Understanding Copper's Lattice Structure and Properties
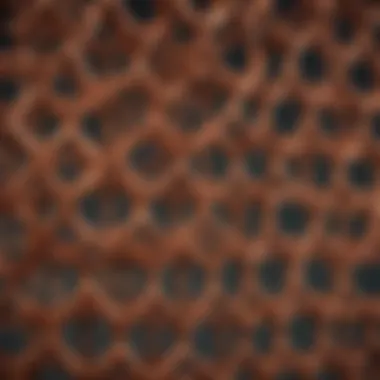
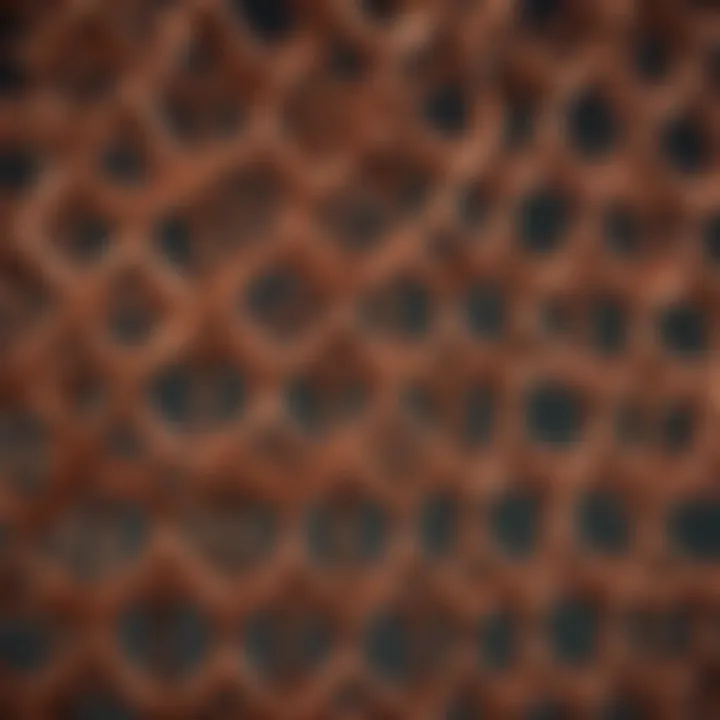
Intro
Copper is a metal that has fascinated scientists and engineers for centuries. Its properties and applications are vast and varied. Understanding copper's lattice structure is crucial for grasping why it behaves the way it does in different environments. The atomic arrangement plays a significant role in determining its electrical conductivity, thermal conductivity, and mechanical malleability. This article aims to present a detailed overview of the copper lattice structure, focusing on its atomic arrangement, related physical properties, and implications in various scientific fields.
Key Concepts
Definition of the Main Idea
The copper lattice structure refers to the orderly arrangement of copper atoms in a crystalline form. This arrangement dictates many of copper's outstanding properties. In solid-state physics and materials science, lattice structures are essential for understanding how materials will perform under different conditions. Typically, copper crystallizes in a face-centered cubic (FCC) structure, which enhances its ability to conduct electricity and heat.
Overview of Scientific Principles
The lattice structure involves several scientific principles. Atoms in a lattice are arranged periodically, and this periodicity affects how the atoms interact with each other. The lattice parameters, which define the distances between atoms, are critical in determining the strength and flexibility of the metal. Additionally, interatomic forces such as metallic bonding influence the overall characteristics of copper.
Key properties stemming from its lattice structure include:
- Electrical Conductivity: High due to the freedom of movement of electrons in the FCC structure.
- Thermal Conductivity: Excellent because of efficient energy transfer among atoms.
- Malleability: The ability to undergo deformation without breaking, aided by its atomic arrangement.
Current Research Trends
Recent Studies and Findings
Ongoing research on copper's lattice structure aims to enhance its properties for better performance in various applications. Recent studies have focused on exploring new alloys and composite materials that incorporate copper as a primary element. Modifications in lattice parameters through alloying or heat treatment can lead to improved qualities.
Notable findings suggest that slight variations in crystal structure can substantially influence mechanical attributes. For instance, research has shown that doping copper with other elements can lead to desirable changes in strength and resistance to corrosion.
Significant Breakthroughs in the Field
A significant breakthrough in this domain is the development of nanoscale copper materials. At such scales, quantum effects become pronounced, affecting not only mechanical properties but also electronic characteristics. These innovations open new avenues in electronic engineering and nanotechnology, emphasizing the relevance of understanding copper's atomic structure more deeply.
"Understanding the atomic arrangement of copper not only enhances its applications but also can lead to breakthroughs in various scientific and industrial fields."
"Understanding the atomic arrangement of copper not only enhances its applications but also can lead to breakthroughs in various scientific and industrial fields."
In summary, the exploration of copper's lattice structure is a multidimensional topic, relevant in both theoretical and practical applications. By understanding the foundational principles, recent advancements, and future implications, we can appreciate copper's critical role in technology and industry.
Preface to Copper
Copper is a metal that has been integral to human civilization for thousands of years. From its use in ancient tools and weapons to its critical role in modern electronics, copper's importance cannot be understated. Understanding copper's lattice structure is essential, as it provides key insights into the properties that make it valuable across a wide range of applications. The atomic arrangement within copper lattices influences its electrical and thermal conductivities, malleability, and overall performance in various industrial processes.
Historical Context
The history of copper dates back to around 10,000 years ago, when it was first used in the form of simple tools and decorative items. Among the first civilizations to utilize copper were the Sumerians, Egyptians, and various cultures in the Americas. These early applications demonstrated copper's utility as a conductor of heat and electricity. Over time, as techniques for mining and smelting improved, the production of copper increased, leading to its widespread adoption in various sectors, including construction and manufacturing.
The alloying of copper with tin to produce bronze marked a significant leap in technological advancement, fostering the Bronze Age and impacting social and economic structures. Today, copper is regarded as one of the most significant industrial metals, accounting for substantial portions of materials used in electrical wiring, plumbing, and renewable energy technologies.
Significance in Modern Applications
In today's world, copper serves a multitude of purposes, making it a cornerstone material in multiple industries. Its excellent electrical conductivity makes it indispensable for electrical wiring and components in gadgets, ensuring efficient power distribution. The demand for copper in electrical applications is increasing, driven by the growth of renewable energy technologies, particularly solar panels and wind turbines.
Furthermore, copper's resistance to corrosion contributes to its prominent role in plumbing and architectural applications. Copper alloys, such as brass and bronze, enhance its properties, making these materials suitable for a broad spectrum of uses that require durability and appearance.
In summary, the importance of copper in both historical and modern contexts establishes a foundational understanding of its lattice structure. This structure underpins many of its applications, which will be explored in detail throughout this article.
"Copper, with its unique properties and versatility, continues to be an essential material in advancing technology and shaping our everyday lives."
"Copper, with its unique properties and versatility, continues to be an essential material in advancing technology and shaping our everyday lives."
Overall, acknowledging copper's historical significance and diverse applications allows us to appreciate the depth of knowledge required to understand its lattice structure and the subsequent implications on its use in modern society.
Fundamentals of Crystallography
Crystallography forms the backbone of materials science, specifically in understanding how materials like copper exhibit their unique properties at an atomic level. This section delves into the essential principles underlying crystalline structures and paves the way to appreciate the intricacies of the copper lattice. By understanding these fundamentals, one can interpret how atomic arrangements impact physical characteristics such as conductivity and strength, making it invaluable for students, researchers, and industry professionals.
Crystal Systems
In crystallography, crystal systems represent distinct patterns of atomic arrangements. There are seven primary crystal systems: cubic, tetragonal, orthorhombic, hexagonal, rhombohedral, monoclinic, and triclinic. Each system is characterized by specific symmetry and axis lengths, crucial for defining the ultimate lattice structure of a material.
Copper crystallizes in a face-centered cubic (FCC) structure, which enhances its ductility and malleability. This system allows for closer atomic packing, resulting in greater strength and electrical conductivity, two critical attributes in industrial applications. Each crystal system influences how the atoms are organized, which in turn, affects the lattice’s overall behavior under various conditions. Understanding these systems is therefore vital when exploring the behavior of copper in different environments.
Lattice Parameters
Lattice parameters are fundamental to describing the size and geometry of a crystal unit cell, specifically in the context of the crystals formed by copper. These parameters, such as the lengths of the unit cell edges and the angles between them, help define the spatial arrangement of atoms within the lattice.
In the case of copper, the lattice parameters manifest specific values that have significant implications for its physical properties. The lattice constant of copper, for example, measures approximately 3.61 angstroms. This compact structure leads to a high packing efficiency and explains copper's superior conductivity and malleability.
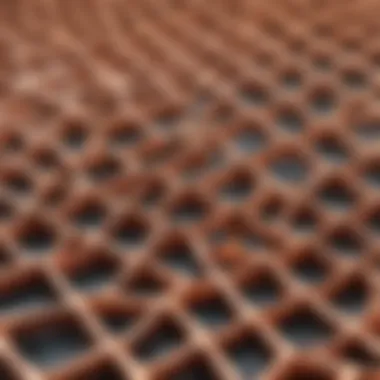
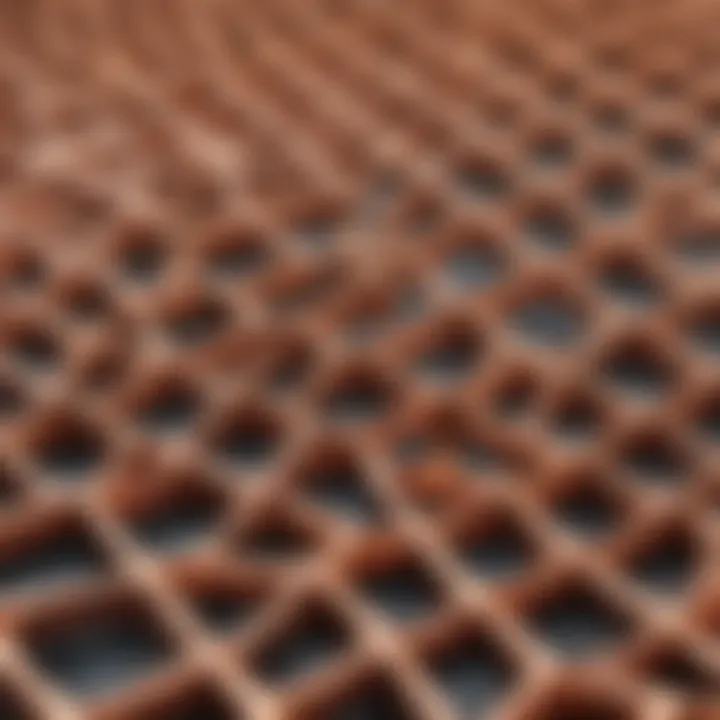
Understanding lattice parameters is crucial when predicting how different forms of copper engage in chemical interactions or how they respond to physical forces. For researchers and professionals, precise knowledge of these values allows for better tailoring of materials for specific applications, particularly in fields such as electronics and construction.
Understanding the specific elements of crystallography not only deepens one's knowledge of copper but also extends to the practical realms of material design and application.
Understanding the specific elements of crystallography not only deepens one's knowledge of copper but also extends to the practical realms of material design and application.
Copper's Atomic Structure
Copper has a unique atomic structure that is essential to its various properties and applications. Understanding this structure is critical as it forms the foundation for explaining other aspects of copper, such as its electrical and thermal conductivity. Copper's atomic arrangement and electron configuration determine how this metal interacts with other elements, influencing its behavior in different environments and its integration into various technologies. This section aims to explore these dimensions in detail, allowing readers to appreciate why copper is valued across disciplines and industries.
Atomic Arrangement
The atomic arrangement of copper is characterized by its face-centered cubic (FCC) lattice structure. In this arrangement, each copper atom occupies one corner of the cube and the center of each face. This configuration is notable because it enables the atoms to pack closely together, resulting in high density and enhanced stability.
- FCC Structure: The close-packed layers of atoms in the FCC structure allow copper to maintain its durability while remaining malleable. This means that it can be shaped into various forms without breaking, making it suitable for applications ranging from wire production to architectural elements.
- Coordination Number: In this lattice, each copper atom has twelve nearest neighbors. The high coordination number contributes to the metallic bonding within copper, leading to its remarkable physical properties.
In summary, the atomic arrangement of copper is not just a random distribution of atoms; it is a well-defined structure that allows for the unique combination of properties that make copper so valuable.
Electron Configuration
The electron configuration of copper plays a crucial role in its chemical behavior. The configuration is [Ar] 3d¹⁰ 4s¹, where argon (Ar) represents the noble gas core. This configuration reveals how electrons are distributed across different energy levels, ultimately affecting the conductivity and reactivity of copper.
- Conductivity: The presence of a single electron in the 4s orbital is significant. This free electron is responsible for copper's excellent electrical conductivity, as it allows for the smooth flow of electric current.
- Reactivity: Although copper is considered relatively inert, its electron configuration allows for specific reactions, especially with nonmetals. This capability makes it a key component in numerous alloys and compounds.
Characteristics of the Copper Lattice Structure
Understanding the characteristics of the copper lattice structure is essential for appreciating its myriad applications and qualities. The structure of copper, particularly its lattice formation, greatly influences its properties such as conductivity, malleability, and mechanical strength. Discerning these characteristics helps in various fields, from materials science to electrical engineering. Therefore, it is crucial to delve into specific elements like lattice types and packing efficiency, which shed light on how these properties manifest in practical scenarios.
Lattice Types
Copper predominantly crystallizes in a face-centered cubic (FCC) lattice structure. This arrangement is notable for several reasons:
- High Symmetry: The FCC structure is symmetrical, which contributes to uniform physical properties in all directions. This isotropy is particularly beneficial in applications requiring consistent conductivity and mechanical strength.
- Coordination Number: In an FCC lattice, each copper atom is surrounded by twelve nearest neighbors, enhancing the metal's ability to conduct electricity efficiently. It enables a vast number of pathways for electrons to move through the material.
- Interatomic Forces: The geometry of the FCC structure allows for effective packing of atoms. The interatomic forces at play in this arrangement lead to fewer disruptions in the electron flow, resulting in excellent electrical conductivity compared to other lattice forms.
While FCC is the predominant structure, variations exist under different conditions. For example, during alloying or phase transitions, the lattice might deviate to a body-centered cubic (BCC) structure, although this is less common in pure copper.
Density and Packing Efficiency
The packing efficiency in a lattice structure refers to how effectively atoms occupy space within the crystal framework. For copper, the FCC structure offers a high packing efficiency of about 74%. This characteristic has several implications:
- Material Strength: High packing efficiency contributes to the mechanical strength of copper. Tightly packed atoms result in a higher resistance to deformation, which is particularly important in applications requiring durability.
- Conductivity: The density of the lattice impacts both thermal and electrical conductivity. A more densely packed structure facilitates better electron flow and heat conduction. This concept is critical when assessing copper's suitability for electrical wiring or heat exchangers.
- Role in Alloys: In addition to pure copper, packing efficiency plays a role in the performance of copper alloys. Elements added to copper can alter both the density and the arrangement of atoms in the lattice, leading to changes in the physical properties. For example, adding tin to create bronze introduces different lattice structures, optimizing certain mechanical properties.
Understanding these characteristics enables researchers and engineers to manipulate copper's properties effectively, making it suitable for a wide range of applications in modern technology and industry. As further insights are attained through experimental methods, the adaptability of copper's lattice structure remains a focal point in material science.
Physical Properties Derived from Lattice Structure
The copper lattice structure plays a crucial role in defining the physical properties of copper. Understanding these properties is vital for various applications, from electrical engineering to materials science. The atomic arrangement within the lattice influences how copper interacts with heat and electricity, contributing to its wide-ranging uses. This section will specifically explore two key properties: electrical conductivity and thermal conductivity.
Electrical Conductivity
Electrical conductivity is one of the most recognized characteristics of copper. The high conductivity of copper can be attributed to its face-centered cubic lattice structure. This arrangement allows electrons to move freely, facilitating the flow of electric current.
- Key factors influencing conductivity:
- Atomic arrangement: The close packing of copper atoms provides minimal resistance to electron flow.
- Electron mobility: Copper has a high density of free electrons, which enhances its conductivity.
- Temperature effects: As temperature increases, some resistance builds up, but copper remains one of the best conductors among metals.
"Copper's exceptional conductivity is why it is commonly used in wiring and electronic circuits."
"Copper's exceptional conductivity is why it is commonly used in wiring and electronic circuits."
Understanding the specifics of the conductivity can guide engineers and researchers in selecting materials for specific applications, ensuring efficiency and reliability.
Thermal Conductivity
Thermal conductivity is another critical physical property associated with copper’s lattice structure. Copper exhibits high thermal conductivity, allowing it to transfer heat effectively. This property is particularly important in thermal management applications.
- Influencing elements:
- Lattice arrangement: Similar to electrical conductivity, the atomic structure permits efficient energy transfer.
- Low thermal resistance: The packed structure allows for rapid movement of heat through the material.
- Phase transitions: Changes in temperature can affect the lattice, but copper generally maintains its thermal performance across a range of conditions.
Copper's role in conductors like heat sinks and cookwares is largely due to its thermal properties. Decisions in materials for heat-related applications often weigh copper's advantages against other metals.
In summary, the physical properties derived from the copper lattice structure are significant in various fields. Knowing how the lattice structure affects electrical and thermal conductivity can lead to better material choices and innovative applications in technology and industry.
Chemical Interactions and Copper Alloys
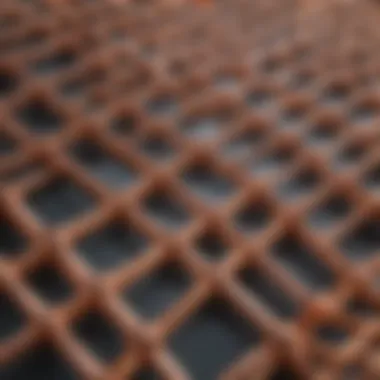
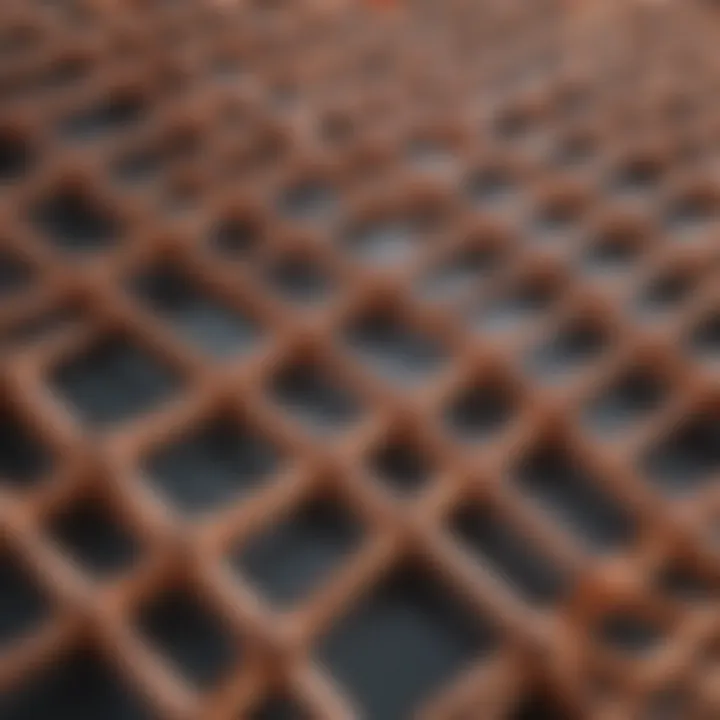
Understanding chemical interactions and their influence on copper alloys is essential in appreciating the broader context of copper's applications in modern technology. The process by which copper can be manipulated and improved through alloy formation is a nuanced area of study. These interactions not only alter the physical properties of copper but also expand its functional capabilities, making it a central material across diverse industries.
Common Copper Alloys
Copper alloys are materials composed predominantly of copper, often mixed with other elements to enhance specific properties. The most significant copper alloys include:
- Brass: Made from copper and zinc, brass is known for its strength and corrosion resistance. It is commonly used in plumbing and electrical fittings.
- Bronze: This alloy typically contains copper and tin. Bronze exhibits excellent resistance to wear and is often found in marine applications and sculptures.
- Copper-Nickel Alloys: These are utilized predominantly in marine environments due to their resistance to seawater corrosion. They are also employed in manufacturing coins.
The choice of alloying elements is critical. It determines factors such as strength, ductility, thermal conductivity, and even susceptibility to corrosion. This versatility underlines why copper remains a fundamental choice for various applications.
Impact on Lattice Structure
Copper alloys reveal interesting transformations in lattice structures compared to pure copper. When new elements are introduced into the copper lattice, several changes occur:
- Modification of Lattice Parameters: The addition of alloying elements can cause distortions in the lattice structure, affecting its dimensions. This leads to changes in mechanical properties such as tensile strength and malleability.
- Interatomic Bonding Changes: Different elements may introduce new types or strengths of bonding within the lattice, altering conductivity and thermal characteristics. For instance, the presence of zinc alters the conductivity of brass compared to pure copper.
- Phase Formation: Alloys often form distinct phases during solidification, which can impact their properties significantly. Understanding the relationship between phase composition and characteristics is vital for engineers and researchers.
In summary, the interactions between copper and alloying elements fundamentally reshuffle its crystalline structure, leading to noticeable changes in physical properties.
In summary, the interactions between copper and alloying elements fundamentally reshuffle its crystalline structure, leading to noticeable changes in physical properties.
The study of the copper lattice is deepened by exploring these interactions and modifications, enabling engineers to predict how new alloys will behave under different conditions. This aspect is crucial for industries ranging from electronics to construction.
Understanding this interplay between copper and its alloys will continue to guide innovations and applications in material science.
Applications of Copper Lattice Structure
The copper lattice structure is not just an academic concept; it shapes many practical applications across various fields. Understanding this structure helps reveal the reasons behind copper’s unique properties. The atomic arrangement within the lattice significantly contributes to both electrical and thermal conductivity, making copper indispensable in many industries. With the increasing demand for efficient materials, examining the applications of copper lattice structures is critical.
Electrical Applications
Copper's lattice structure proves essential in electrical applications due to its high electrical conductivity. This characteristic arises from the arrangement of copper atoms, which facilitates the flow of electrons. The close-packed lattice allows for minimal resistance. Consequently, copper is often used in wiring, connectors, and circuit boards.
- Wiring: In households and industries alike, copper wiring is the backbone of electrical systems. Its ability to conduct electricity efficiently reduces energy loss, making it a preferred choice.
- Connectors: Copper connectors link different electrical components. Their effective conduction is crucial for reliable operation.
- Circuit Boards: In electronics, copper’s lattice structure contributes to the performance of printed circuit boards. Efficient heat dissipation and low energy consumption make it invaluable.
The continual use of copper in electrical components proves how the lattice structure underpins these applications, ensuring reliability and efficiency. More details can be found on Wikipedia.
Architectural Uses
In architecture, the aesthetic and functional qualities of copper come into play. Its lattice structure not only influences its physical properties but also affects its behavior in architectural applications. Buildings often utilize copper for both functional and decorative purposes.
- Roofing: Copper roofs are renowned for their durability and resistance to corrosion. The lattice structure contributes to strength, allowing copper roofs to withstand harsh weather over long periods.
- Facades: Buildings often feature copper cladding due to its appealing appearance. The patina that forms over time adds character and can reduce maintenance needs.
- Plumbing: Copper pipes are commonly used in plumbing systems. The atomic arrangement provides excellent resistance to corrosion, ensuring longevity and reliability.
Copper’s versatility in architectural applications shows how its lattice structure directly influences practical utility and visual appeal.
Copper’s versatility in architectural applications shows how its lattice structure directly influences practical utility and visual appeal.
In essence, understanding copper's lattice structure allows for a deeper comprehension of its various applications, encouraging innovation and efficiency in several fields.
Experimental Techniques for Investigating Lattice Structure
The study of copper lattice structure encompasses various experimental methods to analyze and characterize its arrangement at the atomic level. These techniques are critical for understanding how the atomic organization influences the material's properties. In this section, we will explore significant methods like X-ray diffraction and electron microscopy. Each of these techniques provides unique insights into the lattice's geometrical and electronic characteristics, essential for guiding research and industrial applications.
X-ray Diffraction
X-ray diffraction (XRD) stands as a cornerstone technique in crystallography. This method provides vital information on the crystal structure of materials, including copper. When X-rays are directed at a crystalline sample, they interact with the electron cloud around the atoms, causing scattering. The scattered rays produce a diffraction pattern that is characteristic of the crystal structure. Scientists analyze this pattern to determine parameters such as lattice spacing and symmetry.
The main benefits of using XRD include:
- Non-destructive testing: XRD can analyze samples without altering their physical state.
- High accuracy: This technique offers precise measurements of lattice parameters, helping scientists understand the material better.
- Broad applicability: It can be employed for various materials beyond copper, aiding comparative studies.
Moreover, XRD can help identify phase transitions and the presence of alloying elements in copper samples.
X-ray diffraction is a powerful tool for determining the crystal structure, helping researchers design better materials based on copper.
X-ray diffraction is a powerful tool for determining the crystal structure, helping researchers design better materials based on copper.
Electron Microscopy
Electron microscopy represents another pivotal method for analyzing the copper lattice. Unlike X-ray diffraction, which provides average information over larger areas, electron microscopy focuses on the individual grains within the crystal. There are two primary types of electron microscopy used: Scanning Electron Microscopy (SEM) and Transmission Electron Microscopy (TEM).
- Scanning Electron Microscopy (SEM): This involves scanning a focused beam of electrons across the surface of a copper sample to create detailed images. SEM can reveal surface morphology and topography information, allowing the examination of structures at nanoscale levels.
- Transmission Electron Microscopy (TEM): TEM allows scientists to observe the internal structure of materials at the atomic level. A thin sample of copper is bombarded with electrons, which pass through the sample. This technique gives high-resolution images and detailed information about the arrangement of atoms.
Both methods yield valuable data about crystallographic defects, grain boundaries, and phase separation, crucial for understanding the performance of copper in various applications. By utilizing electron microscopy, researchers can correlate microstructural features with chemical and physical properties, ultimately driving innovation in the field.
Theoretical Models of the Copper Lattice
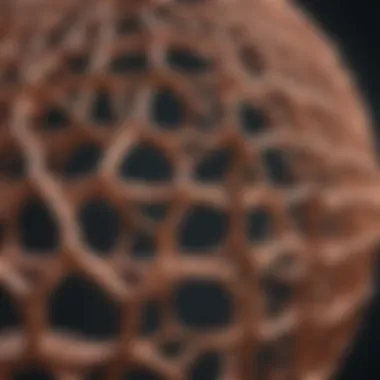
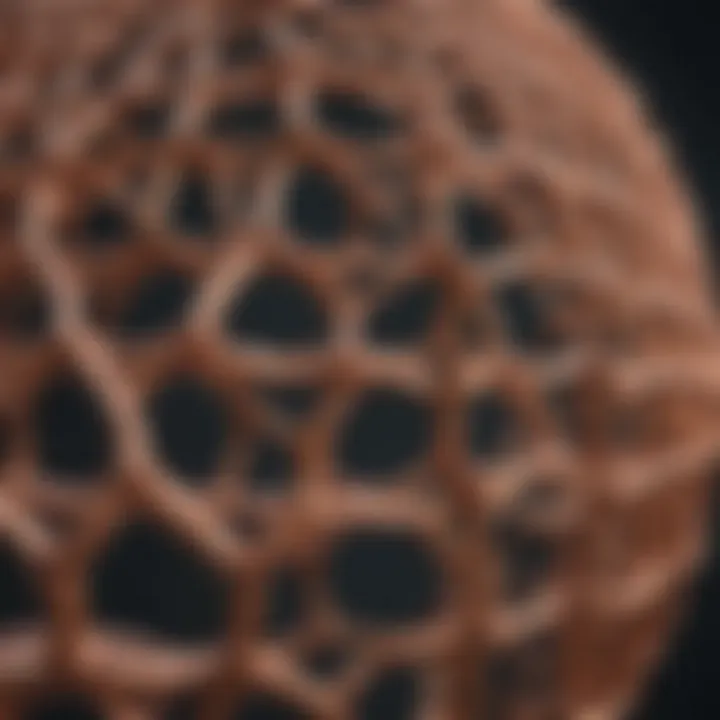
The study of the copper lattice structure requires a strong foundation in theoretical models. These models are crucial to understanding how copper behaves at the atomic level. They provide insights into the arrangement of atoms and predict material properties. Quantum and classical models play significant roles in this field.
Understanding theoretical models helps researchers predict how copper can be manipulated and utilized in various applications. This becomes especially important as technology progresses and new alloys or applications for copper emerge. Knowing these models supports advancements in material science and engineering.
Quantum Mechanical Models
Quantum mechanical models offer a precise framework for examining the copper lattice. They consider the principles of quantum mechanics to explain the behavior of electrons around copper atoms. In these models, the wave-particle duality of electrons is a fundamental concept. This duality affects how atoms interact, leading to the formation of the lattice structure.
Key aspects of quantum mechanical models include:
- Electron Orbital Theory: This theory illustrates how electrons cloud around atomic nuclei, affecting bonding and interactions.
- Energy Bands: Electrons in copper occupy specific energy levels. These bands determine electrical conductivity and other physical properties.
- Interatomic Forces: Quantum models account for the forces between copper atoms. These forces dictate the stability and arrangement of the lattice.
Classical Models
Classical models provide a contrasting approach to the study of copper lattices. These models are based on classical physics principles and simplify atomic behavior. They often assume that atoms are solid spheres, focusing on interactions and forces between them. While less precise than quantum models, they offer valuable insights, especially when exploring larger-scale phenomena.
Elements of classical models include:
- Newtonian Mechanics: This applies to the movement and interaction of atoms, enabling calculations of forces and potential energies.
- Lennard-Jones Potential: This mathematical model is common in describing the interactions between non-bonded atoms, critical in lattice stability analysis.
- Heating Effects: Classical models analyze how heat influences atomic behavior, particularly relevant in copper's thermal properties.
Both quantum and classical models contribute uniquely to the study of copper lattices. While quantum models offer precision, classical models provide essential approximations. Researchers often combine these approaches for a more comprehensive understanding. Their collective insights enhance our grasp of copper’s applications, influencing future materials research.
Future Directions in Copper Lattice Research
The exploration of copper lattice research is crucial as it drives innovation in both academia and industry. As the demand for advanced materials grows, understanding the intricacies of the copper lattice structure becomes more essential. Several factors influence the trajectories of this research, including technological advancements, environmental sustainability, and the quest for improved material properties.
Emerging techniques and methodologies in nanostructuring and alloy development play a significant role in this evolution. Researchers aim to enhance the properties of copper by studying its behavior at the nanoscale and creating new alloys that exhibit superior characteristics. Both aspects not only contribute to theoretical understandings but also to practical applications in various fields such as electronics, energy storage, and structural engineering.
"The future developments in copper lattice research will likely shape the next generation of materials, influencing everything from electronics to renewable energy."
"The future developments in copper lattice research will likely shape the next generation of materials, influencing everything from electronics to renewable energy."
Furthermore, the incorporation of computational modeling and simulations is improving our ability to predict how modifications to the lattice could affect physical properties. This synergy between empirical research and theoretical frameworks facilitates a more profound understanding of copper and its alloys.
Nanostructuring Techniques
Nanostructuring techniques are emerging as vital methods for enhancing the physical properties of copper. These techniques often involve manipulating the atomic structure of copper at the nanoscale, resulting in materials with improved strength, ductility, and conductivity. The use of methods such as chemical vapor deposition and laser ablation are advancing research tremendously.
Benefits of these techniques include:
- Enhanced Conductivity: Nano-sized grains can reduce barriers for electron flow, increasing electrical conductivity.
- Improved Mechanical Properties: Smaller, more uniform grains lead to enhanced strength and toughness.
- Tailored Properties: Researchers can design materials with specific attributes for targeted applications.
Researchers continue to investigate how these techniques influence copper's lattice structure, with potential implications for the development of nanocomposites and other advanced materials.
Potential New Alloys
Research into potential new alloys offers a promising avenue for enhancing the properties of copper. By alloying copper with various elements, scientists can create materials that capitalize on the strengths of both copper and other metals. For example, adding elements such as aluminum or magnesium may improve strength while preserving copper’s excellent conductivity.
Key considerations when developing new alloys include:
- Compatibility: Ensuring the alloying elements do not compromise copper's inherent properties.
- Cost-Effectiveness: Utilizing readily available materials to keep production feasible.
- Environmental Impact: Considering the sustainability of raw materials and the impact on the environment.
Potential new copper alloys are likely to be applied in various sectors, including electronics, aerospace, and renewable energy systems, making ongoing research vital for future developments.
The resultant insights from these studies not only contribute to theoretical models but also to practical advancements that could revolutionize material science.
Closure
The conclusion of this article serves as a critical synthesis of the various themes and discussions surrounding the copper lattice structure. It highlights the significance of understanding copper's unique atomic arrangement and its implications across numerous fields, from materials science to electrical engineering. Recognizing copper's role in shaping contemporary technology and industry bolsters the motivation for further study and exploration.
Summary of Key Points
This article outlined several core aspects of copper's lattice structure:
- Atomic Arrangement: The systematic organization of atoms within copper significantly influences its physical properties.
- Lattice Parameters: Understanding lattice dimensions is essential for predicting how copper behaves under different conditions.
- Conductivity: Both electrical and thermal conductivities derive from the lattice structure, which contribute to the versatility of copper in applications.
- Copper Alloys: Various alloys, formed through interatomic interactions, enhance or modify specific characteristics for targeted uses.
By thoroughly reviewing these points, readers gain insights into the complexities and multifaceted nature of copper.
Implications for Future Research
Continuing research into the copper lattice structure holds promise for numerous advancements. Some of these include:
- Nanostructuring Techniques: Innovative methods to manipulate copper at the nanoscale may reveal new properties, leading to applications in nanotechnology.
- Potential New Alloys: Developing new alloy combinations can optimize attributes like strength and corrosion resistance, expanding copper’s usability.
- Advanced Characterization Techniques: Employing sophisticated techniques, such as synchrotron radiation, can provide deeper insights into atomic arrangements and lattice dynamics, fostering better materials.
Future studies should aim to not only build on existing knowledge but also explore unexplored aspects of copper, encouraging a deeper understanding of its complex lattice structure and enhancing its practical applications.
"The beauty of scientific inquiry lies in the continuous quest for deeper understanding and innovation."
"The beauty of scientific inquiry lies in the continuous quest for deeper understanding and innovation."
This concluding section emphasizes the inherent value of research into the copper lattice structure while inviting ongoing curiosity and scholarship in this fundamental area of study.