Understanding Electric Field Sensors: Principles and Applications
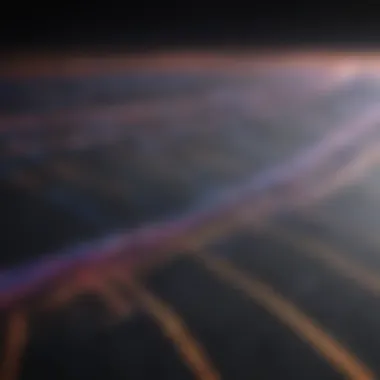
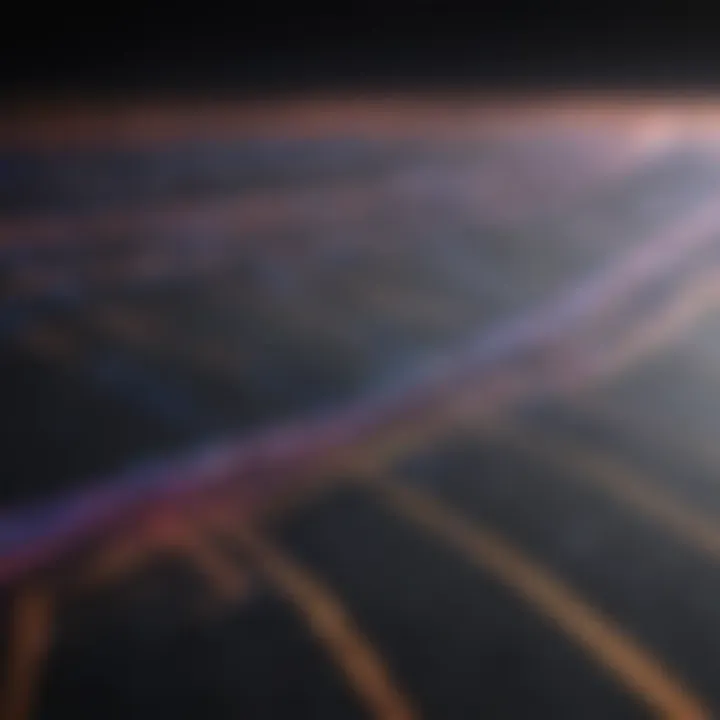
Intro
The exploration of electric field sensors opens a door to understanding various applications across scientific and industrial realms. These sensors, integral in measuring electric fields caused by charged particles, are pivotal in multiple disciplines, including environmental monitoring and laboratory research. The functionality of these devices lies in their ability to detect variations in electric currents, making them essential tools for many professionals.
The significance of electric field sensors cannot be overstated. In areas such as meteorology and medical diagnostics, they help in understanding atmospheric phenomena and analyzing biological processes. Moreover, ongoing technological advancements are continually enhancing their capabilities. This article seeks to unravel the basics, recent developments, and practical uses of electric field sensors.
Key Concepts
Definition of the Main Idea
Electric field sensors are devices designed to quantify electric fields produced by charged objects or changing electrical currents. They work based on principles of electromagnetism, where varying electric fields can indicate the presence and characteristics of charges around them. The simplest form is the capacitive sensor, which measures field strength based on capacitance changes when nearby charges are introduced.
Overview of Scientific Principles
The operating principles of electric field sensors can be categorized into various types, including:
- Capacitance-based sensors: Measure changes in capacitance that occurs due to proximity of charged objects.
- Field-effect sensors: Utilize changes in electrical current to detect variations in field strength.
- Optical techniques: Some advanced sensors employ optical properties for non-contact measurements of electric fields.
Understanding these principles is critical for appreciating how electric field sensors operate and their application across numerous fields.
Current Research Trends
Recent Studies and Findings
Recently, there has been a notable shift towards miniaturization and integration of electric field sensors with microelectronic systems. Research studies highlight the development of programmable sensors designed for real-time data acquisition within urban environments. This flexibility allows for detailed monitoring of electric fields in contexts like power lines or electronic devices.
Significant Breakthroughs in the Field
Significant breakthroughs include the use of nanostructured materials that enhance the sensitivity and response time of sensors. These materials can detect much weaker field strengths compared to traditional sensors. Recent findings suggest that combining electric field sensors with machine learning algorithms can provide better analytical capabilities, offering predictive measures for system behavior.
"Advancements in sensor technology are revolutionizing how we measure electric fields, pushing boundaries of precision and application."
"Advancements in sensor technology are revolutionizing how we measure electric fields, pushing boundaries of precision and application."
The convergence of these trends signals a future where electric field sensors play an even larger role in scientific inquiry and industrial processes.
Foreword to Electric Field Sensors
Electric field sensors play a significant role in the realms of physics and engineering. These sensors are fundamental devices that measure electric fields produced by static or dynamic electric charges. Their operation relies on basic physical principles that make these measurements possible. Understanding electric field sensors is crucial for advancements in various fields such as environmental science, industrial automation, and electrical research. In this article, we will cover the essential concepts, advancements, and challenges in this emerging field.
Defining Electric Field Sensors
Electric field sensors are instruments designed to detect and measure electric fields. These fields are created by charged particles or varying electrical currents. The sensors can convert the electric field into a readable form, usually in volts per meter (V/m). The basic functionality rests on principles like capacitance and charge detection. There are different types of electric field sensors, including passive and active varieties, each with its specific application and construction.
Understanding how these devices function is important for effective use in research and applications. For instance, passive sensors rely on naturally occurring charges, while active sensors generate their signals through external power sources. This distinction is crucial for selecting appropriate sensors for specific tasks.
Importance in Scientific Research
In scientific research, electric field sensors hold a critical position. They enable precise measurements needed to understand complex electrical behaviors in various environments. Scientists utilize these sensors for numerous applications, from laboratory experiments to field research.
The use of electric field sensors in research supports several key areas:
- Data Collection: Scientists require reliable data. Electric field sensors provide accurate readings of electrical influences around charged objects.
- Experimental Validation: Researchers can test theories about electric phenomena, validating or challenging existing physical laws.
- Environmental Monitoring: Sensors help monitor electric fields in ecosystems, providing insights into the interaction of organisms with electromagnetic fields.
"Electric field sensors can transform understanding in various scientific disciplines, especially when precision is essential."
"Electric field sensors can transform understanding in various scientific disciplines, especially when precision is essential."
In summary, electric field sensors are indispensable to modern scientific inquiry. Their ability to capture specific data from electric fields supports advancements in science and technology. Understanding their principles and importance sets the stage for deeper exploration in this article.
Fundamental Principles of Electric Fields
The study of electric field sensors cannot be fully understood without discussing the fundamental principles of electric fields. Electric fields serve as the invisible force governing the interaction between charged particles. Their importance extends across various disciplines, making the comprehension of their principles essential for both practical applications and theoretical knowledge. Without a clear understanding of concepts like electric charge and the characteristics of electric fields, one cannot effectively work with electric field sensors.
Understanding Electric Charge
Electric charge is fundamental to the concept of electric fields. It is a property of subatomic particles, primarily electrons and protons, dictating their electromagnetic interactions. There are two types of electric charge: positive, carried by protons, and negative, held by electrons. The interaction of these charges creates electric fields around them.
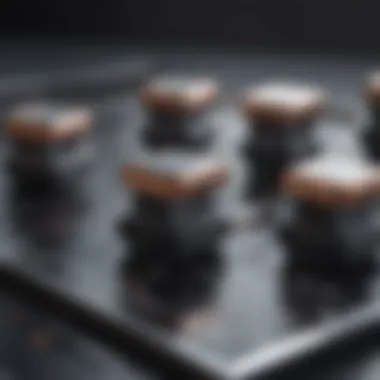
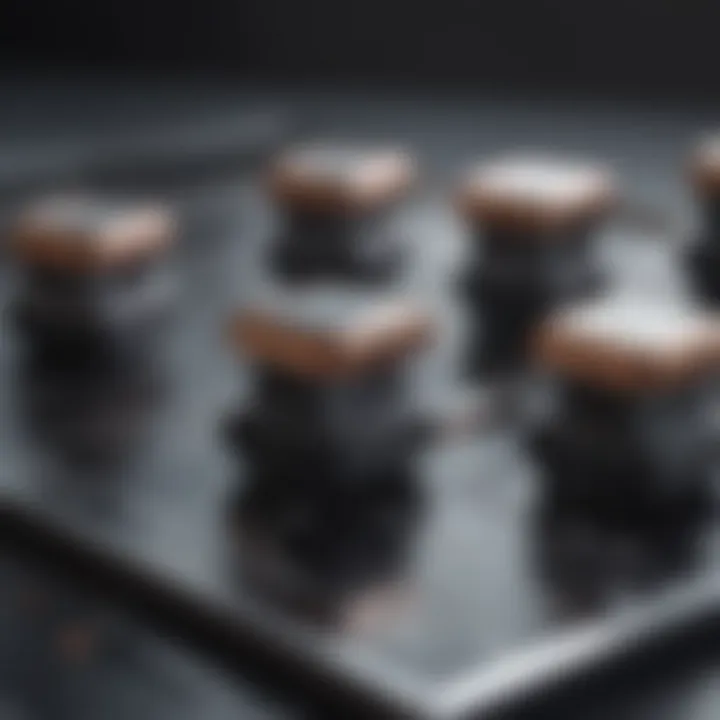
The essential characteristics of charge include:
- Conservation: Charge is neither created nor destroyed. It can only be transferred from one body to another.
- Quantization: Electric charge exists in discrete amounts, the smallest unit being the charge of a single electron or proton.
- Coulomb's Law: This law quantifies the force between two charged objects, which is directly proportional to the product of their charges and inversely proportional to the square of the distance between them.
Understanding these properties is crucial for comprehending how electric field sensors detect changes in electric fields. Manipulating these charges allows engineers and scientists to create sensors that respond efficiently to varying electrical conditions.
Characteristics of Electric Fields
Electric fields arise from the presence of charged particles and exhibit several distinctive characteristics. These fields can be visualized as lines of force emanating from charged bodies. Some key characteristics include:
- Direction: Electric field lines point away from positive charges and towards negative charges, indicating the direction a positive test charge would move in the field.
- Strength: The strength of an electric field is measured in volts per meter (V/m). It diminishes with distance from the source charge.
- Superposition Principle: When multiple charges are present, the total electric field at a point is the vector sum of the individual fields created by each charge. This principle highlights the cumulative effect of electric fields from various sources.
These features are critical in various applications, from electromechanical systems to communication technologies. Knowledge of electric fields informs the design and functionality of electric field sensors, ensuring accurate readings and effective monitoring.
Key Insight: Electric field sensors, by relying on the understanding of electric charge and fields, allow researchers to unlock a myriad of applications in scientific exploration and industrial processes.
Key Insight: Electric field sensors, by relying on the understanding of electric charge and fields, allow researchers to unlock a myriad of applications in scientific exploration and industrial processes.
The exploration of electric charge and electric fields lays a solid foundation for further discussing electric field sensors and their applications in real-world scenarios.
Types of Electric Field Sensors
Electric field sensors are pivotal in numerous applications, serving diverse fields from environmental monitoring to industrial automation. Understanding the types of electric field sensors can help guide the selection process for specific applications. Each type offers distinct advantages and disadvantages depending on the context of use, enabling researchers and practitioners to tailor solutions to their needs.
Passive Sensors
Passive sensors operate without any external power source. Instead, they rely on the electric field itself to function. These sensors detect the changes in electric fields and usually employ capacitive methods for measurement. One fundamental characteristic is that they can be highly sensitive to low electric field strengths. This makes them particularly useful in environments where electromagnetic interference is minimal, such as laboratory settings. However, passive sensors can be less effective in conditions with fluctuating external fields since they lack active compensation mechanisms.
Some common types of passive sensors include:
- Capacitive sensors: They measure variations in capacitance caused by changes in electric field strength.
- Inductive sensors: These operate based on the inductance variation in response to electric fields.
An advantage of using passive sensors is their simplicity and lower cost. Still, their reliance on external fields means that they may not generate readings in every situation. They are often used for static measurements in controlled environments.
Active Sensors
In contrast to passive sensors, active sensors require a power source to operate. They generate their own electric fields, allowing them to provide more dynamic responses to environmental changes. The active nature of these sensors significantly enhances sensitivity and accuracy.
Active sensors typically utilize various detection methods, including capacitive, field-effect, or optical detection, to offer comprehensive data collection. For example, field-effect transistors can be employed in active sensors to detect variations in electrical fields with high fidelity. This capability makes them particularly effective in applications requiring real-time monitoring and response.
Some key benefits of active sensors include:
- Higher sensitivity compared to passive sensors.
- Ability to measure both static and dynamic electric fields.
- Flexibility in application across different environmental conditions.
Nevertheless, active sensors can be more complex and costly due to the additional components required for power supply and signal processing.
Comparative Analysis
When examining passive and active sensors, various aspects emerge that can influence the decision of which type to use.
| Feature | Passive Sensors | Active Sensors | | Power Requirement | No external power needed | Requires external power | | Sensitivity | Generally lower | Generally higher | | Application Areas | Static measurements | Real-time monitoring | | Cost | Generally lower | Generally higher | | Environmental Adaptability | More limited | More versatile |
Understanding these differences guides users in selecting the sensor that fits their specific needs. For example, if cost is a primary concern and measurements are happening in a stable environment, passive sensors might be sufficient. Conversely, in high-demand environments requiring ongoing monitoring, active sensors would be the preferred choice.
In summary, both passive and active electric field sensors serve unique functions and choosing the right one depends on various factors including application requirements, cost, and environmental conditions.
In summary, both passive and active electric field sensors serve unique functions and choosing the right one depends on various factors including application requirements, cost, and environmental conditions.
Operating Mechanisms
Understanding the operating mechanisms of electric field sensors is essential, as these fundamental components play a critical role in how these devices function and deliver accurate measurements. The mechanisms influence the reliability, response time, and sensitivity of sensors. To develop a comprehensive understanding, one must consider how these sensors are constructed and how they detect electric fields.
Construction of Electric Field Sensors
The construction of electric field sensors involves a variety of materials and designs that enhance their performance. The typical design varies depending on the type of sensor, whether passive or active.
For instance, passive sensors usually consist of conductive materials that respond to the presence of electric fields. In contrast, active sensors incorporate amplifying components to enhance their response.
Various elements, such as electrodes and insulating materials, are used in their construction. Materials like polymers, ceramics, and metals can be employed to create electrodes that are sensitive to electric fields. Each choice impacts the sensitivity and efficiency of the device. Furthermore, the arrangement of these components can affect the directionality and range of the sensor's response.
Detection Methods
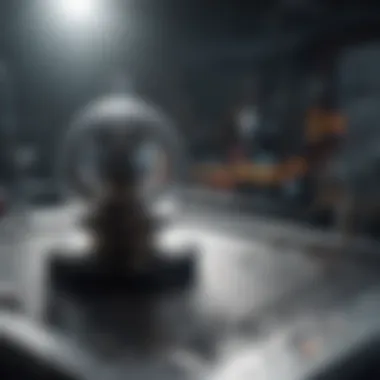
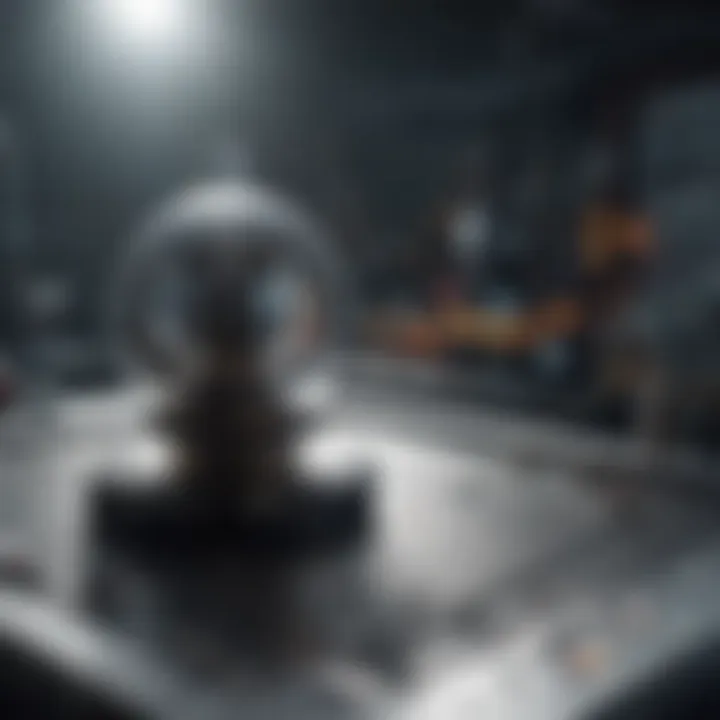
The detection methods utilized by electric field sensors determine how effectively they respond to changes in electric fields. There are three prominent techniques that are widely adopted, each with distinct advantages and applications.
Capacitance-based Detection
Capacitance-based detection relies on the principle of capacitance, where changes in electric fields cause variations in capacitance between two conductive plates. This method is widely recognized for its simplicity and effectiveness in detecting variations in electric fields.
One key characteristic of capacitance-based detection is its ability to provide high sensitivity at close distances. This is particularly beneficial in applications requiring precise measurements over small scales, such as in laboratory experiments.
One unique feature is its adaptability to various environments; it can function well in environments with low noise. However, limitations can arise in high-frequency applications, where response times may be slower due to the inherent characteristics of capacitor charge and discharge cycles.
Field-effect Detection
Field-effect detection uses field-effect transistors to detect electric fields, converting electric field strength into measurable electronic signals. This method offers high sensitivity and fast response time, making it suitable for many applications, including electronic devices and environmental monitoring.
Its key characteristic is the ability to detect minute electric field changes and provide real-time data. Field-effect detection is particularly beneficial for applications focused on detailed analyses of electric field distributions.
A unique aspect is its compact design, allowing for integration into advanced technology systems like smart sensors. On the downside, the performance can be affected by temperature variations, which may lead to false readings if not adequately calibrated.
Optical Detection
Optical detection leverages light's interaction with electric fields to identify field strength. This method typically involves the use of photonic materials that change their optical properties in response to electric fields.
One of the attractive characteristics of optical detection is its immunity to electromagnetic interference, allowing for accurate measurements in noisy environments. This advantage makes it a solid choice for applications such as remote sensing or in situations where electrical noise might be present.
Unique features of optical detection include a wide measurement range and the potential for non-invasive assessments. However, setup complexities and cost factors can make it less favorable for some standard applications compared to more straightforward methods like capacitance-based detection.
The choice of detection method can significantly impact the overall performance and application suitability of electric field sensors, making it a critical consideration in sensor development.
The choice of detection method can significantly impact the overall performance and application suitability of electric field sensors, making it a critical consideration in sensor development.
Common Applications
The common applications of electric field sensors span across various fields such as scientific research, environmental assessment, and industry. Understanding these applications is crucial for grasping the full potential and utility of these sensors. Electric field sensors provide accurate measurements of electric fields, enabling better monitoring and analysis in different scenarios.
Laboratory Research
In laboratory settings, electric field sensors serve pivotal roles in experiments and studies involving electromagnetic phenomena. These sensors are essential for tasks like measuring the strength of electric fields generated by charged particles or assessing electric properties of materials. The precision offered by these sensors aid researchers in conducting reproducible and accurate experiments, ultimately advancing our knowledge in physics and related fields.
Researchers use electric field sensors to validate theoretical models and to test hypotheses. For example, in particle physics or materials science, scientists need reliable data about electric forces that impact their experiments. The sensors can also help in detecting minute variations in electric fields, contributing to deeper insights into material properties or behaviors under different conditions.
Environmental Monitoring
Electric field sensors play a significant role in environmental monitoring. They help in assessing the impact of electric and magnetic fields on ecosystems. For instance, scientists can use them to evaluate how nearby electrical infrastructure affects wildlife and vegetation. This is increasingly important as concerns regarding electromagnetic pollution grow. By monitoring the surrounding electric fields, researchers can gather data to make informed conclusions about safety and impacts.
Moreover, electric field sensors assist in detecting atmospheric electricity phenomena. This includes measuring drift in electric charges during storm events which is crucial for understanding weather patterns and potential storm development. The data collected also feeds into algorithms for forecasting weather, thereby enhancing predictive capabilities.
Industrial Applications
In industrial settings, electric field sensors demonstrate their versatility and importance. Industries often utilize these sensors for monitoring machinery and ensuring operational safety. In manufacturing, for instance, the sensors help in the evaluation of electromechanical systems, identifying malfunctions before they lead to significant failures. This proactive approach to maintenance is invaluable for reducing downtime and increasing productivity.
Additionally, electric field sensors find uses in quality control processes. By ensuring that products meet specific electric property standards, manufacturers can maintain high levels of quality. In sectors such as electronics, automotive, and energy, reliable functioning of equipment significantly relies on the precise measurements provided by these sensors.
Furthermore, as factories integrate more automation and smart technologies, the role of electric field sensors is expected to become even more pronounced. With advancements in IoT, these sensors will likely become integral components in smart factory setups, enhancing operational efficiency and monitoring capabilities.
Electric field sensors are not merely tools; they serve as critical instruments across disciplines, promoting safety, efficiency, and scientific advancement.
Electric field sensors are not merely tools; they serve as critical instruments across disciplines, promoting safety, efficiency, and scientific advancement.
Overall, the significance of electric field sensors in laboratory research, environmental monitoring, and various industrial applications cannot be overstated. These systems not only facilitate enhanced understanding and monitoring but also drive innovation and improvement in respective fields.
Technological Advancements
The field of electric field sensors is continuously evolving as technologies advance. These advancements not only enhance the performance of sensors but also expand their potential applications. Understanding these technological developments is critical for researchers and professionals who seek to leverage electric field sensors effectively. This section delves into three pivotal advancements: miniaturization, integration with IoT, and the use of innovative materials.
Miniaturization of Sensors
Over recent years, sensor miniaturization has significantly influenced the design and usability of electric field sensors. Smaller sensors often realize essential benefits such as
- Increased Portability: Compact designs allow for easier transportation and deployment in diverse environments.
- Enhanced Sensitivity: Miniaturized sensors often exhibit improved sensitivity, enabling them to detect subtle changes in electric fields, which is useful in sensitive laboratory settings.
- Application Variety: Miniature sensors can be integrated into small devices, opening doors for use in wearables and nanotechnology applications.
This trend toward miniaturization is partly driven by advancements in fabrication techniques, like microelectromechanical systems (MEMS) technology. Such techniques allow for the creation of reliable and cost-effective sensors without sacrificing performance. Researchers are now able to explore novel facets of electric fields with devices they can place in hard-to-reach areas. Therefore, miniaturization is not just a trend but a crucial advancement for the future of electric field sensing.
Integration with IoT
The integration of electric field sensors with the Internet of Things (IoT) is another significant advancement reshaping the technological landscape. This merger carries several advantages:
- Real-Time Data Monitoring: IoT-connected sensors facilitate real-time monitoring of electric fields, which is valuable for applications in environmental studies and industrial diagnostics.
- Remote Control and Automation: With IoT integration, users can manage sensors remotely, enhancing adaptability and functionality. Automation of data collection can improve efficiency, particularly in large-scale studies.
- Data Analysis and Insights: Electric field sensors combined with IoT can collect vast amounts of data, paving the way for sophisticated data analytics and insights into patterns that were previously difficult to discern.
With IoT technology becoming increasingly ubiquitous, the potential for electric field sensors to contribute actively to smart systems is greater than ever. As data becomes a primary driver in various industries, the relevance of sensors within this framework will only increase.

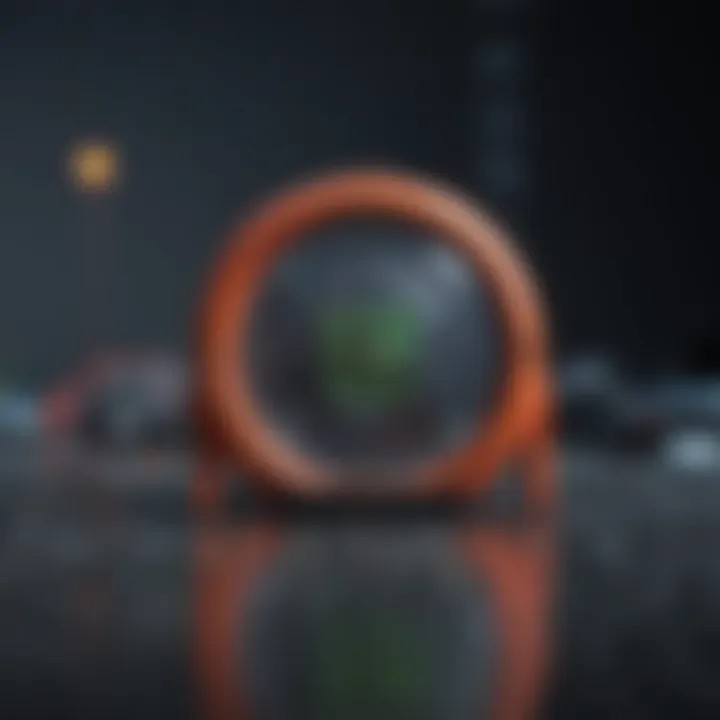
Innovative Materials
The exploration and integration of innovative materials into electric field sensors have been fundamental in enhancing sensor capabilities. Notable materials include:
- Graphene: Graphene’s impressive conductivity and mechanical strength provide benefits like increased sensitivity and durability.
- Conductive Polymers: These materials can be tailored for specific applications, enabling sensors that are lightweight, flexible, and robust against environmental challenges.
- Nanomaterials: Nanotechnology has facilitated the creation of sensors that are not only efficient but also highly sensitive, offering the ability to detect minute changes in electric fields.
Utilizing these advanced materials opens up options for practitioners in developing sensors that meet specific needs, from industrial applications to environmental monitoring. The versatility of these materials contributes significantly to overcoming challenges faced in sensor performance and durability.
"Technological advancements in electric field sensors stand as a catalyst for continuous improvement in performance and applicability."
"Technological advancements in electric field sensors stand as a catalyst for continuous improvement in performance and applicability."
In summary, the current technological advancements in electric field sensors are critical for broadening their applications and enhancing their performance. Miniaturization, IoT integration, and the adoption of innovative materials represent substantial strides in sensor technology, making them indispensable tools in research, industry, and beyond.
Challenges in Electric Field Sensor Development
Electric field sensors play a significant role in many applications, yet their development comes with several challenges. Understanding these challenges is crucial for researchers and engineers. It leads to improvements in sensor design and applications. This section covers sensitivity issues, environmental factors, and calibration challenges. Each aspect affects the performance and reliability of sensors.
Sensitivity Issues
Sensitivity is a critical factor in electric field sensors. It determines how well the device can detect small changes in electric fields. Problems arise due to the inherent noise and interference present in the environment. For instance, electrical noise from nearby devices can mask the signals that sensors are supposed to detect. This situation results in reduced accuracy and precision.
To address these issues, engineers often enhance sensor designs. Some solutions include using higher quality materials that minimize noise or implementing software algorithms for signal processing. However, increasing sensitivity can also lead to increased sensor susceptibility to false positives. It's a delicate balance that requires ongoing research and innovation.
Environmental Factors
Environmental conditions can impact the functioning of electric field sensors. Variations in temperature, humidity, and electromagnetic interference are some common issues. For example, moisture can affect the sensor's insulation properties, leading to erroneous readings. Similarly, changes in temperature can alter the sensor materials, affecting their performance and durability.
Understanding the operating environment is essential before deploying electric field sensors. Devices may require protective coatings or specific housing to mitigate these effects. In some instances, calibration protocols must adapt to changing environmental conditions to ensure reliable data collection.
Calibration Challenges
Calibration of electric field sensors is vital for accurate measurements. The challenge here lies in the complexity of the calibration process, which often requires specialized equipment and conditions. Sensors must not only be calibrated against known references but also regularly recalibrated to maintain accuracy over time.
Another significant issue is that multiple calibration methods exist, each with advantages and disadvantages. Selecting the appropriate method depends on the specific application. This decision can significantly influence the sensor's efficiency and reliability.
Future Trends in Electric Field Sensors
The future of electric field sensors holds significant promise, nestled at the intersection of technological advancement and practical application. As the demand for more efficient sensing solutions rises, understanding the trends shaping this field becomes vital for researchers, engineers, and industries. These sensors are evolving in response to new challenges and opportunities within scientific research, environmental monitoring, and various industrial sectors.
Forecasting Sensor Evolution
Advancements in technology continuously reshape the electric field sensor landscape. Emerging trends suggest a movement toward increased sensitivity and miniaturization. Researchers are developing sensors that can detect smaller changes in electric fields, enhancing their applicability in various domains.
In addition, the integration of machine learning is set to influence sensor design. By analyzing patterns and anomalies in sensor data, algorithms can generate predictive insights. This allows for real-time monitoring and quick response to changing conditions. Furthermore, the trend towards wireless sensors harnesses the potential of IoT. It encourages a shift from tethered systems to more agile, adaptable solutions.
Potential New Applications
The future of electric field sensors is not just about improving technology, but also about broadening their application spectrum. Upcoming areas of focus include:
- Healthcare Monitoring: With a rise in non-invasive diagnostic techniques, electric field sensors could play a role in measuring physiological signals without direct contact.
- Smart Agriculture: By monitoring soil conditions at a granular level, these sensors can enhance precision farming, leading to better yield and resource management.
- Wireless Charging Systems: As the demand for efficient energy transfer technology escalates, the potential for electric field sensors to optimize wireless charging methods is noteworthy.
- Urban Environmental Monitoring: Sensors can help track pollution levels, providing valuable data for urban planning and environmental protection.
Advances in electric field sensors will likely provide not only technical improvements but also innovative applications that push the boundaries of what these devices can accomplish. This growth trajectory clearly indicates a future rich with possibilities.
Culmination
The conclusion of this article encapsulates the essence of electric field sensors, emphasizing their critical role in advancing technology and scientific knowledge. Understanding these sensors is paramount not only for researchers in electrical engineering but also for those in fields like environmental science, industrial automation, and medical technology.
Summarizing Key Insights
In summary, electric field sensors serve as a bridge between theoretical principles and practical applications. They enable the detection and measurement of electric fields in various scenarios, which can lead to breakthroughs in numerous sectors. Key insights from this article include:
- The fundamental principles governing electric fields and how they are harnessed in sensor technology.
- The distinction between passive and active sensors, each with unique operational mechanisms and use cases.
- Various applications ranging from laboratory settings to complex industrial environments that benefit from electric field measurements.
- The ongoing advancements in sensor technology, particularly in miniaturization and integration with IoT systems, making sensors more accessible and efficient.
- Challenges such as sensitivity, environmental factors, and calibration that continue to pose issues in sensor development, necessitating ongoing research and innovation.
Understanding these aspects is crucial for harnessing the full potential of electric field sensors and enhancing their reliability and accuracy in various applications.
Understanding these aspects is crucial for harnessing the full potential of electric field sensors and enhancing their reliability and accuracy in various applications.
Call for Continued Research
The field of electric field sensors is vast and holds significant promise for future exploration. Researchers should focus on:
- Developing more sensitive and precise sensor technologies that can operate effectively in diverse and challenging environments.
- Exploring innovative materials that could lead to better performance and durability in electric field sensors.
- Investigating potential applications in emerging areas, such as smart cities and advanced healthcare monitoring.
Through continued research and development, the impact of electric field sensors can be expanded, potentially changing the way we understand and interact with the electrical phenomena around us. This encourages the scientific community and industries alike to invest time and resources into the ongoing evolution of this technology.