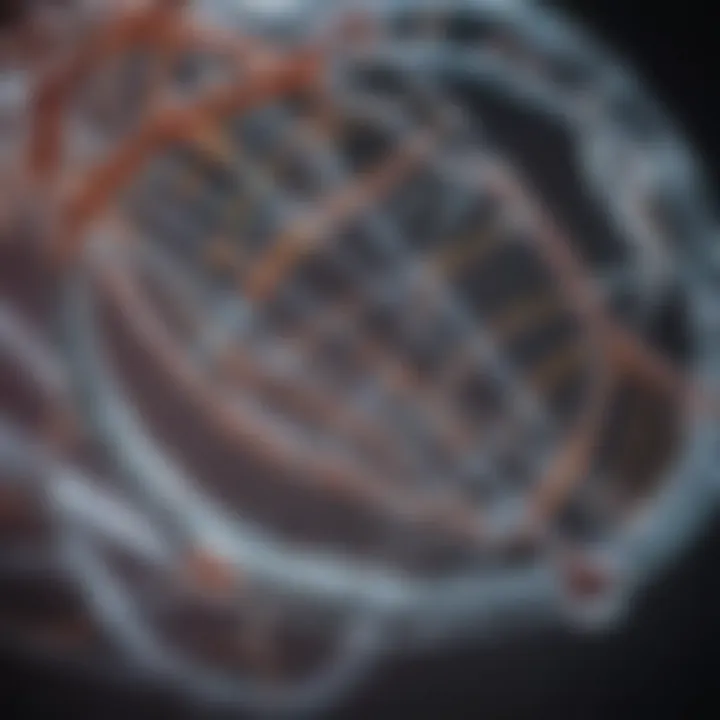
- Blunt ends are produced when the cuts occur straight across the double helix, while sticky ends result from staggered cuts that leave single-stranded overhangs.
- The type of end produced influences the subsequent steps in processes like cloning or ligation, impacting how easily the DNA fragments can be joined with others.
Factors Influencing Activity
The activity of restriction enzymes is not constant; it can be influenced by various factors. Three major factors that can significantly affect enzyme function are Temperature, pH, and Enzyme Concentration. Each of these plays a vital role in optimizing reactions involving restriction enzymes.
Temperature
Temperature significantly affects the reaction environment for restriction enzymes. Each enzyme has an optimal temperature range, typically around 37 to 50 degrees Celsius. High temperatures can increase activity up to a point, but excessive heat can denature the enzyme. Thus, it is essential to maintain the appropriate temperature for maximum activity.
This control allows for enhanced precision in a laboratory setting. Maintaining temperature stability is a common practice in molecular biology labs to ensure reaction efficiency.
pH
The pH of the reaction buffer is another critical parameter. Most restriction enzymes function best at a neutral pH, typically around 7.0. Variations in pH can impact the ionization of the enzyme and substrate, affecting binding and cleavage efficiency. Thus, ensuring the correct pH is necessary to maintain enzyme stability and activity.
Laboratories often use standard buffers designed to keep pH levels constant during experiments, highlighting the common understanding of its importance.
Enzyme Concentration
Enzyme Concentration directly influences the cleavage activity. Higher concentrations can lead to more efficient cuts, but too high a concentration might lead to nonspecific binding to unwanted sites. Thus, finding the right concentration is key to an effective reaction.
Small adjustments in enzyme concentrations can drastically change outcomes in experiments. Typically, optimization processes are performed to determine the optimal concentration for specific applications.
"Understanding the Mechanism of Action of restriction enzymes is vital for any experimental design needing precision in DNA manipulation."
"Understanding the Mechanism of Action of restriction enzymes is vital for any experimental design needing precision in DNA manipulation."
In summary, the mechanism of action for restriction enzymes involves binding to highly specific recognition sites followed by cleavage. Influencing factors like temperature, pH, and enzyme concentration are crucial for optimal enzyme performance. By grasping these concepts, researchers can enhance their experimental outcomes.
Recognition Sites: An Overview
Restriction enzymes function by targeting specific sequences of DNA, known as recognition sites. Understanding these sites is crucial, as their specificity determines how effectively these enzymes can accomplish their role in molecular biology. Recognition sites play a pivotal role in applications such as cloning, gene editing, and synthetic biology. Knowledge of these sites enhances our ability to manipulate DNA for research and practical applications.
Definition of Recognition Sites
Recognition sites are short sequences of nucleotides within the DNA where restriction enzymes bind and induce cleavage. Each enzyme is usually specific to a particular recognition site, which is often a sequence of 4 to 8 base pairs long. These sites can either be palindromic or non-palindromic, affecting how enzymes interact with DNA strands. The precise nature of these sequences ensures targeted cuts, allowing scientists to manipulate genes with accuracy.
Types of Recognition Sites
There are primarily two types of recognition sites that restriction enzymes interact with: palindromic sequences and non-palindromic sequences.
Palindromic Sequences
Palindromic sequences are two-fold structures where the sequence of nucleotides reads the same forward and backward on complementary strands. This inherent symmetry is crucial for the binding of restriction enzymes, as it provides an effective anchoring point for enzyme interaction. One key characteristic of palindromic sequences is their reliability in creating defined cuts in DNA. This reliability makes palindromic sequences a favored choice in laboratory settings for manipulating genetic material.
Benefits of Palindromic Sequences
- High specificity for enzyme activity.
- Ease of design for cloning vectors.
- Reduced chances of off-target effects, enhancing experimental accuracy.
While palindromic sequences offer many advantages, they might limit the range of usable target sites in a given genome due to their relatively specific nature. Nonetheless, their predictable behavior in enzymatic reactions greatly aids in genetic manipulation efforts.
Non-Palindromic Sequences
Non-palindromic sequences are those that do not exhibit symmetry. Such sequences are recognized by some restriction enzymes, leading to a more diverse set of possible recognition sites. The diverse nature of non-palindromic sequences allows for a range of cutting patterns within DNA, expanding the tools available for genetic engineering.
Benefits of Non-Palindromic Sequences
- Broader range of target sites, allowing flexibility in gene editing.
- Facilitates unique cutting patterns that can be advantageous in specific applications.
However, non-palindromic sequences can sometimes lead to less predictable enzyme activity. The variability in recognition might complicate experimental designs in certain instances. Nonetheless, the incorporation of non-palindromic recognition sites enhances the toolkit available to researchers, providing a richer landscape for exploration in molecular biology.
Identifying Recognition Sites
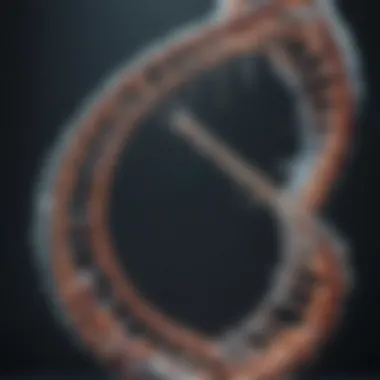
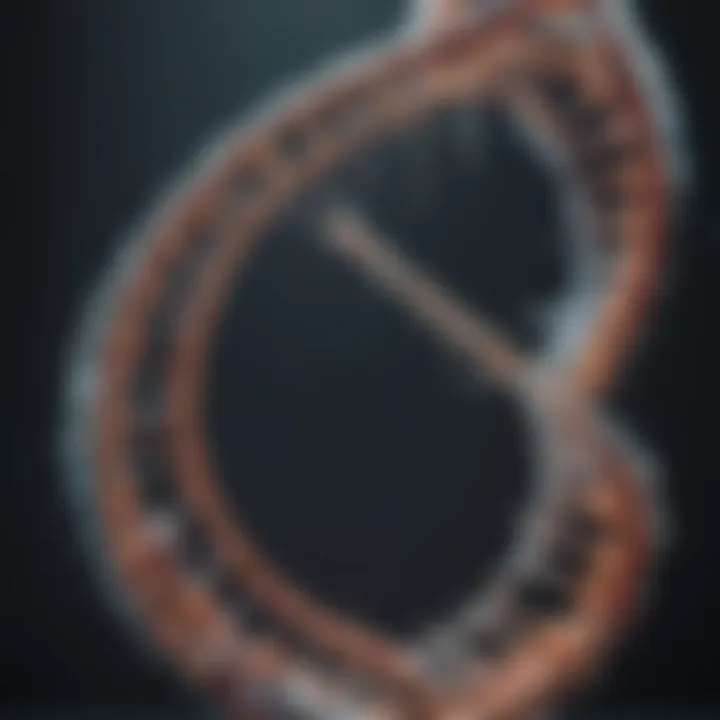
Identifying recognition sites is a critical component in understanding how restriction enzymes function. These sites are specific sequences in DNA where enzymes exert their action, leading to cleavage. Without a deep grasp of how to identify these sites, researchers might struggle with effective cloning and genetic engineering. Accurate identification of these sites can significantly enhance the efficiency of experiments, minimizing time and resource expenditure. Moreover, the ability to locate these sites helps in designing experiments that utilize restriction enzymes effectively.
In Silico Analysis
In silico analysis refers to the use of computer software to predict and analyze recognition sites within DNA sequences. This method provides a powerful tool for researchers, allowing them to quickly identify potential binding sites for various restriction enzymes in any given DNA sequence. Tools such as bioinformatics software develop specific algorithms designed to analyze DNA sequences and predict where enzymes might cleave.
This approach is beneficial because it saves both time and effort compared to traditional lab techniques. On the downside, predictions can sometimes lack accuracy, so validation through experimental methods remains essential to confirm any findings observed in silico.
Experimental Techniques
Restriction Mapping
Restriction mapping is a classic technique used to determine the locations of specific restriction enzyme recognition sites within a DNA fragment. By digesting DNA with various restriction enzymes, researchers can generate a unique pattern of fragments whose sizes can be analyzed. These size patterns help in constructing a map of where the cleavage events occur, providing insight into the DNA structure.
One key characteristic of restriction mapping is its simplicity in design. It requires minimal specialized equipment, making it accessible for many laboratories. Furthermore, it provides direct experimental validation, which is crucial for confirming results from in silico analyses. However, the main disadvantage is that it can be time-consuming, especially when dealing with large DNA samples or multiple enzymes.
Gel Electrophoresis
Gel electrophoresis is a technique widely used to separate DNA fragments based on size. It plays a vital role in analyzing the products of restriction enzyme digestion. By running DNA samples through an agarose gel, researchers can visualize the size of the fragments generated during restriction mapping. This visualization helps confirm the presence and location of the recognition sites.
The key characteristic of gel electrophoresis is its ability to effectively resolve a wide range of DNA sizes, allowing detailed analysis of fragments. It is a preferred choice due to its straightforward implementation and clear results. However, a limitation of this technique is that interpretation can be subjective, and it requires calibration against a DNA ladder for accurate size determination.
Understanding these techniques is fundamental for researchers in molecular biology, enhancing their ability to manipulate and study genetic material explicitly.
Applications in Molecular Biology
Restriction enzymes, through their recognition sites, have transformed the landscape of molecular biology. They form the backbone of several biotechnological applications, enabling precise manipulation of genetic material. Below, we will explore three primary applications where these enzymes are indispensable: cloning and gene manipulation, synthetic biology, and gene therapy.
Cloning and Gene Manipulation
Cloning is the process of creating identical copies of DNA fragments. Restriction enzymes facilitate this process by enabling scientists to cut DNA at specific recognition sites. This precise cutting allows for the insertion of desired genes into vectors, such as plasmids. For example, the enzyme EcoRI recognizes the palindromic sequence GAATTC and cuts it to enable the insertion of foreign DNA. This technique has numerous applications in research and medicine.
By inserting genes of interest, researchers can produce proteins or study gene functions in a controlled environment. The ability to manipulate genes at specific sites ultimately leads to breakthroughs in functional genomics and helps in developing genetically modified organisms (GMOs). It is important to understand that the choice of restriction enzyme influences the efficiency and accuracy of cloning. Therefore, selecting the appropriate enzymes based on recognition sites is crucial in gene manipulation processes.
Synthetic Biology
Synthetic biology integrates the principles of engineering and biology to design and construct new biological parts or systems. In this context, restriction enzymes serve as essential tools for assembling composite genetic elements. Through their specificity for recognition sites, these enzymes allow the construction of new biosynthetic pathways or circuits by cutting and recombining DNA fragments from various organisms.
The power of restriction enzymes in synthetic biology is evident in the creation of biosensors, metabolic pathways, or even whole organisms programmed to perform particular functions. This approach relies on the ability to tailor genetic constructs using specific recognition sites to achieve desired outcomes. Notably, scientists can engineer bacteria that produce biodegradable plastics or biofuels, showcasing the potential of restriction enzymes in addressing contemporary challenges.
Gene Therapy
Gene therapy is an innovative approach that aims to treat or prevent diseases by directly modifying a person's genes. Here, restriction enzymes play a significant role in the delivery and integration of therapeutic genes into target cells. By using enzymes like Cas9, derived from the CRISPR system, researchers can precisely edit genes at designated recognition sites to correct genetic disorders.
The application of restriction enzymes in gene therapy holds great promise, particularly for genetic conditions like cystic fibrosis or muscular dystrophy. However, it also raises ethical considerations surrounding the long-term effects and potential off-target activities. Gene therapy remains a rapidly evolving field, and the continued study of restriction enzymes and their recognition sites will likely lead to new treatments and strategies for combating inherited diseases.
The precision of restriction enzymes in targeting specific DNA sequences has paved the way for advanced applications in molecular biology, making them indispensable tools in genetic engineering.
The precision of restriction enzymes in targeting specific DNA sequences has paved the way for advanced applications in molecular biology, making them indispensable tools in genetic engineering.
By weaving these applications into the fabric of modern science, restriction enzymes are proving to be a key player in the ongoing exploration of genetics and molecular biology. As research progresses, the implications for cloning, synthetic biology, and gene therapy continue to expand, leading to new discoveries and innovations.
Significance in Biotechnology
Restriction enzymes play an essential role in biotechnology. Their ability to recognize and cleave specific DNA sequences allows scientists and researchers to manipulate genetic material with a high degree of precision.
In agricultural biotechnology, restriction enzymes enable the introduction of new traits into crops. For instance, scientists can create transgenic organisms that exhibit desirable characteristics such as enhanced resistance to pests or improved nutritional content. These advancements help to increase food security and sustainability in agricultural practices.
- Improved Crop Varieties: Through genetic transformation, crops can be engineered to withstand environmental stresses.
- Increased Yield: Genetically modified organisms (GMOs) can contribute to higher productivity in farming.
In medical biotechnology, restriction enzymes have transformative applications as well. They facilitate the development of therapeutic agents by allowing precise modifications of DNA.
- Gene Cloning: Researchers can clone genes responsible for diseases, which aids in understanding pathologies.
- Vaccine Development: Restriction enzymes play a crucial role in the creation of subunit vaccines, helping to fight infectious diseases.
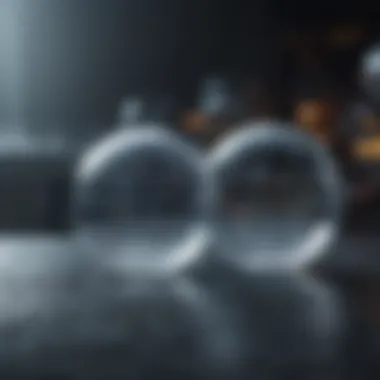
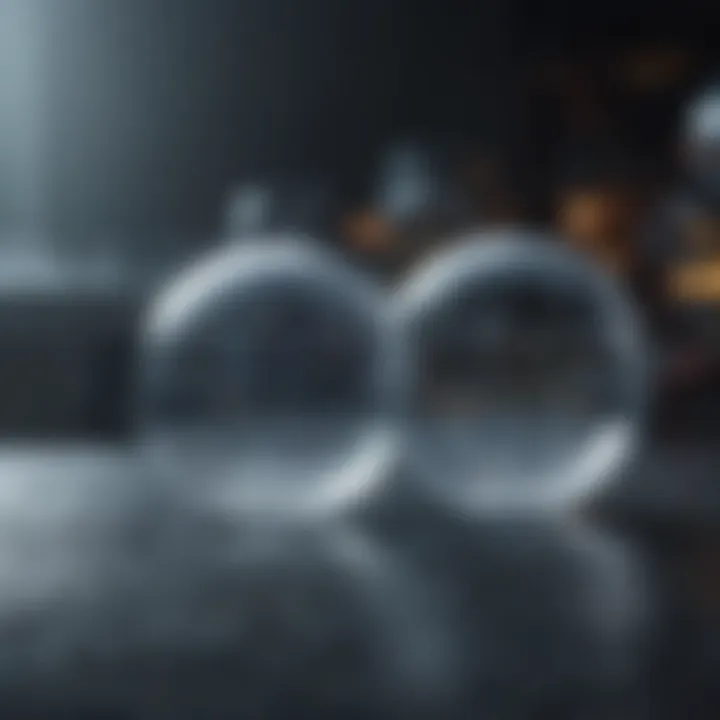
"The precision of restriction enzymes has revolutionized genetic engineering, allowing targeted manipulation of genomic sequences in various fields of biotechnology."
"The precision of restriction enzymes has revolutionized genetic engineering, allowing targeted manipulation of genomic sequences in various fields of biotechnology."
The significance of restriction enzymes extends far beyond academic research. They are embedded in commercial processes that yield diagnostic tools, therapeutics, and genetically modified organisms. The biotechnology sector relies on these enzymes for innovation, thus driving both scientific advances and economic growth.
Agricultural Biotechnology
In the realm of agricultural biotechnology, restriction enzymes are instrumental in genetic modification processes. They enable the isolation and integration of specific genes that confer beneficial traits to plants.
Farmers benefit from these innovations through enhanced crop resilience, which directly impacts yield stability. For example, resistance to pests can be achieved through the introduction of bacterial genes, such as those from Bacillus thuringiensis. This family of insecticidal proteins allows for reduced reliance on chemical pesticides.
Moreover, restriction enzymes facilitate the transfer of beneficial traits across species, broadening the genetic pool available for enhancement. Such capabilities are vital in adapting crops to changing climates and pests, further ensuring food security in the future.
Medical Biotechnology
In the medical sector, the significance of restriction enzymes cannot be overstated. They are essential for genetic research, enabling the exploration of genes linked to diseases. Understanding the genetic basis of conditions allows for the development of targeted treatments.
Restriction enzymes also play a role in gene therapy, which aims to correct genetic disorders by inserting normal genes into a patient's cells. This technique could potentially alleviate symptoms of conditions like cystic fibrosis and hemophilia.
Additionally, the role of restriction enzymes in developing biopharmaceuticals is growing. Enzymes are utilized to create recombinant proteins that serve as hormones, enzymes, or antibodies in treatments. The consistent activity and specificity of restriction enzymes ensure that these proteins are produced accurately and efficiently, paving the way for more effective therapies.
Future Directions
The topic of future directions in the field of restriction enzymes and their recognition sites is essential for understanding ongoing advancements in biotechnology. As researchers continue to explore and manipulate these enzymes, several trends and innovations are emerging.
Designer Enzymes
Designer enzymes represent a significant leap in the application of restriction enzymes. Unlike natural enzymes, designer enzymes are engineered for specificity and efficiency. They can be tailored to recognize unique DNA sequences that natural enzymes might overlook. This customization opens doors for more precise genetic modifications. The benefits of designer enzymes include reduced off-target effects and improved yields in cloning and gene editing applications. Researchers employ techniques such as directed evolution and rational design to create these specialized enzymes.
For example, a designer enzyme could be specifically constructed to target a harmful mutation in a gene, allowing for precise repair. This functionality is not only valuable in research but also holds promise in therapeutic applications. The potential applications span from agricultural improvements to targeted cancer therapies.
CRISPR and Genome Editing
CRISPR technology has revolutionized the approach to genome editing, and its synergy with restriction enzymes amplifies this impact. While CRISPR itself does not classify as a restriction enzyme, it utilizes principles reminiscent of them. CRISPR operates by providing a precise guide RNA that directs the Cas9 enzyme to a specific DNA sequence for cleavage. This biological response can be enhanced by incorporating restriction enzymes for more versatile genome editing.
The integration of restriction enzymes with CRISPR could streamline the process of inserting genes or deleting unwanted sequences, making it a comprehensive toolkit for genetic manipulation. Researchers are actively exploring how these enzymes can enhance CRISPR's precision and efficiency.
Furthermore, advancements in gene therapies, synthetic biology, and agricultural improvements continue to emerge due to these technologies. The implications for biosafety and ethical considerations are also paramount, urging a conversation around how these powerful tools should be responsibly used.
Ethical Considerations
The topic of ethical considerations in the realm of genetic engineering, particularly concerning the role of restriction enzymes, is of paramount importance. As we advance into an era where manipulating the genetic framework of organisms is becoming routine, ethical implications arise. These include the potential for biodiversity loss, unforeseen ecological impacts, and the moral dilemmas surrounding genetic modifications in human beings. The decisions made today can lead to far-reaching consequences for future generations.
One critical area of focus is biosafety. This refers to the measures needed to ensure that genetic engineering does not pose risks to human health or the environment. Every laboratory must adhere to strict guidelines when working with restriction enzymes and genetically modified organisms. The intentional release of genetically modified organisms into the environment should be approached with caution. The objective is to prevent unintended consequences that could arise from their interactions with native species or ecosystems.
Another significant aspect is the role of regulations in genetic engineering. Governments worldwide have implemented strict regulatory frameworks to oversee genetic experiments. These regulations ensure that all scientific activities involving restriction enzymes are conducted responsibly. They foster a culture of safety and ethical conduct in research settings. Compliance with these regulations not only serves to protect human health and the environment but also reinforces public confidence in biotechnological advancements. Often, regulatory bodies require detailed risk assessments before any genetic modification is approved.
The necessity of ethical considerations in biotechnology cannot be overstated. Our strategies in genetic manipulation must always favor safety and sustainability, ensuring we respect the intricate balance of life on Earth.
The necessity of ethical considerations in biotechnology cannot be overstated. Our strategies in genetic manipulation must always favor safety and sustainability, ensuring we respect the intricate balance of life on Earth.
In summary, the ethical considerations surrounding restriction enzymes extend far beyond scientific curiosity. The balance between innovation and responsibility must be maintained. Ongoing dialogue and collaboration among scientists, policymakers, and the public are needed to navigate the complexities of these issues. This ensures that while we explore the potential of restriction enzymes and genetic engineering, we also safeguard our environment and societal values.
Closure
Summary of Key Points
The pivotal points discussed throughout this article include:
- Definition of Restriction Enzymes: These are specialized proteins that recognize and cut specific DNA sequences.
- Mechanism of Action: Understanding how these enzymes bind to their recognition sites and perform cleavage is foundational for their application.
- Types of Recognition Sites: Recognition sites can be palindromic or non-palindromic, which affects enzyme specificity and functionality.
- Applications and Impacts: From cloning to gene therapy, restriction enzymes have diverse utility in both research and practical scenarios.
- Ethical Considerations: Addressing the ethical implications is fundamental to continuing research and application in genetic engineering.
Final Thoughts on Future Research
Looking ahead, the landscape of genetic engineering is rapidly evolving. Future research may include:
- Advancements in Designer Enzymes: Custom-designed enzymes may enhance specificity and efficacy in genetic manipulation.
- Integration with CRISPR Technology: Combining CRISPR with traditional restriction methods could offer more robust tools for genome editing.
- Biosafety Protocols: As applications expand, developing strong biosafety measures and guidelines will be essential to prevent misuse and ensure public safety.
Overall, delving deeper into restriction enzyme recognition sites paves the way for innovations that could significantly transform our capability to understand and manipulate genetic material in ethical and beneficial ways.