Understanding EEG: Key Principles and Applications
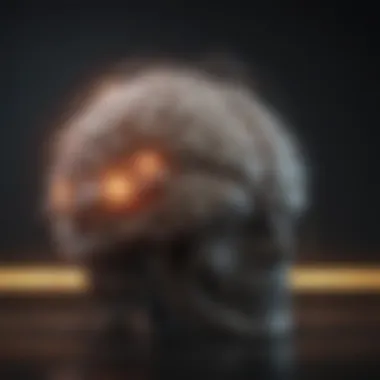
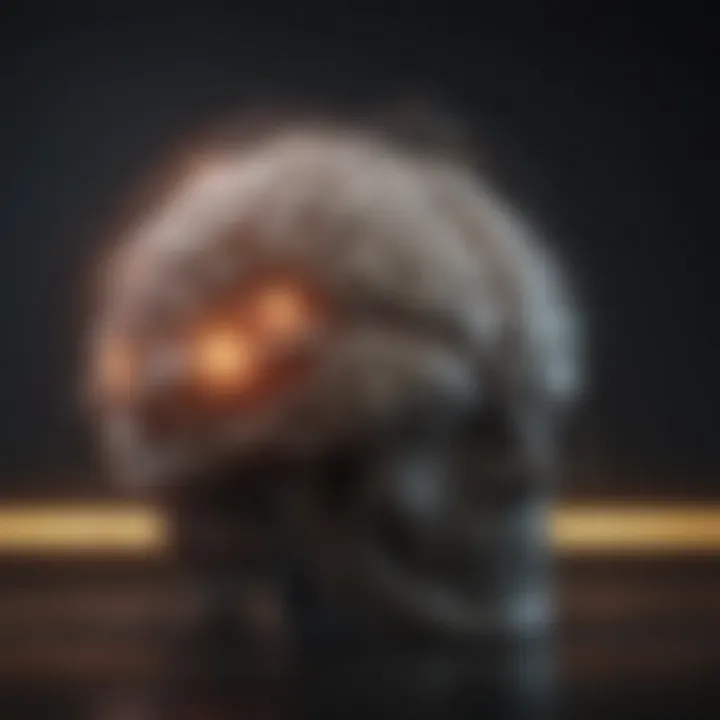
Intro
Electroencephalography, or EEG for short, has become a pivotal method for studying the brain's electrical activity. This field not only uncovers the dynamics of how our brain operates but also dives into various applications, from clinical diagnostics to cutting-edge research ventures. In understanding EEG, one probes beyond mere signal capture; it also involves an exploration of neurological functions and innovative technologies like brain-computer interfaces.
In this discussion, we will break down key concepts and current research trends, as well as examine EEGās vast implications in both health and technology. Each step through this article aims to present intricate details that cater to students, researchers, educators, and professionals, enriching their grasp of this fascinating subject.
Key Concepts
Definition of the Main Idea
EEG refers to the non-invasive monitoring technique that records electrical impulses in the brain. By placing electrodes on the scalp, EEG measures voltage fluctuations resulting from ionic current within neurons. This technique shines a light on how various brain regions communicate, revealing patterns that correspond to cognitive processes such as attention, memory, and sleep.
Overview of Scientific Principles
EEG works on the principle that neurons generate electrical signals during synaptic transmission. These signals create detectable waves that can vary in frequency and amplitude. EEG categorizes these waves into several bands:
- Delta (0.5-4 Hz): Primarily associated with deep sleep
- Theta (4-8 Hz): Linked to lighter stages of sleep and relaxation
- Alpha (8-12 Hz): Occurs during calm, alert states and is dominant when one is awake and relaxed
- Beta (12-30 Hz): Corresponds to active thinking, problem-solving, or nervous energy
- Gamma (30 Hz and above): Associated with peak focus and cognitive function
Each type of wave plays a distinct role in understanding the mental state of individuals, making EEG an invaluable tool in both clinical and research contexts.
Current Research Trends
Recent Studies and Findings
Recent advancements in EEG technology have allowed for more sophisticated analysis of brain activity. Techniques such as high-density EEG and machine learning algorithms are improving the precision in identifying specific brain disorders. For instance, studies have shown that patterns of EEG activity can correlate with conditions like epilepsy and schizophrenia, leading to more tailored therapeutic approaches.
Significant Breakthroughs in the Field
Research is also bridging EEG with other technologies to enhance its applicability. Combining EEG with functional MRI (fMRI) has emerged as a promising path for deeper insights into brain functions. Furthermore, developments in brain-computer interfaces, utilizing EEG signals, are paving the way for new treatments in neuroprosthetics and augmented reality applications.
"EEG is a window into the brain's electrical activity, allowing us to interpret the otherwise invisible processes behind thought and behavior."
"EEG is a window into the brain's electrical activity, allowing us to interpret the otherwise invisible processes behind thought and behavior."
These advancements are indicative of a rapidly evolving field that not only seeks to decode brain functionalities but also strives to apply these insights in transformative ways across various sectors, thereby enriching our overall understanding of cognitive processes.
Intro to EEG
Electroencephalography, commonly known as EEG, has carved its place as an indispensable tool in both medical and research landscapes. This introduction to EEG sets the stage for a larger discourse on its implications and applications. EEG primarily involves capturing the electrical activity in the brain through strategically placed electrodes. This understanding is not merely academic; rather, it serves as a gateway to insights into brain health, cognitive functions, and even mental disorders.
Understanding EEG is paramount to grasping how neurological signals can reflect a personās mental state. For students, researchers, and professionals alike, familiarizing oneself with EEG opens up avenues for exploring brain-computer interfaces and novel technological applications that may reshape our interaction with machines. Itās not just about technology; the principles behind EEG can lead to better patient care and revolutionary methodologies in understanding the human brain.
What is EEG?
At its core, EEG is a scientific technique employed to monitor and record the brain's electrical activities. By placing small metal discs, known as electrodes, on the scalp, EEG measures voltage fluctuations that arise as neurons communicate. This method provides a non-invasive way to observe brain function in real time.
EEG can aid in diagnosing numerous conditions, including epilepsy, sleep disorders, and the effects of traumatic brain injuries. The ability to visualize these brain waves enables healthcare professionals to develop targeted treatment plans. Plus, with advancements in technology, carrying out an EEG has become simpler and more efficient, making it ever more accessible for clinical use.
Historical Context
The journey of EEG began in the 1920s, thanks largely to Hans Berger, a German psychiatrist. He was the first to record brain activity through a method he called electroencephalography. During that time, some met his findings with skepticism; however, as the benefits became more evident, EEG gained prominence in both clinical and research contexts.
Berger's initial experiments laid the groundwork for successive innovations in the field. Through the decades, EEG evolved from mere observation to becoming a staple in diagnosing neurological disorders. Fast forward to today, this technique is crucial in both adult and pediatric medicine, proving its significance time and time again in both diagnosis and ongoing research ventures.
Basic Principles of EEG
To grasp the nuances of EEG, itās essential to understand its fundamental principles. EEG capitalizes on electrodes to detect brain activity, which reflects in wave patterns ā each associated with different states of consciousness and mental activities.
- Electrode Functionality: Electrodes are placed on the scalp to detect electrical impulses. These electrodes are generally arranged in a specific grid pattern to maximize coverage of the brain's surface.
- Wave Patterns: EEG results display a variety of waveforms that are categorized based on frequency:
- Delta Waves (0.5-4 Hz): Common in deep sleep, associated with restorative processes.
- Theta Waves (4-8 Hz): Linked to light sleep, relaxation, and creativity.
- Alpha Waves (8-12 Hz): Manifesting when individuals are awake but relaxed.
- Beta Waves (12-30 Hz): Present during active thinking and problem-solving.
- Gamma Waves (30 Hz+): Linked with high-level cognitive functioning and information processing.
Understanding these basic principles provides a framework for integrating EEG into practical applications, from clinical diagnostics to innovative research in cognitive neuroscience. It is within these fundamentals that the vast potential and implications of EEG can be appreciated.
Mechanisms of EEG Functioning
The mechanisms behind EEG are fundamental to understanding how this technology works and why it is such a valuable tool in both clinical and research settings. EEG provides detailed insights into brain activity by recording electrical signals produced by neurons. This section will elucidate key components including electrode configuration, various waveforms associated with brain activity, and signal acquisition techniques.
Electrode Configuration
The configuration of electrodes in EEG plays a pivotal role in capturing the brain's electrical activity. Electrodes are typically arranged on the scalp according to the International 10ā20 System, ensuring standardized placement for consistent data collection. More precisely, a mix of silver/silver chloride or gold electrodes, well-known for their conductivity, is used to achieve optimal results.
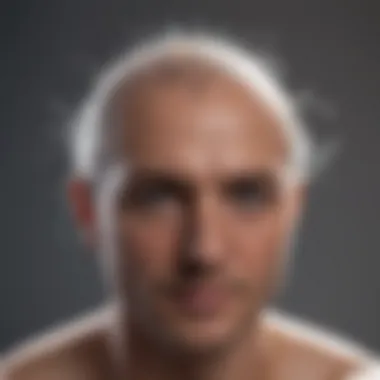
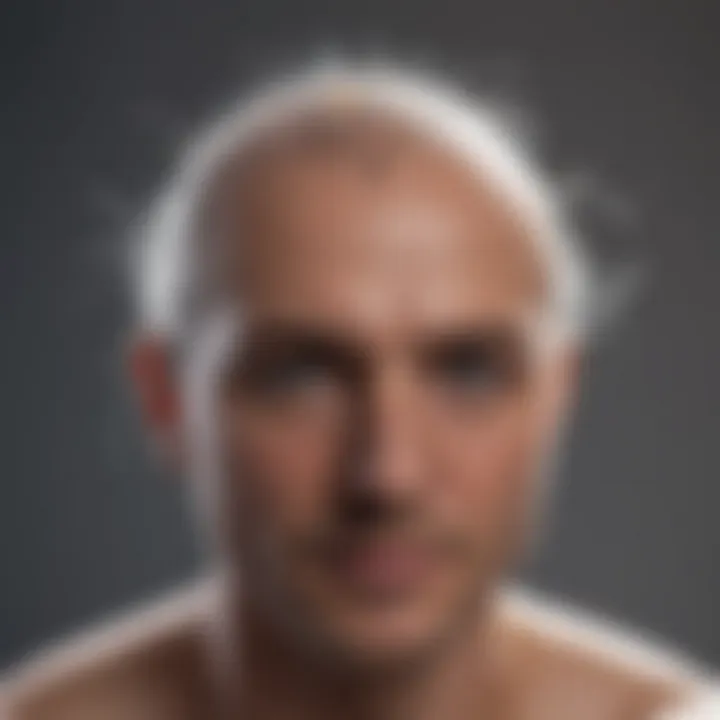
One important aspect is the distinction between monopolar and bipolar configurations. The monopolar setup involves measuring voltage differences between a reference electrode and multiple active electrodes, allowing for a broader perspective of electrical brain activity. On the other hand, the bipolar configuration measures the potential difference between two electrodes, offering a more localized analysis. Each configuration has its benefits; monopolar setups can capture more extensive brain activity, while bipolar configurations excel in pinpointing specific signal changes.
Choosing the right configuration largely hinges on the specific objectives of the EEG study. In clinical settings, for example, epilepsy monitoring often leans toward bipolar configurations to detect localized activity that may indicate seizure foci.
Waveforms of Brain Activity
Brain activity can be categorized into various waveforms, each signifying different states of consciousness or cognitive functions. Understanding these waveforms is essential for interpreting EEG data effectively.
Delta Waves
Delta waves, which oscillate at a frequency of 0.5 to 4 Hz, are the slowest brain waves recorded through EEG. They are predominantly associated with deep sleep stages. A defining characteristic of delta waves is their high amplitude, which suggests a state of restorative rest. In clinical practice, delta waves are beneficial as they can indicate brain recovery during sleep disorders and anesthesia. However, an excessive presence of delta waves in awake patients might indicate abnormal brain function, such as in cases of traumatic brain injury.
Theta Waves
Theta waves range from 4 to 8 Hz and are often linked to states of drowsiness or light sleep. They play a significant role in processes such as creativity, intuition, and daydreaming. Theta waves are particularly interesting because they have been associated with meditation and stress relief, making them relevant in both therapeutic and research contexts. One unique feature of theta waves is their capability to emerge during certain cognitive tasks, suggesting active problem-solving or memory retrieval. However, their prominence during wakefulness might also hint at an emotional imbalance or attention deficit issues.
Alpha Waves
Alpha waves vibrate at frequencies between 8 to 12 Hz and typically accompany a relaxed yet alert mental state, like during meditation or quietly resting with closed eyes. A key characteristic of alpha waves is their capacity to reduce during focused mental efforts, which underscores their usefulness in cognitive performance monitoring. In educational and cognitive studies, alpha waves are often analyzed to evaluate attention levels and learning states.
Beta Waves
In contrast to the slower waveforms, beta waves range from 12 to 30 Hz and indicate active thinking, problem-solving, and active engagement with the environment. They can be particularly prominent when we are anxious or stressed, which makes them a double-edged sword in psychological assessments. The high frequency of beta waves can signify alertness and focused activity, but excessive amounts may also point toward stress or anxiety disorders, which is particularly relevant in clinical psychology.
Gamma Waves
Gamma waves operate at frequencies above 30 Hz and represent the brain's highest level of information processing. They are linked with cognitive functions such as memory, perception, and consciousness. One intriguing aspect of gamma waves is their potential role in frequency synchronization, which is thought to underlie interconnected brain activity during tasks requiring high mental engagement. However, it's worth noting that high levels of gamma waves are sometimes observed in people with certain neurological disorders, making it a crucial area for further research.
Signal Acquisition Techniques
EEG signal acquisition techniques have evolved over the years, allowing for better data quality and lower noise interference. The use of dry electrodes, for instance, has gained traction recently, reducing the need for conductive gel while speeding up the setup process. Moreover, advanced technology like cap-mounted electrodes improves the user's comfort and accuracy, facilitating longer study durations without causing irritation.
Understanding these methods helps in selecting the most effective techniques for specific research or clinical applications, leading to better outcomes in data interpretation and subsequent analyses.
"Effective EEG analysis reaps insights not only from raw signals but from understanding the contexts in which they were gathered."
"Effective EEG analysis reaps insights not only from raw signals but from understanding the contexts in which they were gathered."
Through examining mechanisms of EEG functioning, we uncover its extraordinary capacity to reflect different cognitive states and how various parameters can impact the quality of results. Isolating these mechanisms enhances the precision of diagnoses and furthers our grasp on both healthy and pathological brain functions.
Clinical Applications of EEG
The landscape of clinical EEG applications stretches far and wide, cutting through various medical specialties. Understanding these applications is pivotal, as they not only inform practitioners about brain activity but also guide treatments and interventions. EEG is a non-invasive technique, making it a valuable diagnostic tool in numerous clinical settings. The ease of use and immediate feedback it provides is advantageous for both patients and healthcare providers.
Epilepsy Monitoring
Epilepsy monitoring is perhaps the most well-known clinical application of EEG. For a patient, undergoing an EEG can feel daunting; however, itās essential for diagnosing and managing epilepsy effectively.
The key here is the detection of seizure activity. Every type of seizure creates a unique electrical pattern in the brain. By placing electrodes on the scalp, doctors can observe these patterns in real-time, streaming vital data that can lead to accurate diagnoses. This is crucial because not all episodes of altered consciousness stem from epilepsy; some may be psychiatric or metabolic in nature.
"Up to 30% of patients with epilepsy may be misdiagnosed without proper EEG analysis."
ā Neurology Today, 2022
"Up to 30% of patients with epilepsy may be misdiagnosed without proper EEG analysis."
ā Neurology Today, 2022
Moreover, video EEG monitoring allows for a simultaneous visual assessment during a seizure, aiding in the accurate classification of seizures. This combined approach not only helps in developing an appropriate treatment plan but also improves educational strategies for patients and their families about what to expect. Thanks to advancements in technology, long-term monitoring can even be conducted at home, offering convenience without compromising the quality of care.
Sleep Studies
Another significant application of EEG lies in sleep studies. Sleep disorders have become increasingly prevalent, often leading to diminished quality of life. EEG plays a central role in sleep analysis, enabling researchers and clinicians to delve into the complex architecture of sleep stages.
During a polysomnography study, the EEG captures brain wave activity across the night, revealing the duration and quality of REM and non-REM sleep. This is critical because disruptions in sleep cycles can contribute to numerous health issues, including obesity, cardiovascular disease, and mental health disorders. The data gleaned from sleeping EEG is invaluable for diagnosing conditions like sleep apnea, narcolepsy, and insomnia.
A detailed EEG analysis might not only inform you about whether you're hitting those deep sleep stages but can also cue into specific disturbances that are affecting sleep quality, thus tailoring interventions effectively.
Neurodegenerative Disease Assessment
EEG is not just about epilepsy and sleep; its utility stretches into the realms of neurodegenerative disease assessment as well. Conditions like Alzheimerās disease and Parkinsonās disease can yield distinct electrical activity patterns detectable by EEG. For instance, Alzheimerās patients often show decreased alpha wave activity and increased theta wave presence, which can serve as early indications of cognitive decline.
Routine EEGs in this context can assist in monitoring the progression of the disease and the efficacy of treatment strategies. Furthermore, EEG findings can often correlate with cognitive performance in these patients, thus offering insights into the effectiveness of therapeutic interventions.
As the field of neuroscience advances, integrating EEG with other diagnostic modalities can refine our understanding and management of cognitive impairments. For instance, combining EEG data with MRI scans could offer a composite view of brain structure and function, paving the way for more targeted treatments.
In summary, EEG's diverse clinical applications provide a rich tapestry of insights into brain function, guiding both diagnosis and treatment across various neurological conditions. Its versatility as a diagnostic tool underscores the crucial role that EEG plays in contemporary clinical practice.
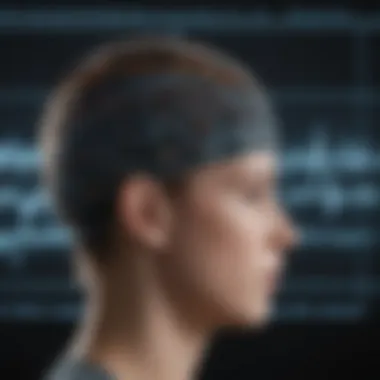
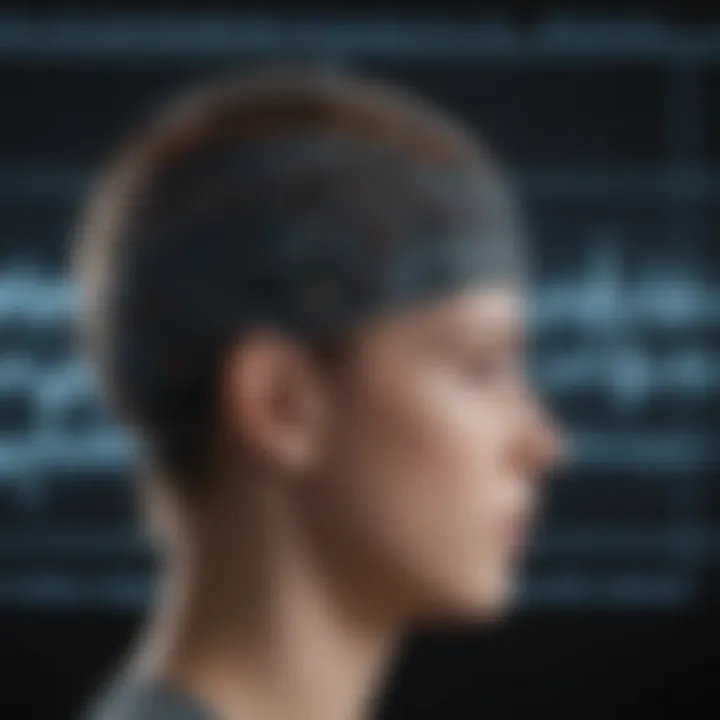
Research Applications in Neuroscience
The exploration of electroencephalography (EEG) meshes tightly with the investigations in neuroscience. EEG serves as a bridge to understanding how the brain functionsānot just in healthy states, but also when faced with challenges. Researchers utilize it to unravel complex neural processes that govern cognition, emotion, and interaction with technology, facilitating multiple benefits and considerations in various scientific arenas.
Cognitive Function Studies
Cognitive function studies often hinge on understanding the dynamics of attention, memory, and decision-making within the brain. Researchers employ EEG to gather real-time data concerning brain activity while individuals engage in cognitive tasks.
- Temporal Resolution: One of the standout features of EEG is its exceptional temporal resolution. This allows scientists to observe brain responses almost instantaneously as subjects react to stimuli, providing insights into how cognitive processes unfold over milliseconds.
- Event-Related Potentials: One commonly measured aspect in these studies is the event-related potential (ERP), which refers to measured brain responses that are the direct result of a specific sensory, cognitive, or motor event. This offers a rich understanding of the timing and nature of cognitive processes.
- Algorithmic Analysis: Advanced analytics and machine learning algorithms are increasingly being applied to EEG data. These innovations allow researchers to decode cognitive states, potentially leading to better brain-computer interaction designs.
These elements emphasize that EEG is more than a diagnostic tool; it's a window into the cognitive machinery and a means to enhance learning and memory techniques based on neurophysiological insights.
Emotional Processing
The intricate relationship between brain activity and emotions is another focal point of EEG research. By monitoring brain waves during emotional stimuli, scientists gain insights into how various moods and feelings manifest neurologically.
- High Temporal Sensitivity: EEG captures changes in emotional states quickly, which is essential in understanding the fleeting nature of emotions. For instance, studies have shown variations in alpha and theta wave patterns during emotional arousal.
- Diagnostics: Understanding emotional processing through EEG has clinical implications, particularly for conditions such as depression and anxiety. It helps in determining effective therapeutic approaches, tailoring treatments based on neural activity patterns.
- Psychophysiological Correlates: The overlap between emotional states and neurological activities offers a solid basis for further research into psychophysiological correlates, advancing both neuroscience and psychology.
Insights gained here shed light on the underlying mechanisms responsible for emotional responses, ultimately contributing to better mental health outcomes.
Brain-Computer Interfaces
The fusion of EEG and brain-computer interfaces (BCIs) marks a groundbreaking area of research. BCIs, which enable direct communication between the brain and external devices, rely heavily on real-time EEG data.
- Control and Communication: EEG can capture brain patterns that correspond to imagined movements or thoughts, enabling users to control devices simply with their minds. Imagine someone unable to move finding freedom through technology that interprets their neural signals into actions.
- Innovations in Accessibility: This technology opens doors for individuals with severe disabilities, allowing them to communicate and interact with their environments more effectively.
- Neurofeedback Applications: Additionally, BCIs leveraging EEG are being explored in therapeutic settings, where neurofeedback is employed to help users regulate their brain activity.
As these applications develop, understanding EEGās role in neural communication becomes vital, bringing together neurotechnology and therapeutic outcomes.
EEG stands at the crossroad of neuroscience and technology, enhancing our understanding and interaction with the brain.
EEG stands at the crossroad of neuroscience and technology, enhancing our understanding and interaction with the brain.
Understanding EEG Data and Interpretation
Understanding EEG data and its interpretation is crucial for anyone delving into the field of electroencephalography. The insights derived from EEG not only help in diagnosing various neurological disorders but also expand our knowledge of brain functionality. Without mastering the interpretation of EEG data, one might find it nearly impossible to see the full picture of neural activity. This section will cover the key elements that guide the extraction and interpretation of EEG data, alongside the benefits that come with this understanding.
Data Collection Techniques
Data collection in EEG is primarily about gathering electrical signals via electrodes placed on the scalp. These electrodes serve as conduits, capturing the brain's electrical activity and sending it to a computer for analysis. The configurations can be as diverse as a 10-20 system, which is a standardized method for placing electrodes based on specific landmarks on the skull. Sometimes, researchers may opt for higher density montages, which deploy larger numbers of electrodes; this provides finer spatial resolution. However, collecting high-quality EEG data is not merely about counting electrodes; it's also about the methods used to ensure the signals are captured accurately.
- Using a high-quality amplifier is essential for amplifying the tiny electrical signals produced by the brain.
- Signal sampling rates must be appropriately set to prevent losing critical information.
- Environmental factors can also affect data collection; hence, eliminating or controlling for noiseālike electrical equipment or even mobile phonesāis a key consideration.
Artifact Reduction Strategies
Artifacts are, without a doubt, one of the most frustrating challenges in EEG interpretation. They can arise from various sources, including muscle movements, eye blinks, and even external electrical activity. Implementing effective artifact reduction strategies not only improves overall data quality but also increases the accuracy of interpretations.
One commonly used method involves signal filtering, allowing researchers to isolate brain waves from noise. Another effective strategy is utilizing tools like Independent Component Analysis (ICA). This technique helps in identifying and removing specific artifact patterns without compromising the integrity of the brain signals. Finally, educating participants about minimizing head movements during recordings can also be a straightforward approach to reduce artifacts.
Analytical Methods
Analyzing EEG data involves extracting meaningful information from the collected signals. Two primary analytical methods stand out: Frequency Analysis and Time-Domain Analysis.
Frequency Analysis
Frequency Analysis focuses on examining the different frequencies present in the EEG signal. This method allows researchers to identify distinct brain wave patterns, such as alpha or beta waves, that correlate with various cognitive states. One key characteristic of Frequency Analysis is its ability to transform time-domain data into the frequency domain through techniques like the Fast Fourier Transform (FFT). This transformation is particularly advantageous because it reveals how much of the signal's power lies within specific frequency bands.
However, while Frequency Analysis is highly beneficial in identifying oscillatory patterns, it may come with its own drawbacks. For instance, it lacks temporal resolution, meaning it's not as effective for understanding brief changes in neural activity. Still, its utility in exploring sustained brain activity cannot be understated.
Time-Domain Analysis
On the other hand, Time-Domain Analysis offers a different lens through which to interpret EEG data. This technique involves analyzing the signals as they change over time, which can provide insight into the dynamics of brain processes. The key characteristic here is the ability to assess how quickly events unfold, making it a popular choice for certain types of cognitive research.
A unique feature of Time-Domain Analysis is its capability to capture event-related potentials (ERPs), which are brain responses elicited by specific stimuli. This can be exceptionally advantageous when investigating how the brain responds to external stimuli in real time. However, while Time-Domain Analysis is potent, the downside is that it might not sufficiently highlight the underlying frequencies present in the data.
"Understanding the intricacies of both analytical methods is paramount for researchers wanting to glean every bit of insight from EEG data. They are complementary in their approach, offering diverse perspectives on brain activity."
"Understanding the intricacies of both analytical methods is paramount for researchers wanting to glean every bit of insight from EEG data. They are complementary in their approach, offering diverse perspectives on brain activity."
In summary, understanding EEG data collection and interpretation is multifaceted and requires careful attention to detail. By utilizing proper data gathering techniques, applying effective artifact reduction strategies, and mastering analytical methods, researchers can extract invaluable insights to advance the understanding of brain function.
Challenges and Limitations of EEG
Spatial Resolution Limitations
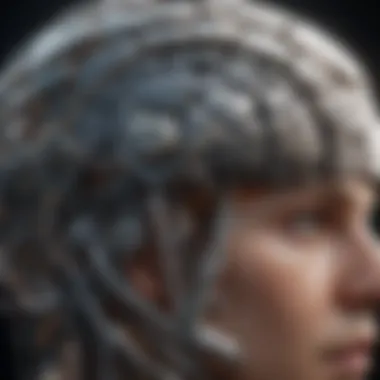
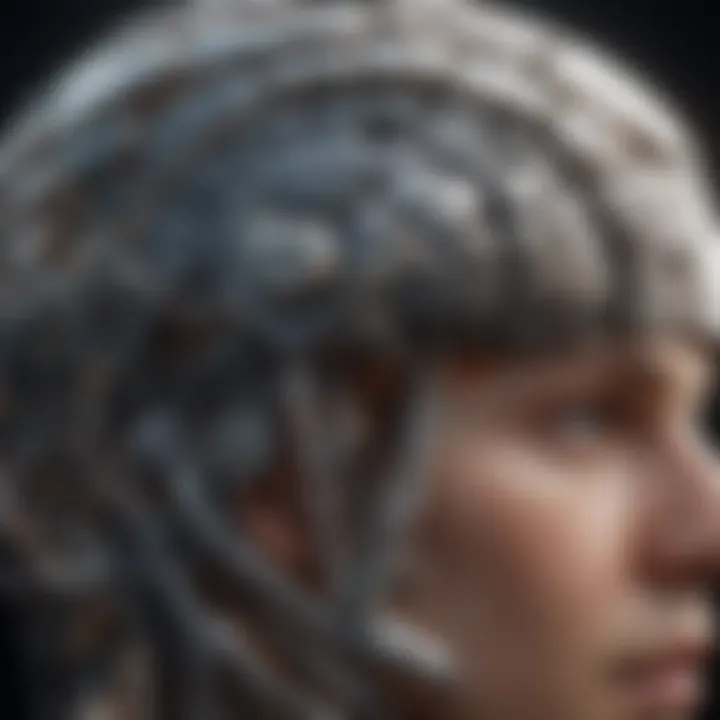
When it comes to measuring brain activity, EEG faces a significant hurdleāits spatial resolution. This refers to the ability to pinpoint the exact location in the brain that is producing electrical signals. Though EEG is excellent for capturing the timing and pattern of brain waves, it often struggles to provide precise information about where those signals are coming from. The electrodes, usually placed on the scalp, can only give a general idea of the brain's activity and not a pinpoint location. Thus, multiple areas of the brain may be influenced by the same electrical activity, creating a muddled interpretation.
The underlying geometric arrangement of the electrodes and the nature of how electrical signals propagate through the skull add to the challenge. With the headās complex structure, signals can dissipate or be obscured as they travel through layers of tissue and bone, making it difficult for researchers and clinicians to accurately determine the source of electrical activity. This limitation often leads to an awkward game of connect-the-dots, where the dots are far apart, making the picture less clear. To make matters more complex, not all brain activities are detectable through scalp EEG, as some deep-seated electrical activities escape detection entirely.
To elaborate on this further:
- EEGās resolution is generally around 1 to 2 centimeters. In contexts where precise localization is necessaryālike during surgical planningāthis deficiency can be problematic.
- Compared to imaging modalities like fMRI or PET, EEG lags behind, as those techniques can provide images with millimeter-level precision.
Despite these spatial limitations, EEG remains a valuable tool, particularly for understanding dynamic brain processes such as events tied to cognition and seizure activity.
Signal Noise Issues
Another thorn in the side of EEG is the prevalence of signal noise, which complicates the interpretation of brain data. Essentially, this refers to the unwanted electrical signals and artifacts that can muddle the valuable data being collected. This noise can arise from various sources, including electrical interference from nearby devices, muscle activity (like jaw clenching or eye blinking), and even external factors such as the room temperature or ambient electrical fields.
Artifacts can draw attention away from genuine brain activity and can appear in the data as erratic spikes or fluctuations that lead one down the wrong diagnostic path. Understanding how to filter out this noise is an ongoing challenge. In an ideal scenario, differentiating between the brainās electrical signals and the noise that accompanies them is crucial for accurate interpretation. A poor signal-to-noise ratio can lead to misdiagnosis or an incomplete understanding of a subjectās condition.
Hereās a breakdown of common sources of noise:
- Physiological Artifacts: Movements such as blinking or tapping can introduce noise significant enough to obscure critical brain signals.
- Environmental Noise: Electromagnetic fields from other electronic devices can interfere, overshadowing the delicate signals emanating from the brain.
- Improper Equipment Setup: If electrodes are not correctly placed or if there are issues with the equipment, the quality of the data can suffer.
To tackle these signal noise issues, researchers and practitioners employ various artifact reduction strategies, such as preprocessing and filtering techniques, but these solutions are not foolproof.
A careful balance must be struck; while it is critical to minimize noise, over-filtering can result in the loss of valid signals, leading to skewed analysis.
A careful balance must be struck; while it is critical to minimize noise, over-filtering can result in the loss of valid signals, leading to skewed analysis.
In summary, while EEG technology has its strengths and has made revolutionary strides in neuroscience, spatial resolution and signal noise are challenges that researchers must continually navigate. Achieving the best results involves an ongoing quest for better techniques to enhance signal clarity while acknowledging the inherent limitations.
Future Perspectives of EEG Technology
As we look towards the horizon of neurotechnology, the future perspectives of EEG technology indicate a dynamic and promising journey ahead. The relevance of this topic cannot be overstated, as advancements in EEG hold the power to revolutionize our understanding of brain activity and its applications in both clinical and research settings.
By enhancing the fidelity of EEG readings and integrating various modalities, professionals can glean deeper insights into neurological conditions, paving the way for innovative treatments and therapeutic strategies. As society strides towards a more technologically integrated future, EEG technology, alongside its innovative applicationsāincluding brain-computer interfacesācould redefine how we interact with our own cognitive processes.
Innovative Technologies in EEG
Innovative technologies in EEG are emerging at a rapid pace. For instance, the development of higher density electrode arrays is one such advancement that allows for a more comprehensive mapping of brain activity. These tightly packed electrodes capture more detailed information, enabling researchers and clinicians to pinpoint precise regions of activation.
Additionally, wireless EEG systems are becoming increasingly popular, enhancing mobility and ease of use in both laboratory and everyday settings. This flexibility opens up avenues for continuous monitoring and perhaps even remote diagnostics. Notable advances also include the incorporation of machine learning algorithms, which enable real-time analysis of EEG data. These algorithms can detect subtle changes in brain wave patterns, providing valuable insights into conditions such as epilepsy or sleep disorders.
Another remarkable development is the integration of EEG with wearable technology. Devices embedded with EEG sensors could offer users personalized feedback on their mental state, fostering self-awareness and improving mental health management. All these innovations not only improve accuracy but also broaden the application of EEG in diverse fields such as consumer technology, mental health, and even entertainment.
Integration with Neuroimaging Techniques
The blending of EEG with neuroimaging techniques, such as functional Magnetic Resonance Imaging (fMRI) or Positron Emission Tomography (PET), represents a significant leap forward. Each method has its strengths: while fMRI provides excellent spatial resolution, EEG excels in capturing rapid changes in brain activity. By combining these modalities, researchers can achieve a more holistic view of brain function.
This synergy enhances the interpretation of brain data, allowing for a clearer understanding of the dynamics between brain regions during various tasks. For example, during a cognitive task, having simultaneous fMRI and EEG data could reveal not just where the activity occurs, but how different areas are communicating over time. This integrative approach has the potential to advance our comprehension of complex brain disorders, improving diagnostic capabilities and therapeutic outcomes.
Moreover, as technology progresses, the cost of neuroimaging is gradually decreasing, making it more accessible for educational and clinical use. This could encourage further exploration into treatment modalities that harness the strengths of both EEG and neuroimaging, leading to tailored patient care approaches.
As the field continues to evolve, we stand on the brink of a neurometric revolution that could fundamentally alter our understanding of the brain and its myriad functions.
"The future is not something we enter. The future is something we create." - Leonard I. Sweet
"The future is not something we enter. The future is something we create." - Leonard I. Sweet
At the core, the continuous advancements in EEG technology and its integration into a wider framework of neuroimaging depict a frontier of possibilities that will likely transform how we perceive and treat neurological disorders.
End and Implications
In reflecting on the intricate tapestry that is electroencephalography (EEG), one takes a step back to appreciate its profound significance across various domains. EEG is not merely a method of tracing the brainās electrical signals; it serves as a window into the dynamic interplay of neural activity that accompanies every thought, emotion, and action we experience. Understanding EEG means grasping how our brains communicate and operate, paving the way for nuanced insights into both the physical and psychological realms of health.
Summary of Key Insights
EEG has proven invaluable in multiple fields, particularly in clinical and research settings. Some key takeaways include:
- Clinical Utility: The ability to monitor and evaluate conditions like epilepsy or sleep disorders highlights EEGās role in diagnostics. This diagnostic tool is essential for determining treatment strategies and measuring efficacy.
- Research Applications: EEG offers researchers a non-invasive means to study cognitive processes, providing valuable data on attention, perception, and even creativity. Its further integration with technologies like machine learning could enhance data interpretation capabilities.
- Technological Innovation: As EEG technology evolves, innovations such as wearable EEG devices are opening doors for real-time monitoring in daily life. This could shift how we approach mental health care, making it more personalized.
"The cost of ignorance is always higher than the price of knowledge."
"The cost of ignorance is always higher than the price of knowledge."
With the advancements in EEG technology come ethical considerations that require careful navigation to ensure it does not infringe upon individual rights or exacerbate existing disparities in healthcare access.
The Ethical Considerations
As with any technology that probes into the inner workings of the human brain, EEG brings forth ethical implications that warrant serious contemplation:
- Privacy Concerns: The collection and interpretation of brain data pose risks regarding personal privacy. Who owns this data? How can we ensure it is safeguarded against misuse?
- Informed Consent: Navigating the ethical terrain demands transparency. Patients must be educated on what EEG entails, its potential implications, and any risks involved.
- Access and Representation: The benefits of EEG research and applications should extend equitably across societal strata. Disparities in access could lead to biased results, hindering the validity of findings.