Ultrasonic Oxygen Sensors: Mechanisms and Future Prospects
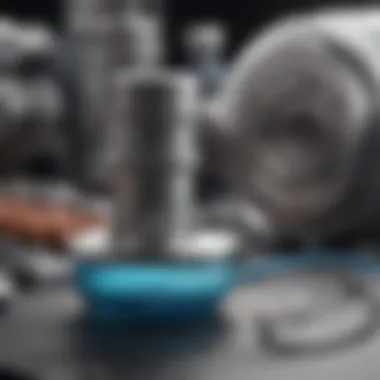
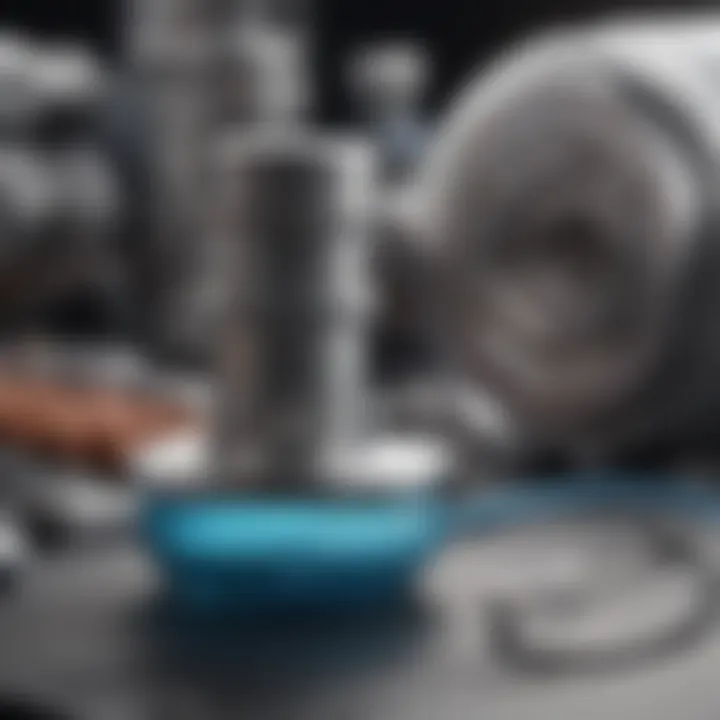
Intro
Ultrasonic oxygen sensors stand at the frontier of technological innovation, heralding a new age in gas detection. Utilizing sound waves to determine oxygen concentration, these devices go beyond traditional sensors, offering enhanced accuracy and efficiency. Understanding their operational principles leads us to appreciate why they are being increasingly adopted across various sectors including healthcare, environmental monitoring, and industrial safety.
A deeper dive into the mechanisms, advantages, and challenges of ultrasonic oxygen sensors reveals a landscape ripe with potential yet fraught with complexities. This article aims to illuminate these aspects, serving as a compass for students, researchers, educators, and professionals eager to grasp the future trajectory of oxygen sensing technology.
Prelude to Ultrasonic Oxygen Sensors
Ultrasonic oxygen sensors are a critical piece in the puzzle of modern technology. They bring precision and innovation to various fields that rely on accurate gas detection. Understanding how these sensors work can open doors to new applications and improvements across industries. The mechanisms behind these sensors are nothing short of remarkable, providing a means to measure oxygen levels with non-invasive methods that further enhance their utility.
What is an Ultrasonic Oxygen Sensor?
An ultrasonic oxygen sensor is a device that utilizes ultrasonic waves to detect the concentration of oxygen in the environment. Unlike traditional methods that may involve chemical reactions or physical interactions with gases, ultrasonic sensors emit sound waves that bounce off oxygen molecules. By analyzing these reflected waves, the sensor can determine the concentration of oxygen present.
These sensors are designed to operate in a range of environments, ensuring adaptability. With ongoing advancements in technology, they are increasingly favored for their ability to provide quick readings without altering the gas composition in their surroundings—an essential advantage in sensitive applications such as medical environments and industrial processes.
Historical Context and Development
The journey of ultrasonic oxygen sensors dates back to when researchers first explored the possibilities of using sound waves for detection purposes. The concept of employing ultrasonic waves gained traction in the mid-20th century, as scientists recognized the potential for non-invasive measurement techniques in various scientific endeavors.
In the early days, much of the focus was on developing the basic technology. Early models exhibited limitations in sensitivity and reliability. However, continuous research and development led to significant enhancements. Today, modern sensors are often integrated with sophisticated algorithms and microcontrollers, enabling them to process data in real-time and adapt to fluctuating environmental conditions.
"Advancements in sensor technology often reflect broader trends in research and development, illustrating a commitment to precision and efficiency in monitoring essential gases like oxygen."
"Advancements in sensor technology often reflect broader trends in research and development, illustrating a commitment to precision and efficiency in monitoring essential gases like oxygen."
In recent years, the rise of the Internet of Things (IoT) and smart technologies has spurred further innovations in ultrasonic oxygen sensing. Sensors can now interface with other devices, allowing for enhanced data analytics and remote monitoring capabilities. This transforms how industries approach gas detection, ensuring safety and efficiency.
Overall, understanding the historical context and ongoing evolution of ultrasonic oxygen sensors provides valuable insight into their significance in modern applications. Knowing where they come from highlights the importance of continuous innovation in addressing the challenges associated with oxygen measurement.
The Science Behind Ultrasonic Detection
Understanding the mechanisms underlying ultrasonic detection is key to grasping how ultrasonic oxygen sensors operate. In this section, we will delve into fundamental principles such as the properties of ultrasonic waves, their interaction with oxygen molecules, and the various signal processing techniques utilized in these sensors. Each of these components plays a vital role in enhancing the reliability and precision of oxygen measurement, which is crucial across numerous applications, from medical devices to environmental monitoring.
Principle of Ultrasonic Waves
Ultrasonic waves are sound waves that fall above the threshold of human hearing, typically beyond 20 kHz. Their ability to penetrate different mediums makes them vital for effective sensing in various applications.
When investigating ultrasonic oxygen sensors, it is important to note how these waves can provide non-invasive and real-time measurements. The mechanics behind ultrasonic waves involve fluctuations in pressure that create vibrations in the medium through which they propagate. Key aspects to consider include:
- Frequency: The frequency of these waves influences their behavior in different materials. Higher frequencies can lead to better resolution but may also impact penetration depth.
- Impedance: This refers to how much opposition the waves face while traveling through different substances. Variations in impedance between air and oxygen aid in determining the concentration of oxygen present.
- Wave Propagation: The travel speed of ultrasonic waves is contingent upon the characteristics of the transmitting and receiving mediums. Changes in the composition can alter this speed, which sensor technology can leverage for precise measurements.
Interaction with Oxygen Molecules
The interaction of ultrasonic waves with oxygen molecules is a fascinating phenomenon that showcases the underlying physics at play. Oxygen molecules have specific acoustic properties, allowing them to scatter and absorb ultrasonic waves differently than other gases.
When waves encounter an oxygen molecule, they may undergo phenomena such as:
- Refraction: Here, the waves bend as they travel through different media, altering their direction based on the density and temperature of the gas.
- Scattering: Some waves get scattered at various angles based on the molecular structure of oxygen. This scattering effect is a pivotal aspect in determining concentration levels.
- Absorption: Oxygen molecules can absorb specific frequencies of ultrasonic waves, helping to provide accurate metrics on gas concentrations.
Understanding these interactions helps in designing sensors that are not just efficient but also highly accurate in measuring oxygen levels. Essentially, this allows for the employment of advanced algorithms to interpret the data obtained from these interactions, leading to better and more reliable sensor outputs.
Signal Processing Techniques
The collected data from ultrasonic sensors undergoes various processing techniques crucial for interpreting and utilizing the information effectively, as raw data can often be too complex or noisy for practical applications.
Some important signal processing methods include:
- Noise Reduction: Filtering techniques help eliminate background noise, enhancing the quality of the measurements.
- Fourier Transform: This mathematical technique helps in dissecting the signal into its component frequencies, enabling a clearer understanding of the interactions occurring within the sensor environment.
- Machine Learning Algorithms: With the advancements in technology, machine learning approaches are increasingly being integrated to predict and calibrate the sensors’ responses under various conditions. This not only improves the accuracy but also enhances user interface and functionality.
"The essence of sensor technology lies in not just detection, but interpretation; understanding the science behind every wave is what leads to innovation."
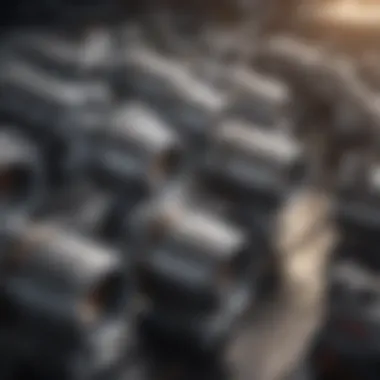
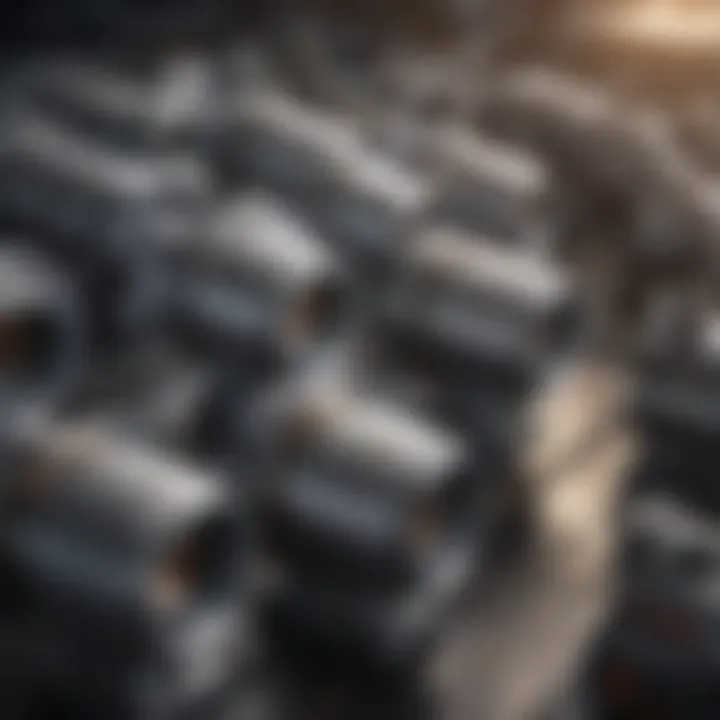
"The essence of sensor technology lies in not just detection, but interpretation; understanding the science behind every wave is what leads to innovation."
By distilling these complex processes into practical applications, researchers and professionals can pave the way for more effective utilization of ultrasonic technology in oxygen sensing.
Key Components of Ultrasonic Oxygen Sensors
Understanding the key components of ultrasonic oxygen sensors reveals not only how they function but also underscores their unique advantages and potential limitations. Each element is crucial, and their interplay defines the sensor's performance and reliability. In this section, we delve into transducers, microcontroller integration, and the necessity for an efficient power supply, highlighting their roles and significance.
Transducers and Their Functions
At the heart of an ultrasonic oxygen sensor lie transducers. These devices transform electrical energy into ultrasonic waves and vice versa. Without them, the entire mechanism would collapse like a house of cards. Essentially, transducers generate sound waves at frequencies typically above 20 kHz, which is beyond the human audible range.
- Operation: When the transducer emits ultrasonic waves, they interact with oxygen molecules in the environment. Changes in the speed and frequency of the reflected sound waves provide insight into the concentration of oxygen present.
- Material: Piezoelectric materials, commonly used in these transducers, respond to electric currents by changing shape, generating the necessary sound waves. A common example is lead zirconate titrate (PZT).
- Benefits: Thanks to their rapid response time and ability to operate in various conditions, transducers facilitate precise measurements even in dynamic environments.
"The efficiency and accuracy of ultrasonic oxygen sensors fundamentally hinge on the proficiency of these transducers."
"The efficiency and accuracy of ultrasonic oxygen sensors fundamentally hinge on the proficiency of these transducers."
Microcontroller Integration
Microcontrollers serve as the brains of the ultrasonic oxygen sensor. They process the data collected from the transducers, allowing the system to analyze and interpret the signals received.
- Functionality: When the ultrasonic waves bounce back, the microcontroller analyzes the shift in frequency, correlating it to oxygen concentration levels. It performs complex calculations swiftly, ensuring timely readings.
- Flexibility: This integration brings adaptability. Microcontrollers can be programmed to meet specific industry requirements or can easily modify algorithms to enhance detection capabilities.
- Communication: Further, these chips can connect with other systems or networks. In modern applications, they can relay data to smartphones or central monitoring systems, enhancing usability and functionality.
Power Supply and Efficiency Considerations
Power supply considerations are pivotal for the practical application of ultrasonic oxygen sensors. The efficiency of the sensor hinges on how well it is powered while maintaining consistent and accurate readings.
- Source Types: Whether powered by batteries or mains electricity, the supply must cater to the sensor's demands without interruption. Lower consumption designs are always favorable to extend operational life in portable devices.
- Energy Management: Many modern sensors employ energy-saving features, like sleep modes, where components draw minimal power when not actively measuring, enhancing battery life significantly.
- Cost vs. Capability: There is always a balance to strike between affordability and quality. Superior power supply units may increase initial costs but can significantly enhance sensor performance and longevity.
By examining these key components—transducers, microcontrollers, and power management solutions—we can appreciate not only the sophistication underlying ultrasonic oxygen sensors but also their potential for transformative applications across various fields. As these components evolve, so too does the likelihood of broader adoption and innovation in sensor technology.
Advantages of Ultrasonic Oxygen Sensors
When diving into the world of ultrasonic oxygen sensors, it's crucial to appreciate the myriad of advantages they bring to the table. These sensors have carved a niche for themselves in various industries due to their unique mechanisms and functionalities. Below, we delve into three pivotal areas that highlight their strengths.
Non-Invasive Measurement Techniques
One of the standout features of ultrasonic oxygen sensors is their non-invasive measurement capabilities. Unlike traditional sensors that often require physical probes or invasive techniques, ultrasonic sensors utilize sound waves to gauge oxygen levels. This characteristic is particularly beneficial in medical applications, where patient comfort is paramount.
- Patient Comfort: By avoiding invasive procedures, these sensors minimize discomfort during oxygen level assessments, which is especially significant in monitoring patients with chronic respiratory issues.
- Enhanced Safety: Non-invasive methods reduce the risk of infections. This aspect is vital in healthcare settings where maintaining sterile environments is crucial.
- Operational Efficiency: These sensors can be integrated into existing monitoring systems without the need for extensive modifications, saving both time and resources.
Non-invasive techniques are a game changer for many sectors, and ultrasonic oxygen sensors are leading the charge.
Higher Precision and Sensitivity
When it comes to precision, ultrasonic oxygen sensors stand tall compared to their counterparts. The key reasons for this include:
- Advanced Signal Processing: The technology employed in these sensors allows for a high level of detail in capturing data. This ensures that subtle variations in oxygen levels can be detected promptly, providing users with vital information.
- Real-Time Monitoring: The ability to monitor changes in oxygen concentrations in real time enhances responsiveness, especially in critical situations like emergency medical care or industrial settings where air quality can fluctuate.
- Calibration Capability: These sensors can often be calibrated to rise to specific accuracy standards ensuring that even slight deviations are exhibited without delay.
Together, these elements craft a narrative of reliability and exceptional performance, ensuring they are trusted in applications where every ounce matters.
Adaptability to Various Environments
The adaptability of ultrasonic oxygen sensors makes them suitable for a range of applications across industries and environments. Here’s how:
- Robust Design: Many models are designed to withstand harsh conditions, from extreme temperatures to humidity, ensuring their longevity and functionality in diverse situations.
- Flexible Integration: Because they don't remake standard measurement frameworks, ultrasonic sensors can be incorporated easily into a variety of existing systems, whether in labs, manufacturing plant, or even the field.
- Versatility: Whether monitoring oxygen levels in medical environments, assessing air quality in industrial scenarios, or conducting research in environmental studies, these sensors perform remarkably well across the board.
In sum, the adaptability of these sensors doesn't just enhance their usability; it opens the door for continued innovations and applications in the future.
"The flexibility and ease of integration of ultrasonic oxygen sensors are game changers for monitoring air quality and ensuring public safety in various industries."
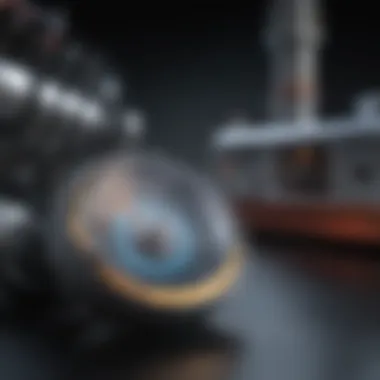
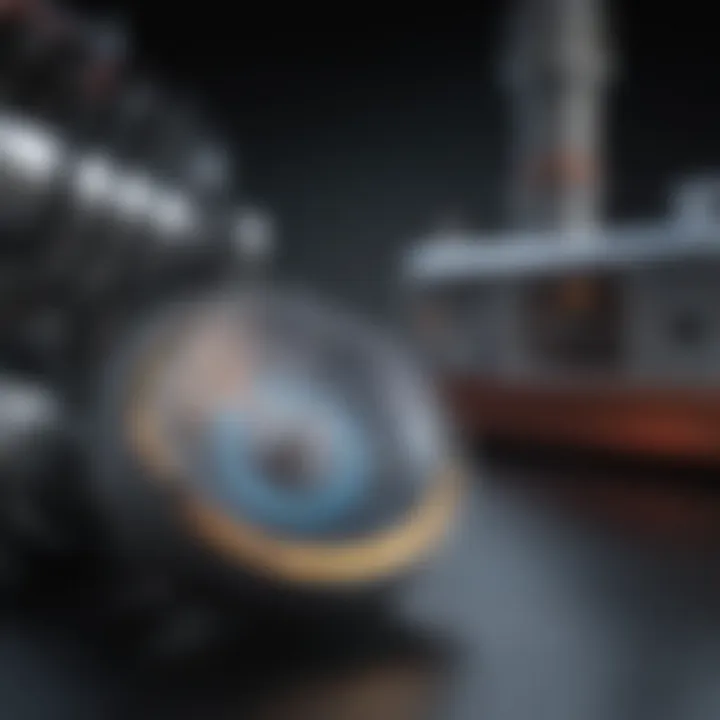
"The flexibility and ease of integration of ultrasonic oxygen sensors are game changers for monitoring air quality and ensuring public safety in various industries."
Ultrasonic oxygen sensors mark a significant advancement in the way we measure and analyze oxygen levels in various contexts, offering more than just a technological solution—they represent a leap towards greater efficiency, effectiveness, and convenience.
Applications Across Industries
The significance of ultrasonic oxygen sensors reaches far and wide, touching various sectors that benefit from precise and reliable oxygen measurement. As technology advances, these sensors are becoming more vital, not just for their scientific accuracy but due to their diverse applicability under different conditions. In medical, industrial, and environmental settings, ultrasonic oxygen sensors provide valuable insights that drive better decisions. This section delves into these applications, examining the unique contributions of ultrasonic technology to enhance processes and outcomes across multiple industries.
Medical Applications in Respirology
In the realm of healthcare, particularly in respirology, ultrasonic oxygen sensors play a pivotal role. These sensors enable non-invasive monitoring of blood oxygen levels, offering real-time data that healthcare professionals can use to make informed decisions. For instance, during surgeries or critical care, the accurate assessment of oxygen saturation can be literally lifesaving.
Furthermore, in chronic conditions like asthma or COPD (Chronic Obstructive Pulmonary Disease), these sensors help in tracking a patient’s parameters, ensuring timely interventions if levels drop. The precision that ultrasound technology brings is unmatched, often outperforming traditional methods that might lag in response or accuracy.
"The ability to monitor patients continuously without physical intrusion speaks volumes about the technological progress we’ve made in medical devices, particularly in managing respiratory conditions."
"The ability to monitor patients continuously without physical intrusion speaks volumes about the technological progress we’ve made in medical devices, particularly in managing respiratory conditions."
This not only enhances patient comfort but also provides a wealth of data over time for better diagnosis and treatment plans.
Industrial Uses in Manufacturing
Turning our gaze to the manufacturing floor, ultrasonic oxygen sensors are changing the game in quality control and process optimization. In industries where the presence of oxygen could threaten product quality—think food packaging or materials processing—the ability to monitor and adjust oxygen levels precisely is crucial.
For example, in food manufacture, atmosphere modification techniques often require precise levels of oxygen for preserving the quality and extending shelf life. Ultrasonic sensors can effectively monitor these levels, helping maintain optimal conditions without the need for complex systems.
- Key Benefits Include:
- Enhanced product integrity through more accurate oxygen measurement.
- Reduced waste from over-packaging or spoilage.
- Streamlined processes that save time and resources.
Additionally, in environments with hazardous materials, continuous oxygen monitoring is essential for safety protocols. By integrating ultrasonic sensors into existing manufacturing lines, businesses can safeguard not just the products but also the people working with them.
Environmental Monitoring and Research
In today's era where environmental concerns are paramount, ultrasonic oxygen sensors have carved a niche in ecological monitoring. Their application extends to tracking oxygen levels in various ecosystems, essential for understanding and mitigating the effects of pollution and climate change.
Research facilities have begun to employ these sensors in studies related to aquatic systems, where dissolved oxygen levels are critical indicators of water quality.
Here’s how these sensors contribute:
- Ecosystem Health Assessment: Regular monitoring helps in evaluating the health of aquatic life.
- Pollution Tracking: Identifying areas of decline in oxygen levels can signal pollution events early, prompting immediate action.
- Data for Regulations: Gathering accurate data offers vital support for environmental regulations and compliance.
The versatility and responsiveness of ultrasonic sensors allow researchers to conduct long-term studies that reveal trends and impacts of environmental changes.
Challenges and Limitations
When discussing ultrasonic oxygen sensors, it is crucial to recognize that, like any technology, they come with their own set of challenges and limitations. Understanding these issues not only aids in appreciating the current capabilities of these sensors but also emphasizes the areas that require further research and development. In this section, we will explore various factors such as environmental interference, calibration and maintenance needs, and cost considerations that play significant roles in the practical application of ultrasonic oxygen sensors.
Environmental Interference Issues
Ultrasonic oxygen sensors operate by emitting and receiving ultrasonic waves, which makes them susceptible to various forms of environmental interference. External factors such as temperature fluctuations, humidity, and pressure changes can significantly affect the accuracy of these sensors. For instance, high levels of noise or vibrations in industrial settings can mask the ultrasonic signals, leading to erroneous readings. Additionally, the presence of other gases or particulate matter in the air can alter the behavior of sound waves, complicating the detection process.
To mitigate these challenges, sensor designers often employ advanced filtering techniques and algorithms to minimize the noise impact. However, this necessitates a more robust design and can potentially increase costs. Therefore, addressing environmental interference is not just about understanding limitations; it's also about finding strategies to overcome them for enhanced sensor reliability.
Calibration and Maintenance Requirements
Proper calibration of ultrasonic oxygen sensors is vital for ensuring precision in measurements. As sensors age or operate in varying conditions, their performance can deviate from expected readings. Regular calibration aligns the sensor outputs with accurate values, which is essential for both research and practical applications.
Moreover, recalibration often involves specific procedures that can be time-consuming and require technical skills. This need for regular maintenance can create barriers for organizations, especially smaller ones that may not have the resources for meticulous compliance with these procedures. The knowledge gap can also hinder effective sensor implementation—users must be educated on maintenance protocols to ensure long-term functionality.
Cost Factors and Market Accessibility
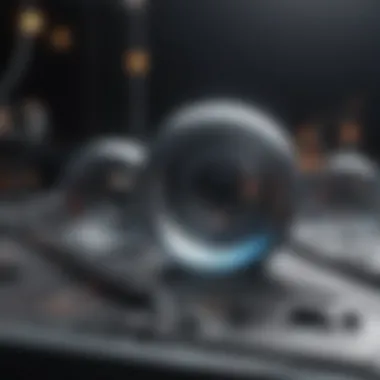
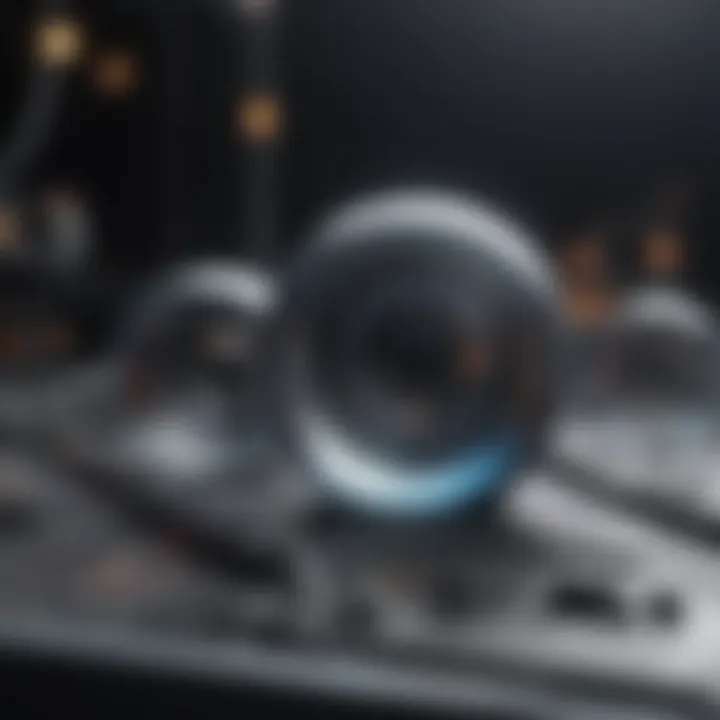
Economic constraints often play a fundamental role in the adoption of any technology, and ultrasonic oxygen sensors are no exception. The costs associated with high-quality sensors can be prohibitive for many businesses. Factors such as research and development expenses, coupled with the sophisticated manufacturing process, drive up the price.
In addition, the market accessibility of these sensors can vary significantly from region to region. This disparity might limit opportunities for innovation and widespread adoption, particularly in developing nations. Establishing a balance between performance, cost, and accessibility is essential. Better solutions may arise from collaboration among manufacturers, researchers, and policymakers aimed at reducing costs while maintaining high standards of quality.
With advancing technology, certain innovations may lower production costs and improve accessibility, but the path to achieving this is paved with acknowledged challenges.
With advancing technology, certain innovations may lower production costs and improve accessibility, but the path to achieving this is paved with acknowledged challenges.
Future Directions in Ultrasonic Oxygen Sensing
The exploration of future directions in ultrasonic oxygen sensing bears significant importance in understanding how these devices can evolve and enhance their roles in various applications. As industries and technologies progress, the demand for more sophisticated and efficient sensing mechanisms becomes paramount. This section delves into exciting prospects that could reshape how ultrasonic sensors function, paving the way for innovation.
Emerging Technologies in Sensor Design
Advancements in sensor design are poised to revolutionize ultrasonic oxygen sensors. The emergence of nanotechnology has opened doors for the creation of more sensitive and compact sensors. For example, utilizing nanomaterials can significantly increase the surface area for reactions, translating to faster detection times and improved accuracy. Moreover, integrating micro-electromechanical systems (MEMS) technology allows for the development of smaller, lightweight sensors that can be deployed in tight spaces without compromising performance.
- Potential benefits include:
- Enhanced sensitivity and precision
- Smaller form factors making them more versatile
- Lower manufacturing costs in the long run
Additionally, the application of machine learning algorithms in signal processing could learn from patterns in data to improve performance over time. This means that future sensors may not only detect oxygen levels but also predict trends and anomalies based on historical data.
Integration with Smart Technologies
The integration of ultrasonic oxygen sensors with smart technologies holds immense potential. As more devices become interconnected, enhancing data analytics and real-time monitoring capabilities is vital. Sensors that communicate with smart systems can provide continuous feedback to users, allowing for immediate responses to any detected changes.
Imagine a smart healthcare system where ultrasonic oxygen sensors continuously feed data to healthcare providers, enabling them to monitor patients remotely. Similarly, in industrial settings, these sensors can blend seamlessly into existing IoT frameworks, facilitating predictive maintenance and improving operational efficiency.
- Key considerations for this integration include:
- Reliability and security in data transmission
- Scalability to accommodate various applications
- User-friendly interfaces for accessibility
Sustainability and Environmental Impact
As the world shifts towards more sustainable practices, the development of ultrasonic oxygen sensors must consider their environmental impact. Future designs could focus on utilizing eco-friendly materials and more energy-efficient technologies, reducing the carbon footprint associated with their production and operation.
Moreover, improving recycling and end-of-life strategies for these sensors can contribute to reducing electronic waste. Research into biodegradable components and sustainable production methods presents an exciting avenue for development. With an emphasis on circular economy practices, ultrasonic oxygen sensors can align better with global sustainability goals.
The adaptation of these devices to have minimal environmental effects not only enhances their acceptance in sensitive applications but also aligns with regulatory trends prioritizing eco-friendliness.
"The future of ultrasonic oxygen sensors will not only be about accuracy but also about their impact on the world around us."
"The future of ultrasonic oxygen sensors will not only be about accuracy but also about their impact on the world around us."
Closure: The Significance of Ultrasonic Oxygen Sensors
In light of current advancements, the significance of ultrasonic oxygen sensors becomes increasingly clear. These tools are not just novel gadgets; they serve pivotal roles in multiple arenas — from healthcare to environmental monitoring. Understanding their mechanisms and capabilities opens the door to leveraging these sensors effectively for enhanced public health, industrial efficiency, and ecological balance.
Reflecting on Current Research and Innovations
Modern research in the field of ultrasonic oxygen sensing is shedding light on various innovative applications. The integration of machine learning algorithms with sensor data is a notable trend. This partnership enhances the accuracy of the readings, transcending conventional limitations faced by earlier technologies.
Moreover, ongoing studies are examining new materials that can improve the performance and durability of ultrasonic sensors. For instance, researchers are exploring the use of piezoelectric materials that can more effectively convert energy from sound waves into data. These innovations not only aim to enhance sensitivity but also to reduce the overall costs of manufacturing, making these sensors more accessible.
In parallel, there's a tangible interest in miniaturization. The drive to create compact, lightweight sensors opens up new horizons in various fields. Medical applications, particularly in respirology, benefit significantly from portable designs that can be used in a patient’s home. The ability to track oxygen levels in real-time without invasive procedures is a game-changer.
"The future of ultrasonic oxygen sensors lies in their seamless integration with other technologies, paving the way for smarter healthcare solutions."
"The future of ultrasonic oxygen sensors lies in their seamless integration with other technologies, paving the way for smarter healthcare solutions."
The Role in Future Scientific Endeavors
As we look toward the horizon, ultrasonic oxygen sensors have the potential to play critical roles in several emerging scientific endeavors. One particularly exciting prospect is their application in climate monitoring. These sensors can provide continuous data regarding atmospheric oxygen levels, helping scientists comprehend changes in our environment and guiding efforts to combat climate change.
Additionally, their multifunctionality may lead to the development of multipurpose sensors that could monitor several gases anomalously. Combining oxygen detection with measurement capabilities for carbon dioxide and methane, for instance, could offer invaluable insights into air quality and pollution levels.
In the healthcare industry, potential applications extend to remote patient monitoring systems that utilize ultrasonic sensors, allowing healthcare providers to gather necessary respiratory data without being physically present. These innovations could be revolutionary in addressing rural healthcare accessibility issues.
The synergy between ultrasonic technology and advances in artificial intelligence could further amplify the sensors' capabilities. Predictive analytics can be employed to forecast health crises based on oxygen levels and their fluctuations, advancing proactive healthcare measures.