Rubber Conductivity: A Comprehensive Exploration
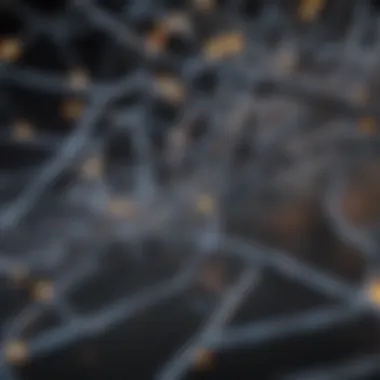
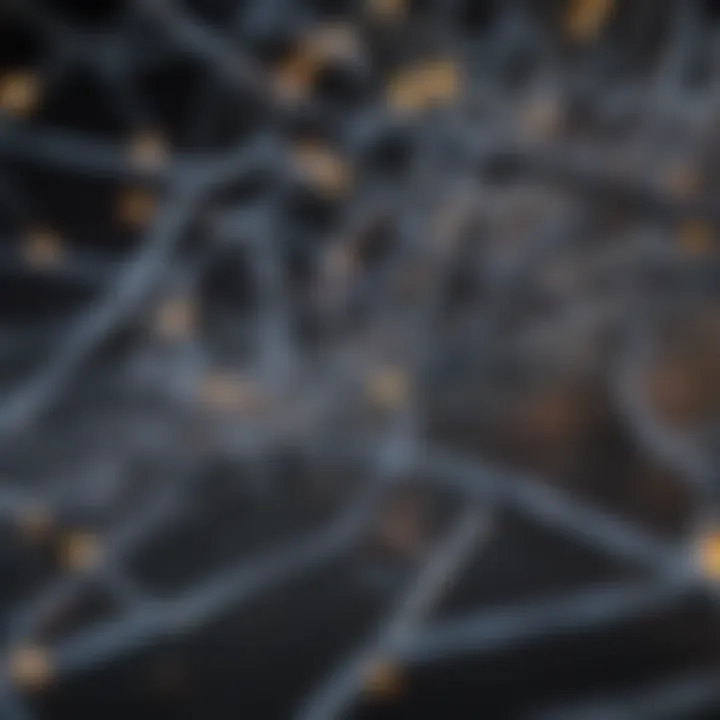
Intro
Rubber, often seen as a mere insulator in the context of everyday usage, holds an intriguing duality in its ability to conduct electricity, albeit under certain conditions. This article embarks on a journey through the less charted waters of rubber conductivity. While some might view rubber as a basic household component—think of tires on a vehicle or the rubber soles of shoes—there's more beneath the surface that begs exploration. Understanding what allows rubber to conduct electricity opens the door to various applications across multiple industries, from automotive to aerospace.
It's essential to recognize that the conductive properties of rubber arise from the complex interplay of its molecular structure and the additives used in its formulation. What may seem simple on the outside often conceals layers of science; thus, we delve into the nuances surrounding rubber's conductivity.
The exploration does not stop at the properties themselves. We will also examine the wider implications, such as how advancements in this field can lead to improved materials that harness the best of both insulating and conductive traits. It’s a fascinating paradox that rubber presents: a material synonymous with flexibility and resistance yet offering potential pathways for electrical conduction.
In this discourse, we'll cover key concepts, current research trends, practical applications, and simmering future possibilities in rubber conductivity. Each aspect will not merely add to a body of knowledge but provoke thought, inviting readers to reconsider the materials we often take for granted.
Prelude to Rubber Conductivity
Rubber conductivity is a topic that blends the realms of material science and electrical engineering. It delves into how certain types of rubber can conduct electricity, which may seem counterintuitive at first, given rubber's well-known insulating properties. Understanding this topic is vital for several reasons. First, it opens the door to innovative applications in modern technology, where conductive rubber can significantly enhance performance and efficiency. Secondly, insights into rubber conductivity can lead to the creation of more effective materials that contribute to sustainability in industries like electronics and automotive. This article seeks to provide clarity and depth on this subject, addressing the components that play a pivotal role in rubber's conductive properties.
Defining Rubber Conductivity
Rubber conductivity refers to the ability of rubber materials to allow the flow of electric current. This characteristic is influenced by several factors, including the type of rubber, the presence of additives like carbon black or metallic particles, and the rubber's overall composition. While pure rubber is typically a poor conductor, when certain materials are blended into the rubber matrix, its conductivity can increase significantly. Understanding these concepts is essential for leveraging rubber in various practical applications, ranging from electronic devices to advanced medical equipment. For instance, conductive rubber could be used to provide a flexible, lightweight connection in wearable electronics without compromising comfort.
Historical Context and Development
The story of rubber conductivity can be traced back to the early experiments with natural rubber, which primarily served as a dielectric material due to its excellent insulating properties. It wasn't until the mid-20th century that researchers began to investigate ways to modify rubber to improve its conductivity. Early advances came from the field of synthetic rubber production, where chemists discovered that incorporating carbon or metal particles into the rubber matrix dramatically enhanced its ability to conduct electricity. Over the decades, this paved the way for the development of various conductive rubber formulations, leading to practical applications in diverse fields.
One notable milestone was in the 1970s when conductive rubber started being used in electronics, largely in the form of gaskets and seals. These materials offered benefits such as flexibility and ease of manufacturing, making them desirable for products that required both electrical contact and environmental protection.
As we moved into the 21st century, research has expanded into understanding how we can manipulate molecular structures to produce rubber with improved conductive properties. This progression highlights the exciting potential for further innovation in conductive materials, spurring ongoing research that incorporates both theoretical and practical elements.
The Science of Rubber
Understanding the science behind rubber is crucial, especially when it comes to its conductivity. This section uncovers how the chemical make-up of rubber, along with its physical attributes, plays a significant role in determining its electrical properties. You can think of rubber not just as a simple elastic material—its unique composition and physical behaviors render it suitable for a variety of applications, particularly where conductivity is a concern. In essence, grasping the nuances of rubber science is foundational to appreciating both its limitations and its potential within many technological realms.
Chemical Composition of Rubber
Rubber is primarily composed of polymers, which are large molecules made up of repeating structural units. The most common type, natural rubber, is derived from latex—a milky fluid extracted from rubber trees like Hevea brasiliensis. This organic composition offers a blend of flexibility, resilience, and—a key point—electrical properties that are somewhat nuanced.
The structure can be modified through chemical processes, resulting in synthetic variants like styrene-butadiene rubber (SBR) and ethylene propylene diene monomer (EPDM). These materials often have distinct conductive properties, influenced by their molecular architecture. Additionally, the presence of fillers, such as carbon black, greatly impacts the overall conductivity. By enhancing the rubber matrix, these fillers facilitate electron movement, transforming a basic insulator into a material with conductive capabilities.
Physical Properties Influencing Conductivity
The relationship between rubber’s physical properties and its conductivity is intricate. Two major factors merit particular attention: elasticity and thermal stability.
Elasticity and its Role
Elasticity refers to the ability of rubber to return to its original shape after being stretched or compressed. This characteristic isn't merely about flexibility; it’s about how these deformations influence the movement of charge carriers within the material. A rubber that exhibits high elasticity can maintain its structure under mechanical stress while allowing for freer movement of electrons.
- Key Characteristic: High elasticity provides resilience, which translates to durability.
- Unique Feature: The ability to stretch and compress significantly impacts the conductive pathways, making elasticity a functional aspect in conductive applications.
- Advantages: When applied correctly, elastic rubber can perform well in various environments, accommodating expansion and contraction without sacrificing conductivity. However, its performance fluctuates under extreme conditions like high temperatures.
Thermal Stability
Thermal stability represents rubber's capacity to retain its properties under varying temperature conditions. This is particularly important, as overheating can cause a breakdown in the material’s structure, leading to significant losses in conductivity.
- Key Characteristic: Materials with high thermal stability can operate effectively in applications where temperature fluctuations are commonplace.
- Unique Feature: Such stability ensures that the conductive pathways remain intact and functional, even under stress.
- Advantages: This reliability can be critical in electronics, where a consistent output is desired. Conversely, thermal instability can result in a loss of conductivity, which is a considerable downside in applications involving high temperatures.
Understanding both elasticity and thermal stability is key in predicting how different types of rubber will perform in real-world applications. The interplay between these properties governs not just the conducting capabilities, but also the longevity and reliability of rubber-based solutions.
Understanding both elasticity and thermal stability is key in predicting how different types of rubber will perform in real-world applications. The interplay between these properties governs not just the conducting capabilities, but also the longevity and reliability of rubber-based solutions.
In this exploration, we see that the science of rubber is not just about its base materials; rather, it’s a tapestry woven from chemical and physical attributes that together determine how rubber can be utilized in conductive applications.
Types of Rubber and Their Conductive Properties
Understanding the different types of rubber and their conductive properties is paramount in grasping how they influence various applications in technology and industry. Rubber, characterized by its flexibility and elasticity, can either be insulating or conductive based on its composition and structure. This section delves into the two main broad categories of rubber: natural and synthetic, along with their specific conductive advantages and disadvantages.
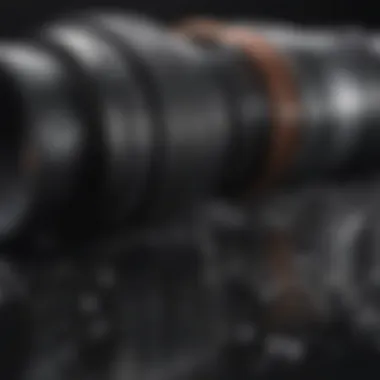
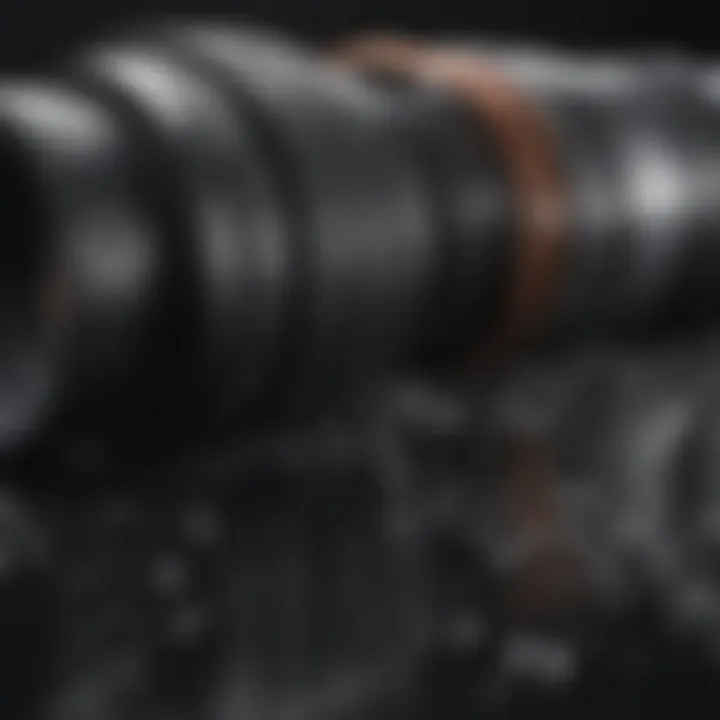
Natural Rubber
Natural rubber, harvested from the latex of rubber trees, boasts impressive resilience and stretchability. Its inherent conductivity is typically lower compared to synthetic counterparts, but certain modifications can improve this aspect. The key characteristic of natural rubber is its high elasticity, allowing it to return to its original shape after deformation. But when it comes to electrical conductivity, natural rubber alone doesn’t often make the cut for many specialized applications.
- Advantages:
- Disadvantages:
- Excellent mechanical strength, which is essential in applications under stress.
- Biodegradable, making it more environmentally friendly.
- Limited conductivity without additives.
- Susceptible to weathering and degradation over time.
To enhance the conductive properties of natural rubber, additives such as carbon black and metal particles are mixed into the compound. These not only improve the conductivity but also maintain the desirable physical qualities of rubber.
Synthetic Rubber Varieties
The development of synthetic rubber varieties has significantly influenced rubber conductivity. These artificial polymers often have tailored properties to suit specific applications. Two particularly notable types are Styrene-Butadiene Rubber and Ethylene Propylene Diene Monomer.
Styrene-Butadiene Rubber
Styrene-Butadiene Rubber, commonly known as SBR, is one of the most widely used synthetic rubbers. Its key characteristic is the blend of both styrene and butadiene monomers, which imparts both durability and flexibility.
- Contributions to Conductivity:
SBR can be formulated to include conductive fillers which enhance its ability to conduct electricity. This flexibility in composition makes SBR a beneficial choice for numerous applications including automotive tires and electrical insulation. - Unique Features:
SBR tends to have superior wear resistance and aging stability compared to natural rubber. However, when used without additives, SBR retains a lower conductivity. - Advantages:
- Disadvantages:
- Excellent abrasion resistance.
- Ability to be produced in large quantities at lower costs.
- SBR may have inferior performance in extreme temperatures compared to other synthetic rubbers.
Ethylene Propylene Diene Monomer
Ethylene Propylene Diene Monomer, often referred to as EPDM, stands out due to its unique key characteristic of enhanced weather resistance and superior elasticity.
- Contributions to Conductivity:
EPDM can be employed in applications requiring not only flexibility but also significant resistance to environmental factors, making it a favorable choice in electrical applications where moisture and temperature can be a concern. - Unique Features:
The incorporation of conductive fillers in EPDM enables it to retain robustness while improving its conduction capabilities significantly. This adaptation has made it a popular choice in various industries, notably automotive and construction. - Advantages:
- Disadvantages:
- High resistance to UV radiation and ozone damage.
- Maintains flexibility at low temperatures.
- More costly than some other rubbers, which could impact production budgets.
In summary, while natural rubber has limitations in conductive properties on its own, modifications can introduce significant advantages. On the other hand, synthetic variants like Styrene-Butadiene Rubber and Ethylene Propylene Diene Monomer provide versatility with options tailored distinctly to enhance conductivity, making them imperative in various applications.
"The choice of rubber type can greatly affect not just performance but longevity and sustainability in practical uses."
"The choice of rubber type can greatly affect not just performance but longevity and sustainability in practical uses."
By grasping these distinctions, engineers and product developers can make informed choices that will impact the efficiency and effectiveness of their designs.
Mechanisms of Conductivity in Rubber
Rubber, while often thought of as an insulator, holds intriguing potential for conductivity under certain circumstances. The study of the mechanisms of conductivity in rubber is critical as it unveils the processes that allow this material to transport electrical charges. Understanding these mechanisms bridges the gap between theoretical explorations and practical applications, making it pivotal for engineers and researchers alike. The interplay of charge carriers, along with the role played by fillers and additives, lays down a foundation for innovating new rubber formulations that can outperform traditional materials.
Charge Carrier Dynamics
The dynamics of charge carriers within rubber materials is a complex and essential aspect of its conductivity. At the core of this dynamic are charge carriers—typically electrons or holes—that move through the rubber matrix when a voltage is applied. Transitions in the rubber's molecular structure can either facilitate or hinder this movement.
- Types of Charge Carriers: In conductive rubber, carriers can be classified mainly into holes (the absence of an electron) and electrons themselves. Their mobility depends significantly on the rubber's inherent properties, e.g., temperature and cross-linking density.
- Temperature Dependence: As temperature increases, the mobility of charge carriers often improves. Therefore, warmer environments can yield different conductive behaviors. This aspect is vital in applications where temperature fluctuations occur, such as in automotive or aerospace sectors.
- Molecular Structure Influences: The arrangement and interaction of the polymer chains can create pathways that either block or allow the passage of these carriers. Rigid structures may reduce conductivity, while flexible and amorphous structures can promote enhanced charge movement.
Ultimately, mastering the dynamics of charge carriers equips manufacturers with the know-how to craft rubber that serves multifunctional purposes in electrical engineering and other fields.
The Role of Fillers and Additives
In the quest to enhance the conductivity of rubber, fillers and additives play indispensable roles. These materials are not merely supplementary; they actively influence the rubber's overall conductive characteristics.
- Types of Fillers: Common fillers include carbon black, metal powders, and conductive polymers. Each serves to improve conductivity by offering alternative pathways for charge carriers.
- Functional Outcomes of Additives: Additives such as plasticizers can modify the rubber's properties to allow more movement within its molecular structure. Such enhancements can lead to increased conductivity, particularly in electrically demanding applications.
- Balancing Conductivity and Mechanical Properties: Striking a balance between improved conductivity and the mechanical integrity of rubber poses a challenge. Overloading rubber with conductive fillers, for example, may lead to a reduction in flexibility or durability. Thus, research continues into optimizing formulations that maintain structural integrity while enhancing electrical properties.
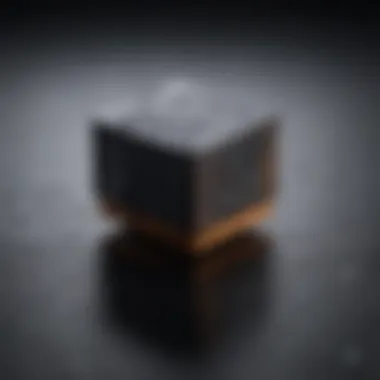
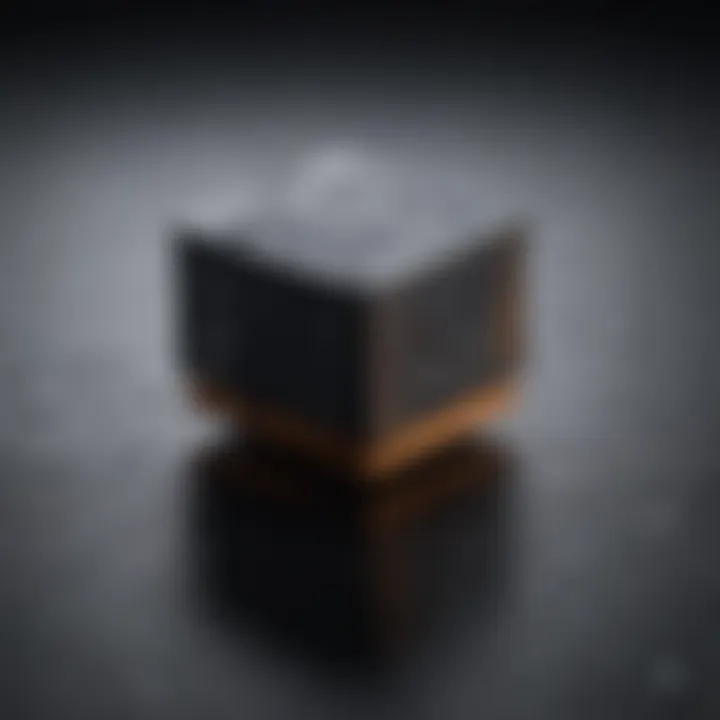
Measurement of Conductivity in Rubber
Understanding how to measure conductivity in rubber is crucial for numerous applications, especially in fields where precise performance is required, such as electrical engineering and material science. The ability to accurately determine conductivity can lead to the optimization of rubber compositions and enhance performance in engineering applications. As rubber is a versatile material with varying compositions, its conductivity can hinge on its type and the additives used, making measurement both a science and an art.
Mastering the measurement of conductivity allows researchers and industry professionals alike to push the boundaries of what rubber can do. For instance, improved methodologies offer insights into the effectiveness of conductive fillers, which can change the material's properties dramatically. Conductivity metrics also play a role in ensuring that rubber products meet safety and performance standards. As the saying goes, "you can't manage what you don't measure," which rings particularly true in this domain.
Instrumentation and Methods
Measurement techniques for rubber conductivity are diverse, driven largely by the material's distinct properties. The primary instruments used include:
- Four-Point Probe Method: This technique involves placing four electrodes in contact with the rubber sample. It helps eliminate contact resistance, providing a more accurate measurement of the sample’s resistance and, thereby, its conductivity.
- Van der Pauw Method: Often used for measuring flat samples, including sheets of rubber. This method requires a uniform thickness and is helpful in determining the conductivity in all four directions of the sample.
- Impedance Spectroscopy: This sophisticated technique measures how a material responds to an applied AC voltage across a range of frequencies. This data is particularly useful for understanding charge carrier dynamics in rubber composites.
When selecting an instrument, the specific application is key. For example, routine quality control may necessitate a different setup compared to in-depth research studies.
Interpreting Conductivity Data
Interpreting the data gathered from conductivity measurements is not merely a matter of looking at numbers; it requires insight into what those figures imply about the rubber's performance. Several factors come into play:
- Conductivity Values: High conductivity values suggest better electron mobility, which is often desirable in applications requiring rapid signal transmission, like in electrical contacts. In contrast, low values indicate insulative properties, suitable for different uses.
- Temperature Effects: The temperature at which measurements are taken can affect conductivity. Thus, understanding these effects is essential when analyzing results and applying them to real-world scenarios.
- Degradation Over Time: Conductivity can change as rubber ages or is exposed to harsh environments. Therefore, long-term conductivity tracking can offer critical insights into material longevity and reliability.
Conductivity metrics provide a window into the essential characteristics of rubber materials, guiding the development of next-generation products with tailored functionalities.
Conductivity metrics provide a window into the essential characteristics of rubber materials, guiding the development of next-generation products with tailored functionalities.
In summary, measuring and interpreting conductivity in rubber isn't simply a technical task—it's a cornerstone of modern material research and application development. Ensuring that appropriate methods are utilized and that results are accurately interpreted can lead to significant advancements in various fields.
Applications of Conductive Rubber
Conductive rubber isn't just a fancy term thrown around in technical circles; it plays a vital role across various sectors. Its unique properties allow it to execute functions that traditional materials often can't. Whether it is to facilitate the flow of electricity, dissipate static, or provide shielding against electromagnetic interference, the diverse applications of conductive rubber are a testament to its versatility. They range from powering intricate electronic devices to enhancing safety in medical instruments, each application bringing distinct advantages and considerations.
In Electrical Engineering
In the realm of electrical engineering, conductive rubber is often considered a silent partner. It finds its place in products like gaskets, seals, and insulating housings, where it not only provides containment but also enables the transmission of electrical signals. The ability to create components that meet strict standards for weather resistance and durability makes conductive rubber invaluable in outdoor installations and industrial applications.
- Electromagnetic Interference (EMI) Shielding: Conductive rubber is widely used in designs needing EMI shielding. The material blocks interference from outside electronic devices, ensuring the integrity of signals in sensitive equipment.
- Flexible Connections: Its elasticity allows for the production of flexible connections in circuit boards. This flexibility leads to better durability, especially in devices that undergo regular use and movement.
Furthermore, components created using conductive rubber can be easily integrated into designs with minimal disruption, transforming traditional electrical systems into more modern, efficient frameworks.
In Consumer Electronics
The consumer electronics space has embraced conductive rubber for a variety of uses, making technology more accessible and user-friendly. Key applications include touchscreen devices, keyboards, and smartphones, where conductive rubber buttons provide tactile feedback while ensuring electrical continuity.
- Touchscreen Interfaces: Conductive rubber pads can be manufactured for touch-sensitive screens, making them effective in reducing wear while ensuring responsiveness.
- Seals and Connectors: Used in connectors and seals for moisture and dust ingress protection, conductive rubber enhances the longevity of devices by keeping unwanted elements at bay.
The popularity of conductive rubber in this sector hinges not just on performance, but also on the potential for cost efficiency. By offering greater lifespan and reduced wear and tear, manufacturers can provide quality products while keeping prices in check.
In Medical Devices
In the medical field, safety and reliability are paramount. Conductive rubber meets these demands effectively, making it a preferred material for several medical devices. From diagnostic equipment to patient monitoring systems, its importance cannot be overstated. The non-toxic nature of many conductive rubber formulations further enhances its suitability for medical use.
- Electrodes: Conductive rubber is often employed in electrodes, found in devices used for diagnostic applications like ECG machines. The elasticity and ability to conform to skin surfaces make them comfortable for patients, while ensuring optimal signal transmission.
- Wearable Health Technology: As the trend towards wearable health devices grows, conductive rubber becomes increasingly relevant. Its flexibility allows for the integration of technology into products designed for continuous use on the human body.
Challenges in Rubber Conductivity Research
Rubber conductivity research faces a labyrinth of challenges that both hinder and inspire further exploration in this important field. Understanding these obstacles is crucial, especially for those engaging in the refinement of rubber materials for various applications. With consistent advances in technology and material sciences, a keen grasp of these challenges can bridge theory with practical application, leading to innovations that enhance rubber’s performance as a conductive material.
Limitations of Current Materials
Despite the strides made in developing conductive rubber compounds, the existing materials still exhibit significant limitations. Traditional rubber types, such as natural rubber, often lack sufficient conductivity without the integration of various fillers or additives. These additives, including carbon black or metal fibers, can change the rubber’s mechanical properties and affect longevity.
For instance, while adding carbon black improves conductivity, it can also lead to a drop in the rubber's elasticity, making it less versatile. This dichotomy poses a delicate balancing act; maintaining flexibility while enhancing electrical performance is not a walk in the park. Additionally, synthetic rubber varieties can also have conductivity issues, as substitutes often introduce new challenges concerning stability and environmental factors.
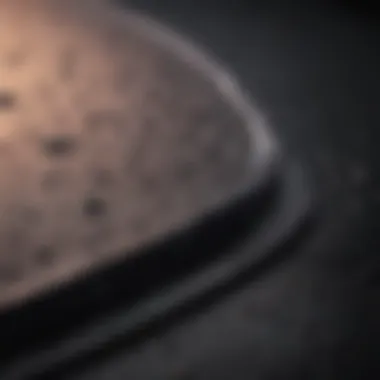
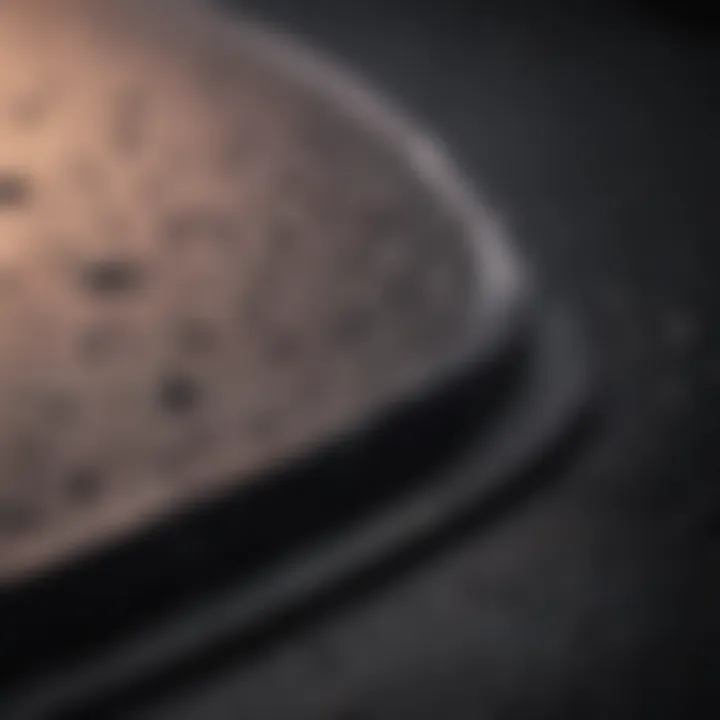
Some researchers suggest a hybrid approach, combining different types of materials to optimize electrical properties while maintaining the necessary physical characteristics. This approach, while promising, is also a complex puzzle that requires detailed experimentation and assessment under different conditions.
Environmental Considerations
While developing conductive rubber, environmental concerns are increasingly at the forefront. The production and disposal of rubber materials can lead to significant ecological footprints. Many conductive fillers can compromise the sustainability of the rubber entirely; substances like heavy metals may enhance performance but pose serious risks for pollution and biosafety.
Moreover, the demand for eco-friendly materials that comply with environmental regulations is rising. As regulations tighten globally, it forces researchers and manufacturers to rethink how conductive rubber is produced and used. The industry is thus collectively leaning towards sustainable practices, including utilizing biodegradable materials or recycling old rubber.
"The adaptation of rubber materials for environmental sustainability is not just a trend; it's a necessity in modern manufacturing.”
"The adaptation of rubber materials for environmental sustainability is not just a trend; it's a necessity in modern manufacturing.”
Combining eco-friendliness with effective conductance requires innovative thinking. Researchers are experimenting with bio-based materials, like natural fibers, that can offer a less harmful alternative while providing acceptable levels of conductivity. However, this shift does bring its own set of challenges in achieving the desired performance levels and ensuring market competitiveness.
In summary, navigating the challenges in rubber conductivity research offers both a pathway for significant advancements in material science and a call to responsibility toward ecological impacts. Understanding limitations and environmental considerations is critical for researchers aiming to innovate and enhance conductive rubber properties effectively.
Future Directions in Conductive Rubber Research
The field of conductive rubber research holds significant promise. With the rise of smart technologies and sustainable materials, exploring future directions can lead to groundbreaking innovations. Researchers are actively looking to create materials that not only perform well but also contribute positively to environmental sustainability. As industries seek more efficient and robust alternatives for traditional conductive materials, the importance of advancing conductive rubber cannot be overstated. This is where innovative ideas and methods intersect with practical applications, creating a landscape ripe for exploration.
Innovative Materials and Composites
Innovative materials are paving the way for new types of conductive rubber. One area of interest is the incorporation of nanomaterials, such as graphene and carbon nanotubes, into rubber matrices. These substances can dramatically enhance the electrical conductive properties of rubber while maintaining its elasticity. This blend can be crucial for applications where flexibility is paramount, like wearable technology or soft robotics.
- Graphene: Known for its excellent conductivity, combining graphene with rubber creates a composite material that could outperform traditional conductive rubbers.
- Carbon Nanotubes: They not only improve electrical conductivity but also lend mechanical strength to the rubber, making it suitable for demanding environments.
Another focus is on biocompatible materials, which can open new doors in the medical field. Developing conductive rubber that is safe for use within the human body could revolutionize medical device functionality.
Researchers are also looking into recyclable alternatives. For instance, the potential of bio-based rubbers, made from renewable resources, is gaining traction. This not only meets the growing demand for sustainability but also helps minimize the environmental impact associated with conventional rubber production.
"The integration of innovative materials in the rubber matrix can lead to a paradigm shift in how we perceive conductors, blending performance with sustainability."
"The integration of innovative materials in the rubber matrix can lead to a paradigm shift in how we perceive conductors, blending performance with sustainability."
Advancements in Manufacturing Techniques
Advancements in manufacturing techniques are equally essential for the future development of conductive rubber. Traditional methods often limit the uniformity and scalability of production. Innovations such as additive manufacturing, also known as 3D printing, are emerging as game changers in this arena. This technology allows for precise control over material properties and the ability to produce complex geometries that would be impossible to achieve with conventional manufacturing methods.
Additionally, improvements in mixing processes can enhance the uniformity of composite materials, ensuring better conductivity and mechanical properties. Techniques like high-shear mixing or the use of twin-screw extruders enable more consistent distribution of conductive fillers within the rubber.
- Additive Manufacturing: Customizable designs tailored to specific applications help optimize the use of materials and reduce waste.
- Improved Mixing Techniques: Enhanced dispersion of conductive materials can lead to improved electrical performance at lower concentrations, maximizing efficiency.
As these new manufacturing approaches mature, they hold the potential to revolutionize the entire industry. From bespoke components to mass production, the possibilities are vast and varied.
Finale
In wrapping up our exploration of rubber conductivity, it's crucial to note its significance extends well beyond just the materials themselves. Understanding how rubber conducts electricity is paramount for various industries, be it electrical engineering, consumer electronics, or medical devices. The ability of rubber to carry electric currents safely and efficiently presents numerous possibilities for innovation and improved technologies.
Summary of Key Points
Reflecting on the key elements addressed in this article:
- Fundamental properties of rubber, including its chemical composition and physical characteristics, are closely linked to its conductivity.
- Different types of rubber, such as natural and synthetic varieties, reveal varying conductive properties, impacting their applications.
- Current practices for measuring conductivity in rubber pave the way for enhanced materials, addressing the limitations highlighted in ongoing research.
- The hurdles faced in advancing conductive rubber stem from both material constraints and environmental challenges, emphasizing the need for sustainable approaches.
Through these points, it becomes evident that rubber conductivity not only facilitates existing technologies but also drives future advancements by sparking new innovations and enhancing current methodologies.
Implications for Future Research
Looking ahead, the future of conductive rubber research is teeming with opportunities. The focus on innovative materials and composites could revolutionize how these materials are utilized across various sectors. Further, refining manufacturing techniques will likely enhance the consistency and performance of conductive rubber products.
- Innovative Materials: Researchers are already exploring alternative fillers and blending different types of rubber to create hybrids that exhibit superior conductivity without compromising mechanical integrity.
- Sustainability Initiatives: With the growing emphasis on environmental responsibility, there is a push towards developing sustainable rubber alternatives that maintain or even improve conductive properties.
By navigating these pathways, the research community can contribute to creating safer, more efficient technologies that integrate conductive rubber into everyday applications. The discussion around rubber conductivity is not just a scientific inquiry; it's a crucial consideration for ensuring our material solutions align with future technological needs.
"Rubber conductivity is not merely a facet of material science; it offers a window into how we can shape a more advanced and sustainable future."
"Rubber conductivity is not merely a facet of material science; it offers a window into how we can shape a more advanced and sustainable future."