Transcribing mRNA from DNA: An In-Depth Guide
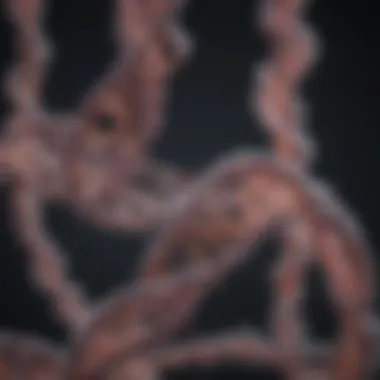
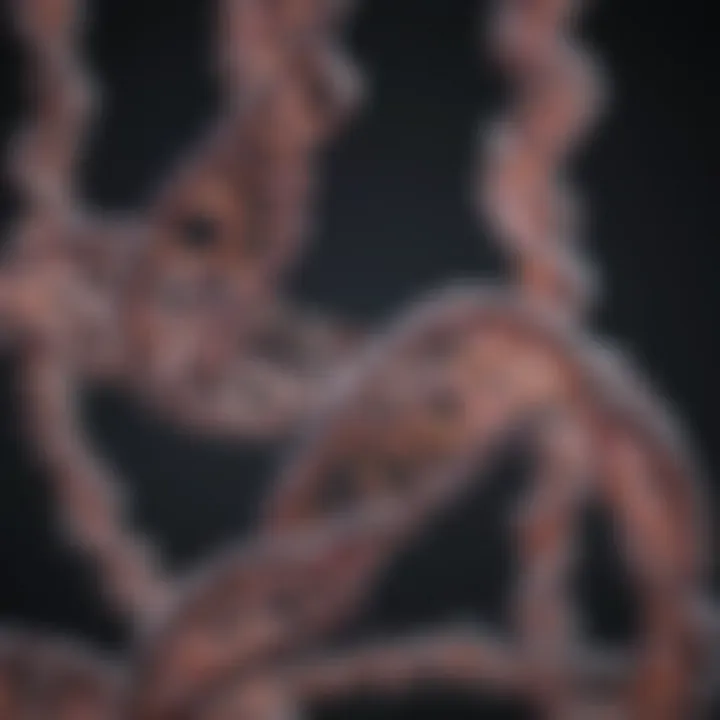
Intro
Transcription is a fundamental biological process that involves the synthesis of messenger RNA from a DNA template. Understanding this process is crucial for students, researchers, educators, and professionals, as it forms the basis of gene expression and regulation. In this guide, we will explore the various components and steps involved in transcription, as well as recent advancements in this field. The interplay of different molecules and regulatory mechanisms in transcription not only highlights the complexity of cellular processes but also provides insights into potential biotechnological applications.
Key Concepts
Definition of the Main Idea
Transcription is the first step in the process of gene expression, where a specific segment of DNA is transcribed into RNA. This RNA, specifically messenger RNA or mRNA, carries the genetic information needed for protein synthesis. The main player in this process is RNA polymerase, an enzyme that binds to the DNA and facilitates the synthesis of mRNA. The importance of transcription cannot be overstated, as it links the genetic information encoded in DNA to the functional proteins within a cell.
Overview of Scientific Principles
The transcription process involves several key steps: initiation, elongation, and termination. During initiation, RNA polymerase binds to a specific region of the DNA called the promoter. This is followed by elongation, where RNA polymerase moves along the DNA template, synthesizing mRNA. The process concludes with termination, where the synthesis of mRNA is finalized, and the RNA polymerase releases from the DNA strand.
Several other components play essential roles in transcription:
- Transcription Factors: These proteins help initiate and regulate the transcription process. They bind to specific DNA sequences and assist RNA polymerase in locating the promoter regions.
- Enhancers and Silencers: These are DNA sequences that can increase or decrease the transcription rate of specific genes. They interact with transcription factors to modulate gene expression.
- Post-Transcriptional Modifications: After synthesis, mRNA undergoes several modifications, including 5' capping, polyadenylation, and splicing. These changes are crucial for mRNA stability and translation into proteins.
"Transcription is a highly regulated process that ensures the right genes are expressed at the correct times and levels, which is essential for cellular function."
"Transcription is a highly regulated process that ensures the right genes are expressed at the correct times and levels, which is essential for cellular function."
Current Research Trends
Recent Studies and Findings
Recent advancements in molecular biology have significantly enhanced our understanding of transcription mechanisms. Studies have focused on the role of non-coding RNAs and their impact on transcriptional regulation. Non-coding RNAs can influence the activity of transcription factors and the accessibility of DNA, adding a layer of complexity to gene expression regulation.
Significant Breakthroughs in the Field
Biotechnological tools, such as CRISPR, have opened new avenues for manipulating transcription. Researchers are now able to edit gene sequences more precisely, leading to potential applications in gene therapy and synthetic biology. This has profound implications not only for basic research but also for drug development and treatment of genetic disorders.
As our understanding of transcription deepens, the implications extend beyond biology to fields like medicine and biotechnology. This guide will further explore these aspects, providing insights intended to enhance comprehension of transcription processes.
Intro to Transcription
Transcription serves as a fundamental mechanism in the biology of all living organisms. It involves the process where messenger RNA (mRNA) is synthesized from a DNA template. This stage is crucial as it translates genetic information contained within DNA into a format that can be read by ribosomes to produce proteins, the workhorses of the cell. Understanding transcription provides insight into gene expression, regulation, and the mechanisms underlying various biological processes.
Defining transcription correctly is essential, but comprehending its implications on gene expression is even more critical. Through this guide, readers will gain an in-depth knowledge of transcription's intricacies and its significance in cellular functions.
Definition of Transcription
Transcription can be defined as the biological process where a specific segment of DNA is copied into RNA by the enzyme RNA polymerase. This entails unwinding the DNA double helix and synthesizing a complementary RNA strand. In more technical terms, during transcription, RNA polymerase binds to the promoter region of a gene, signaling the start of transcription. It then moves along the DNA strand, adding ribonucleotides to form a single-stranded RNA molecule that reflects the DNA sequence.
There are three primary steps in transcription: initiation, elongation, and termination. Each phase is essential in ensuring that the resulting mRNA accurately represents the gene’s information. An understanding of these steps is foundational to grasping how genes are expressed and regulated in various organisms.
Importance of Transcription in Gene Expression
The importance of transcription in gene expression cannot be overstated. It acts as a regulatory layer between DNA and protein synthesis. Gene expression is not a straightforward flow from DNA to protein; rather, it involves intricate controls and checks at multiple stages, of which transcription is the first and foremost.
Key aspects include:
- Regulatory Roles: Transcription determines which genes are expressed at any given time, thus controlling phenotypic traits and responsive behaviors of cells. This is crucial in development, cellular differentiation, and response to environmental stimuli.
- Gene Regulation: The modulation of transcriptional activity can lead to different outcomes in cellular behavior. For instance, transcription factors bind to specific DNA sequences to enhance or repress gene expression, illustrating the complex feedback mechanisms in cellular regulation.
- Implications in Health and Disease: Abnormalities in transcription can lead to a wide range of diseases, including cancer. Understanding transcriptional control can aid in developing therapeutic strategies for these conditions.
"Transcription is not just a necessary step; it is a critical one that influences all aspects of cellular function and organismal development."
"Transcription is not just a necessary step; it is a critical one that influences all aspects of cellular function and organismal development."
In summary, transcription is the starting point of gene expression and thus serves as a linchpin in cellular and molecular biology research. Its importance in understanding biological systems, health, and disease highlights the need for continued study and discussion in this area.
The Molecular Structure of DNA
Understanding the molecular structure of DNA is critical to comprehending the intricacies involved in mRNA transcription. DNA, or deoxyribonucleic acid, serves as the blueprint for life, encoding the information necessary for the growth, development, and functioning of living organisms. The structure of DNA not only underpins the stability and transmission of genetic information but also influences how this information is accessed and utilized during transcription. A clear grasp of its components and configuration is indispensable for professionals exploring gene expression and regulation.
Components of DNA
DNA is composed of four fundamental components known as nucleotides. Each nucleotide contains three distinct parts:
- A phosphate group: This connects adjacent nucleotides, forming the backbone of the DNA strand.
- A deoxyribose sugar: The sugar moiety that lends its name to DNA, it serves as the framework that supports the nucleotide bases.
- Nitrogenous bases: There are four types, specifically adenine (A), thymine (T), cytosine (C), and guanine (G). The sequence of these bases encodes genetic information.
The specificity of base pairing is notable. Adenine pairs with thymine, while cytosine pairs with guanine. This complementary base pairing is a key feature that ensures fidelity during DNA replication and the subsequent transcription processes.
Double Helix Configuration
The structural arrangement of DNA is often referred to as a double helix. This configuration is not merely aesthetic; it plays a significant role in the stability and functionality of the genetic material. The two strands of DNA run in opposite directions and twist around each other, forming a right-handed helix. This structure provides several benefits:
- Stability: The base pairs stack within the helix, shielded from the surrounding environment, which reduces the likelihood of damage.
- Accessibility: The helical structure allows the strands to separate easily. During transcription, RNA polymerase requires access to the unwrapped DNA sequence to synthesize the mRNA strand.
- Replication Fidelity: The complementary nature of the base pairs assists in ensuring accurate replication and allows for repair mechanisms to correct errors if they occur.
"The double helix not only showcases the elegance of DNA but also embodies the complexity and precision required for biological functions."
"The double helix not only showcases the elegance of DNA but also embodies the complexity and precision required for biological functions."
The double helix configuration ultimately permits efficient access and transmission of genetic information, critical during the process of mRNA transcription. Understanding these components and their arrangement provides the foundation for deeper exploration into transcription mechanisms and their comprehensive regulation.
Overview of mRNA
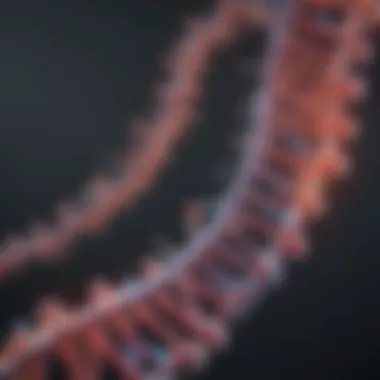
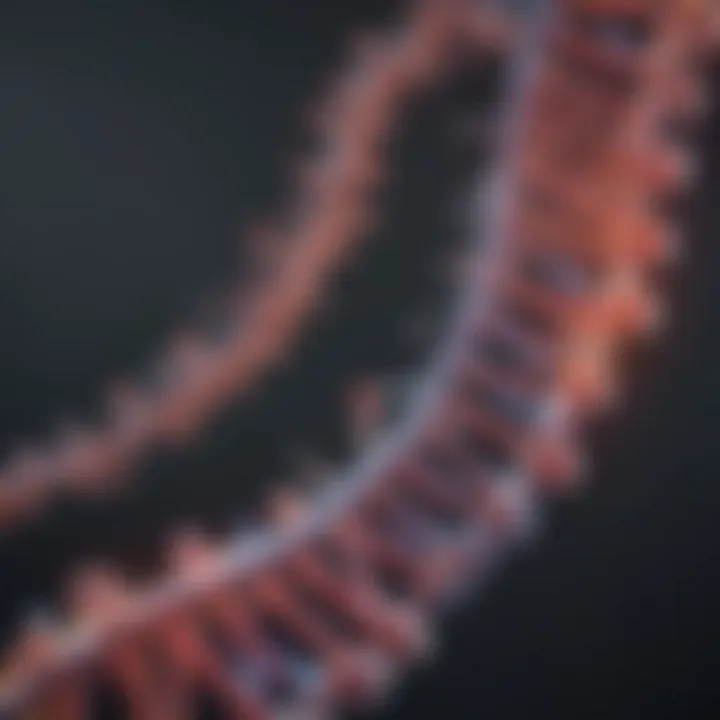
The messenger RNA, or mRNA, plays a pivotal role in the process of gene expression. It acts as a bridge between the genetic information stored in DNA and the protein synthesis machinery of the cell. Understanding mRNA is crucial because it directly reflects the gene activity, allowing us to comprehend how genetic information is utilized and regulated in various biological contexts.
Structure and Composition of mRNA
mRNA is a linear molecule composed of nucleotides, which are the building blocks of RNA. Each nucleotide includes a ribose sugar, a phosphate group, and a nitrogenous base. The sequence of these bases—adenine, uracil, guanine, and cytosine—carries the genetic instructions required to synthesize proteins.
The structure of mRNA is characterized by a few important features:
- 5' Cap: This modified guanine nucleotide at the 5' end of the mRNA protects it from degradation and plays a role in ribosome binding during translation.
- Coding Sequence: The main part of the mRNA molecule consists of codons, which are triplet sequences that correspond to specific amino acids.
- Poly(A) Tail: Located at the 3' end, this sequence of adenine nucleotides enhances the stability of mRNA and assists in export from the nucleus.
These elements work together to ensure that mRNA can be efficiently translated into proteins.
Function of mRNA
The primary function of mRNA is to carry the genetic code from DNA to the ribosomes, where proteins are synthesized. In eukaryotic cells, this process involves several key steps:
- Transcription: mRNA is synthesized from a DNA template by RNA polymerase. This creates a complementary RNA strand that mirrors the coding region of the DNA.
- Processing: Before mRNA exits the nucleus, it undergoes several modifications. This includes adding the 5' cap, polyadenylation, and splicing out introns.
- Translation: The mature mRNA is transported to the cytoplasm, where ribosomes read its sequence and translate it into a specific polypeptide chain, forming a functional protein.
In summary, mRNA is integral to gene expression, serving as the template for protein synthesis. Its structure, along with its processes of transcription and translation, highlights its critical role in the central dogma of molecular biology.
Important Note: mRNA's stability and efficiency are influenced by modifications during processing, which can significantly affect gene expression and cellular function.
Important Note: mRNA's stability and efficiency are influenced by modifications during processing, which can significantly affect gene expression and cellular function.
The Role of RNA Polymerase
RNA polymerase is an essential enzyme that facilitates the transcription of genomic DNA into messenger RNA (mRNA). It plays a central role in gene expression and is critical for the proper manifestation of biological functions. The ability of RNA polymerase to synthesize mRNA determines the fate of a gene, influencing cellular behavior and function. Understanding RNA polymerase thus provides insights into the intricate processes governing life at the molecular level.
Mechanism of Action
The mechanism of action of RNA polymerase involves multiple stages that culminate in the synthesis of RNA. The process starts with the binding of RNA polymerase to the promoter region of the DNA. This specific region is characterized by sequences that signal the start of transcription. Once attached, RNA polymerase separates the two strands of the DNA helix, unwinding it to access the coding strand.
After strand separation, the enzyme synthesizes a single strand of RNA by adding ribonucleotides complementary to the DNA template. This reaction is facilitated by the enzyme's active site, which catalyzes the formation of phosphodiester bonds, linking nucleotides together. As RNA polymerase advances along the DNA, elongation occurs, and the newly formed mRNA strand detaches, allowing RNA polymerase to continue until it reaches a termination sequence. This termination signals the end of the transcription process.
Types of RNA Polymerases
There are several types of RNA polymerases, each serving distinct functions in the cell. The three primary types are RNA polymerase I, II, and III.
- RNA Polymerase I: Predominantly responsible for synthesizing ribosomal RNA (rRNA), this polymerase operates within the nucleolus. The rRNA produced is essential for ribosome formation, a crucial component for protein synthesis.
- RNA Polymerase II: This is the most significant polymerase in terms of mRNA synthesis. It transcribes protein-coding genes and is critical in producing pre-mRNA, which undergoes several post-transcriptional modifications before becoming mature mRNA.
- RNA Polymerase III: It synthesizes smaller RNA molecules, including transfer RNA (tRNA) and some small nuclear RNA (snRNA). These RNA types play various roles in translation and splicing.
The characterization of these RNA polymerases illustrates the complexity and specialization of transcription machinery in eukaryotic cells. Understanding their distinct roles helps researchers appreciate how transcription regulation can affect gene expression and, ultimately, cellular function.
"The nuanced interplay of various types of RNA polymerases reflects the sophisticated mechanisms that govern gene expression and cell behavior."
"The nuanced interplay of various types of RNA polymerases reflects the sophisticated mechanisms that govern gene expression and cell behavior."
In summary, RNA polymerase acts as a fundamental enzyme, orchestrating the conversion of DNA into functional RNA, driving essential biological processes.
Transcription Factors and Their Role
Transcription factors are key players in the process of transcription and have a significant impact on gene regulation. They assist in defining when, where, and how much of a specific gene is expressed. Understanding their role is crucial to grasp the nuances of gene expression and regulation. This section will delve into the definition and functions of transcription factors, as well as the various types that exist within cellular environments.
Definition and Function of Transcription Factors
Transcription factors are proteins that bind to specific DNA sequences, usually located in the promoter region of a gene. Their primary function is to regulate the transcription of genetic information from DNA to messenger RNA. They can act as either activators or repressors of transcription.
- Activators enhance the recruitment of RNA polymerase to the DNA, promoting gene expression. They can interact with coactivators and the general transcription machinery, making DNA more accessible for transcription.
- Repressors, on the other hand, inhibit transcription by blocking the action of RNA polymerase or by recruiting corepressors that modify the chromatin structure to a less accessible state.
This dynamic balance of activators and repressors allows cells to respond rapidly to internal and external signals, ensuring that the appropriate genes are expressed at the right times, thereby maintaining cellular homeostasis.
Types of Transcription Factors
Transcription factors can be broadly classified based on their functionality and structure. Some key categories are:
- General Transcription Factors: These are essential for the basic transcription process of all genes. They form the core transcription machinery required for RNA polymerase to initiate transcription. Examples include TFIID, which recognizes the promoter region, and TFIIB, which helps RNA polymerase bind to the DNA.
- Specific Transcription Factors: These factors bind to unique DNA sequences and are responsible for controlling the expression of particular genes. Their activity is often influenced by cellular signals, which can lead to changes in their concentration or activity.
- Homeotic Proteins: These proteins play a significant role in controlling the body plan of an embryo by regulating the expression of genes that determine the identity of body segments.
- Chromatin-Remodeling Factors: These are essential for modifying the accessibility of DNA. They can reposition or restructure nucleosomes to enable or suppress transcription of specific genes.
Understanding the various types of transcription factors and their specific roles adds depth to our comprehension of genetics, providing insights into both normal and pathological gene regulation.
Understanding the various types of transcription factors and their specific roles adds depth to our comprehension of genetics, providing insights into both normal and pathological gene regulation.
In summary, transcription factors are integral to the orchestration of gene expression. Their various classes and functions emphasize the complexity and precision of genetic regulation within cells. Recognizing their importance is foundational in the field of molecular biology and genetics, paving the way for advancements in research and therapeutic interventions.
The Process of Transcription
Transcription is a crucial stage in the gene expression pathway, whereby messenger RNA (mRNA) is synthesized from a DNA template. Understanding the process of transcription is essential as it solidifies our comprehension of how genetic information is conveyed to produce proteins, which are vital for cellular functions. Without transcription, the instructions encoded within DNA would remain dormant. Thus, this section will elaborate on the distinct phases of transcription: initiation, elongation, and termination, while emphasizing their significance in cellular biology.
Initiation of Transcription
The initiation phase marks the beginning of transcription, where RNA polymerase binds to a specific region on the DNA known as the promoter. This region is upstream of the gene being transcribed and contains crucial sequences that signal where the transcription process should start. Before RNA polymerase can bind, transcription factors must first assemble at the promoter, forming a complex that allows RNA polymerase to recognize and attach to the DNA.
Once the RNA polymerase is bound, the double-stranded DNA unwinds and separates, exposing the template strand. This unwinding is vital; it opens the door for the mRNA strand to be synthesized. The process requires energy, primarily derived from ATP, which is crucial for the formation of the necessary bonds during mRNA synthesis.
Elongation of the mRNA Strand
During the elongation phase, RNA polymerase moves along the DNA template strand, synthesizing mRNA in the 5' to 3' direction. The enzyme catalyzes the addition of ribonucleotides, which are complementary to the DNA template. This synthesis incorporates uracil in the place of thymine found in DNA, pairing with adenine.
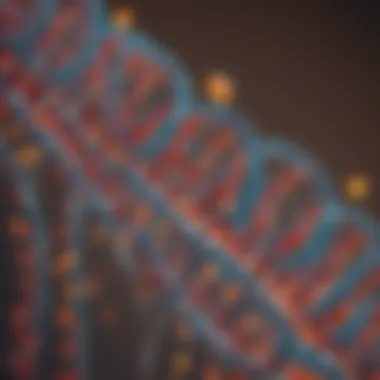
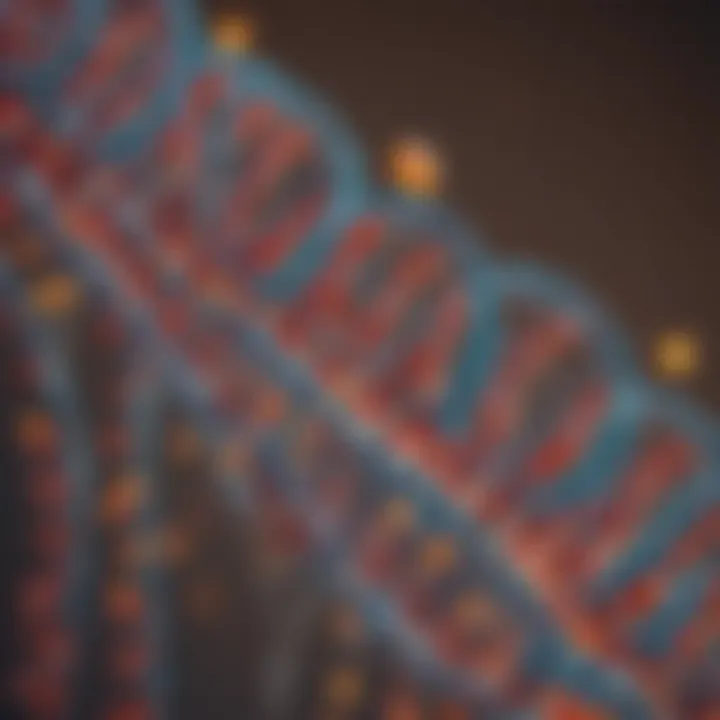
As RNA polymerase advances, it continues to unwind the DNA, forming a transcription bubble where the mRNA strand is synthesized. The emerging mRNA molecule trails behind the polymerase, and with each added nucleotide, the length of the mRNA increases. This phase is marked by rapid transcription of RNA, allowing for efficient gene expression and protein production.
Termination of Transcription
The termination phase is where the synthesis of RNA concludes. This process can occur once RNA polymerase reaches a specific sequence in the DNA known as the termination signal. In bacteria, termination can happen through two mechanisms: Rho-dependent and Rho-independent termination. Eukaryotic cells, however, have a more complex termination process often involving a polyadenylation signal.
Upon recognition of the termination sequence, RNA polymerase will release the newly synthesized mRNA strand and detach from the DNA. This completion of transcription is crucial as it allows the mRNA to move into the next phase of gene expression, where it undergoes various modifications before translation.
"Transcription is the essential bridge between the genetic information contained in DNA and the functional proteins essential for an organism's life."
"Transcription is the essential bridge between the genetic information contained in DNA and the functional proteins essential for an organism's life."
In summary, understanding the process of transcription grants insight into the fundamental mechanisms of gene expression. Each phase - initiation, elongation, and termination - plays an integral role in accurately transcribing genetic information into a usable format, thus underpinning cellular function and regulation.
Post-Transcriptional Modifications
Post-transcriptional modifications are critical aspects of gene expression that occur after the primary mRNA transcript is synthesized. These modifications enhance the stability, transport, and translation of mRNA. They ensure that gene expression can be regulated precisely and efficiently within the eukaryotic cell.
Capping and Polyadenylation
The first significant modification is the addition of a 5' cap to the mRNA molecule. This cap is a modified guanine nucleotide that is crucial for mRNA stability. It protects the mRNA from degradation by exonucleases and assists in the initiation of translation. The cap structure facilitates the export of mRNA from the nucleus to the cytoplasm, where it can be translated into protein.
Another important modification is polyadenylation. A series of adenine residues, known as a poly-A tail, is added to the 3' end of the mRNA. This tail increases the stability of the mRNA and also plays a role in the regulation of translation. The length of the poly-A tail can affect the half-life of the mRNA in the cytoplasm. A longer tail generally corresponds to increased stability and more efficient translation.
Splicing of Introns and Exons
Splicing is a fundamental process where non-coding regions of mRNA, called introns, are removed, and the coding regions, or exons, are joined together. This process is essential for producing a mature mRNA molecule that can be translated into a functional protein. The splicing mechanism is carried out by a complex called the spliceosome, which recognizes specific sequences at the intron-exon boundaries.
This modification allows for alternative splicing, which enables a single gene to produce multiple protein isoforms by varying the combination of exons included in the final mRNA transcript. Such diversity is important for the functional versatility of proteins and plays a crucial role in cellular development and differentiation.
"Post-transcriptional modifications are not merely alterations; they are essential steps that transform the raw genetic transcript into a stable and translatable molecule."
"Post-transcriptional modifications are not merely alterations; they are essential steps that transform the raw genetic transcript into a stable and translatable molecule."
In summary, post-transcriptional modifications such as capping, polyadenylation, and splicing greatly impact mRNA integrity and functionality. They contribute to the regulation of gene expression, allowing the cell to respond quickly to internal and external signals. Understanding these processes is vital for researchers aiming to explore genetic expression and its implications in biology and medicine.
Regulation of Transcription
The regulation of transcription is a pivotal process that dictates how genes are expressed in an organism. It ensures that the right genes are expressed at the right times and in appropriate amounts. This regulation has profound implications for cell function and organismal development. Understanding this intricate process is essential not just for researchers but also for students and professionals involved in genetic studies, biotechnology, and medicine.
Key components in the regulation of transcription include transcription factors, enhancers, and silencers. Transcription factors are proteins that bind to specific DNA sequences, influencing the activity of RNA polymerase. Enhancers, which may be located far from the gene they regulate, assist in increasing transcription levels, while silencers work to decrease gene expression. These elements allow for a highly nuanced control system, responding to various internal and external signals.
The benefits of effective transcription regulation are numerous. This mechanism enables cells to adapt to changing environments, maintain homeostasis, and differentiate into various cell types. Considerations regarding the regulation of transcription also encompass its role in disease. Abnormal regulation can lead to conditions such as cancer, where genes that control cell growth may be overexpressed or silenced inappropriately.
"The tight control of gene expression through transcription regulation is crucial for cellular diversity and function."
"The tight control of gene expression through transcription regulation is crucial for cellular diversity and function."
In summary, the regulation of transcription not only governs gene expression but also represents a fundamental area of study in understanding biology. It bridges the gap between genetic information and its practical manifestations in living organisms.
Mechanisms of Gene Regulation
Gene regulation is a complex array of processes that manage the availability of mRNA for translation into proteins. Mechanisms include chromatin remodeling, RNA interference, and post-transcriptional control.
- Chromatin Remodeling:
- RNA Interference (RNAi):
- Post-Transcriptional Control:
- This involves the alteration of chromatin structure to make DNA more or less accessible to transcription machinery. Nuclear proteins, such as histones, play a central role in this remodeling.
- A method through which small RNA molecules inhibit the expression of specific genes. This can result in the degradation of mRNA or blocking of its translation.
- Various mechanisms occur after mRNA synthesis. These include splicing variations, where different mRNA isoforms are generated from the same gene, allowing for increased diversity of proteins.
Each of these mechanisms contributes to the overall regulation of gene expression, underscoring the complexity and sophistication of cellular control systems.
Role of Enhancers and Silencers
Enhancers and silencers are crucial elements in the transcription regulation landscape. Both play opposite roles, but they are vital in ensuring that gene expression is tightly controlled. Enhancers can be thought of as activators. They bind transcription factors that interact with RNA polymerase to initiate transcription, sometimes even when they are located far from the actual gene they regulate. This allows the cell to coordinate diverse stimuli and express genes accordingly.
Conversely, silencers are sequences that repress gene expression. They recruit proteins that inhibit transcription factor activity or obstruct the progress of RNA polymerase along the gene. The balance between enhancer and silencer activity is essential for precise gene control, influencing various biological processes.
In summary, enhancers and silencers interact through complex pathways to modulate transcription. Investigating their function provides valuable insight into how genes are regulated and the implications for health and disease.
Technological Advances in Transcription Research
The field of transcription research has significantly evolved due to technological advancements. These developments enhance both our understanding and application of transcriptional mechanisms. Understanding these advances is essential to grasp how they influence research outcomes and trajectories. The integration of new technologies enables scientists to explore the intricacies of mRNA synthesis and its regulatory pathways with greater accuracy.
CRISPR and Gene Editing
CRISPR technology revolutionizes the field of genetics and molecular biology. This powerful tool allows for precise editing of DNA sequences, making it a valuable asset in transcription research. With the ability to target specific genes, researchers can manipulate transcription processes and understand gene expression more thoroughly.
- Precision: CRISPR enables specific modifications at desired sites of the genome. This leads to a clearer understanding of how certain changes affect transcription.
- Efficiency: Traditional methods of gene editing are often time-consuming and less accurate. CRISPR provides a more efficient method for making desired alterations.
- Applications: From studying diseases to developing gene therapies, CRISPR has a broad array of applications. Researchers are increasingly using it to identify transcription factor binding sites and studying their functional importance.
Through CRISPR and gene editing, scientists have a new lens through which to view transcription regulation. This information can lead to innovative solutions for diseases linked to transcription errors.
Synthetic Biology Applications
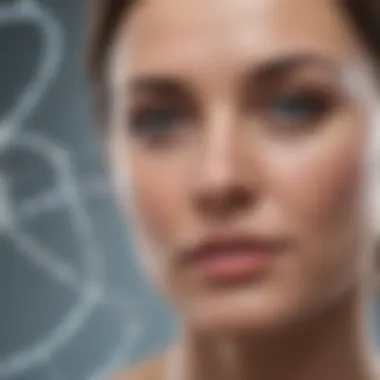
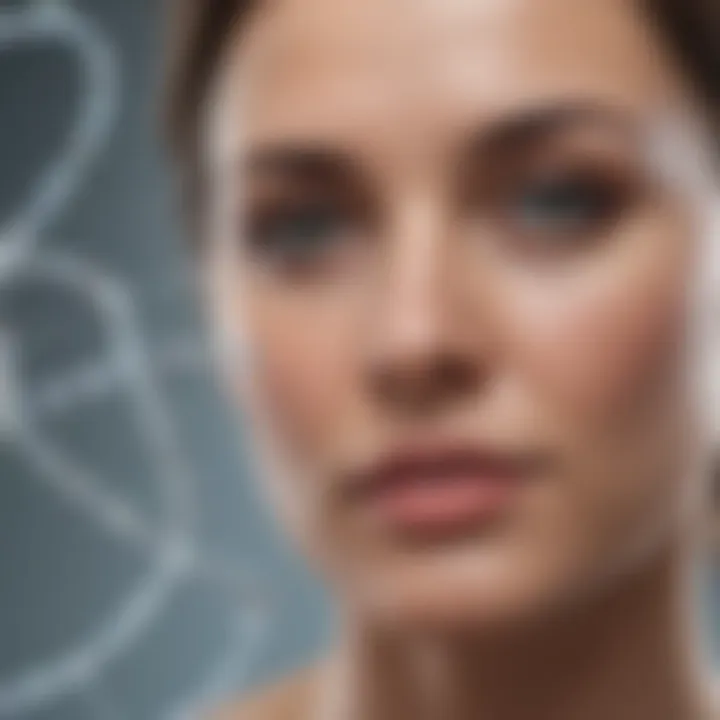
Synthetic biology combines biology and engineering to create new biological parts and systems. This area has expanded the scope of transcription research, allowing for novel applications.
- Gene Constructs: Researchers can design synthetic genes to explore transcriptional dynamics. This helps us understand how different sequences influence gene expression.
- Pathway Engineering: By creating synthetic pathways, scientists can study interactions between various transcription factors and their target genes. This allows insights into how regulatory networks function.
- Therapeutics Development: Synthetic biology facilitates the design of new treatments. By manipulating transcription processes, researchers can find ways to turn off genes associated with diseases.
In summary, the advances in technology like CRISPR and synthetic biology have profound implications for transcription research. These tools not only enhance understanding but also open pathways for therapeutic interventions.
Challenges in Studying Transcription
Understanding the complexities of transcription is essential for advancing genetic research and applications. However, various challenges hinder researchers’ efforts in this domain. Addressing these obstacles enhances our overall grasp of transcription and its role in gene expression. Here are some notable challenges that confront scientists in this field.
Technical Limitations
Research in transcription is often restricted by technical constraints. For instance, traditional methods used to study RNA synthesis can be inefficient and can lead to incomplete or inaccurate data. One primary limitation lies in the resolution of techniques like RNA sequencing. Even high-throughput sequencing faces difficulties in capturing transient mRNA transcripts, which may have brief lifespans. Consequently, quantifying these may generate misleading conclusions about gene expression levels.
Moreover, current technologies might not effectively distinguish between different isoforms of mRNA produced from the same gene. Alternative splicing creates diverse mRNA molecules, and missing these subtleties can lead to oversimplified interpretations of transcriptional control. Additional factors such as cellular noise and variability in transcript lifespan further complicate the analysis.
Interpreting Transcriptional Data
Interpretation of transcriptional data presents another significant hurdle. The sheer volume and complexity of data generated in experiments can be overwhelming. Researchers must discern various signals against a backdrop of background noise. This requires sophisticated bioinformatics tools and analysis methods.
Effective data interpretation also depends on understanding biological contexts. Different cell types and environmental conditions can influence gene expression. Therefore, applying a one-size-fits-all approach to analysis is impractical. Researchers must integrate interdisciplinary knowledge from genomics, proteomics, and molecular biology to attain robust conclusions.
The challenge is not solely technical; it is also conceptual. Scientists need to develop and refine models that predict transcriptional behavior across different scenarios. This requires a continuous cycle of testing hypotheses and revising understandings based on emerging data.
In summary, the challenges in studying transcription extend beyond mere technical issues. They involve a complex interplay between data collection, analysis, and the biological systems at play.
In summary, the challenges in studying transcription extend beyond mere technical issues. They involve a complex interplay between data collection, analysis, and the biological systems at play.
By navigating these challenges, researchers can improve transcription studies and ultimately contribute to our understanding of gene expression and regulation.
Future Directions in Transcription Research
Research in transcription is continuously evolving. Understanding how mRNA is synthesized from DNA is crucial for many biological fields. The future of this research focuses on innovative methods to manipulate gene expression. Advances in technology will likely lead to further insights.
Emerging Trends in Gene Regulation
New methods in gene regulation emerging can change how we approach genetics. One major trend is the use of CRISPR technology. This technique enhances the precision of genetic modifications. By targeting specific genes, researchers can observe the effects of altered transcription processes. Other methods include gene silencing and activation strategies. These techniques provide tools to study not only gene function but also phenotypic outcomes of transcriptional changes.
Researchers are also exploring non-coding RNAs in gene regulation. These RNAs, once thought to serve no purpose, are proving to play significant regulatory roles. For instance, long non-coding RNAs (lncRNAs) can modulate transcription by interacting with DNA, RNA, or proteins. Understanding these roles may open avenues for therapies targeting gene expression.
"Emerging tools and techniques are transforming the landscape of transcription research, revealing deeper insights into gene regulation."
"Emerging tools and techniques are transforming the landscape of transcription research, revealing deeper insights into gene regulation."
Potential for Therapeutic Applications
The therapeutic potential of transcription research is compelling. Understanding transcription opens pathways for innovative treatments. One area of interest is cancer therapy. Cancers often arise from misregulated transcription. By correcting or inhibiting these processes, we might develop targeted therapies.
Gene therapies are also on the rise, aiming to treat genetic disorders at their source. Techniques that enhance or inhibit transcription can possibly remedy ineffective gene expression. Additionally, small molecule drugs targeting transcription factors may provide treatments for various diseases.
As we advance our understanding of transcription, these therapeutic possibilities illustrate the importance of continued research in this field. The implications for healthcare are profound, potentially enabling more effective and personalized medical interventions.
Epilogues
The conclusion of any scientific discussion plays an essential role in reinforcing the significance of the topic at hand. In the realm of mRNA transcription from DNA, the conclusions drawn provide a synthesis of the knowledge acquired throughout the research process. Key findings in this domain are integral for enhancing understanding and guiding future studies. Moreover, it allows readers to grasp the implications of transcription errors or irregularities in gene expression that can result in various diseases.
Summary of Key Points
In summary, several critical points emerge from the analysis of mRNA transcription:
- Transcription Process: This article highlighted the complex stages of transcription, including initiation, elongation, and termination, all vital for synthesizing mRNA accurately.
- Functions of RNA Polymerase: The intricate role of RNA polymerase was discussed, emphasizing its necessity for catalyzing RNA synthesis.
- Significance of Transcription Factors: These proteins are crucial for regulating the transcription process, either enhancing or silencing gene expression based on various signals.
- Post-Transcriptional Modifications: Understanding capping, polyadenylation, and splicing has underscored the importance of mRNA processing before translation.
- Future Directions: The exploration of new technologies and therapeutic applications represents a promising frontier in transcription research.
Final Thoughts on mRNA Transcription Research
Reflecting on the research of mRNA transcription, it becomes evident that our understanding is still evolving. New technologies, such as CRISPR and synthetic biology methods, contribute impactful insights into gene regulation. Continuous exploration is necessary for various practical applications, including genetic therapies and biotechnological innovations.
Moreover, the ability to manipulate transcription processes can lead to advancements in treating genetic disorders and other diseases. As our tools and techniques improve, the potential benefits for health and biology are profound.
"Understanding transcription is paramount. It is the first step in the flow of genetic information that ultimately dictates the function of cells."
"Understanding transcription is paramount. It is the first step in the flow of genetic information that ultimately dictates the function of cells."
Continued investment in research and education will enhance comprehension in this field, bridging gaps that exist in current science.
References and Further Reading
Understanding the transcription process is essential for students, researchers, educators, and professionals in the field of molecular biology. The section on references and further reading serves as a critical resource for those looking to deepen their knowledge. It provides access to scientific literature that illustrates key findings and methodologies related to mRNA synthesis and gene expression.
By engaging with recommended materials, readers can gain insights into both foundational concepts and emerging research in transcription. This section is not just a list of references; it also includes resources that explain methods, tools, and applications relevant to gene transcription and modifications. The significance of this section lies in its ability to bridge the gap between theoretical knowledge and practical application in this rapidly evolving field.
Researchers often face challenges when trying to stay updated with advancements in transcription research. References can guide them through complex topics, making it easier to locate valuable information. Here, we outline several recommended scientific literature sources, and online platforms that facilitate ongoing learning.
Recommended Scientific Literature
- "Molecular Biology of the Cell" by Alberts et al.
This book is a comprehensive resource that covers fundamental concepts in cell biology, including transcription mechanics. Each chapter provides detailed information that can clarify the role of mRNA in cellular function. - "Transcriptional Regulation: A Practical Approach" edited by H. K. Daubner and C. M. Dehosse
This work focuses on transcriptional regulation, providing insights into methodologies used in gene regulation research. It is an excellent reference for researchers conducting experiments. - "Principles of Gene Manipulation and Genomics" by Primrose and Twyman
This text discusses genetic manipulation techniques, particularly relevant for those involved in synthetic biology and gene editing.
Online Resources for Continued Learning
- Encyclopedia Britannica
A well-respected source offering articles on recent discoveries in genetics and molecular biology. Great for both basic understanding and in-depth analysis. Visit Encyclopedia Britannica - Wikipedia
This platform provides a broad overview of transcription-related topics. While caution is advised regarding editing, general information can be quite helpful. Explore on Wikipedia - reddit.com/r/biology
For real-time discussion and shared insights among biology enthusiasts and professionals, this subreddit serves as a community for exchanging information and experiences in transcription studies. Join the Conversation - ResearchGate
A network where academics publish their research findings and link with peers. It can be essential for finding publications that relate closely to specific transcription topics. Connect on ResearchGate
The references and online resources provided here will equip readers with necessary tools to further explore the realm of mRNA transcription, ensuring a solid foundation for both academic pursuits and practical applications in research.