Applications and Implications of Oxygen Permeable Membranes
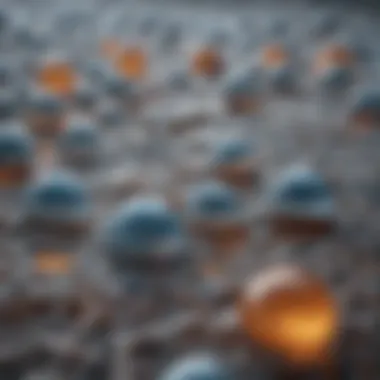
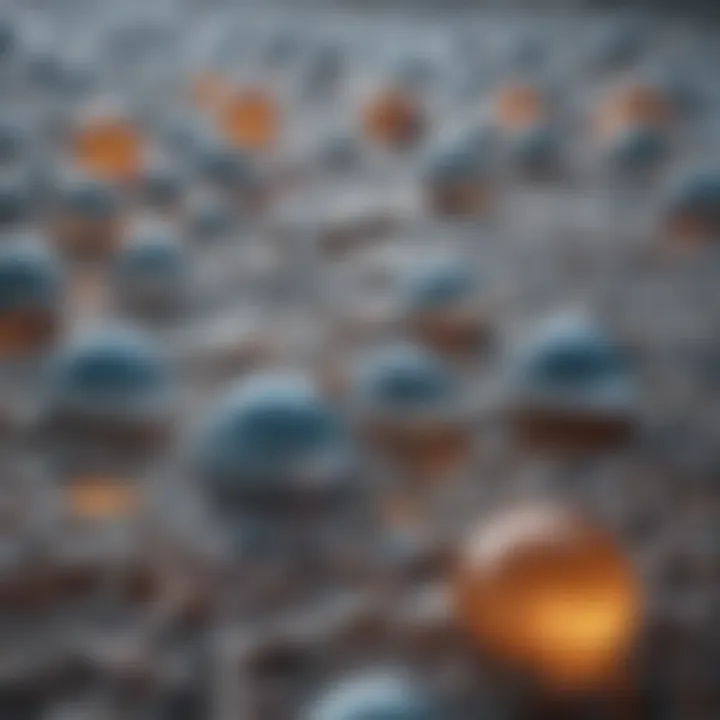
Intro
Oxygen permeable membranes are becoming increasingly crucial in a range of industries, from medicine to environmental science. These membranes allow oxygen to flow through them, serving vital functions in various applications. Understanding the composition and functionality of these membranes provides insights into their significance. By exploring the scientific principles behind them, we can better appreciate how they influence technology and daily life.
Key Concepts
Definition of the Main Idea
Oxygen permeable membranes, as the name indicates, are designed to permit the passage of oxygen molecules while blocking other substances. Made from a variety of materials, these membranes are fundamental to processes where controlled oxygen transfer is necessary. This makes them vital in medical technologies such as oxygen therapy devices and in environmental applications like gas separation processes.
Overview of Scientific Principles
The behavior of oxygen permeable membranes hinges on certain scientific concepts, particularly the principles of diffusion and permeability. Diffusion is the movement of molecules from areas of higher concentration to lower concentration. The rate of diffusion through a membrane is determined by its material properties, thickness, and temperature. Additionally, permeability is defined as how easily a substance can pass through a material. In the case of oxygen permeable membranes, factors such as pore size and chemical composition play significant roles in guiding their efficiency.
Current Research Trends
Recent Studies and Findings
Research in the area of oxygen permeable membranes has seen significant advancements. Studies indicate a growing interest in developing next-generation materials that enhance membrane performance. Recent findings suggest that incorporating nanomaterials can improve both oxygen permeability and selectivity. This includes developments in polymer composites that increase durability without compromising flexibility.
Significant Breakthroughs in the Field
One notable breakthrough in the field involves the synthesis of more responsive and adaptive membranes. Advances in material science have created membranes that can adjust their permeability based on environmental conditions. This innovation allows for more efficient operations in applications ranging from industrial oxygen supply systems to medical devices used in critical care settings.
"The development of sophisticated oxygen permeable membranes marks a transformative approach in both industrial and healthcare sectors, paving the way for more effective solutions."
"The development of sophisticated oxygen permeable membranes marks a transformative approach in both industrial and healthcare sectors, paving the way for more effective solutions."
Prolusion to Oxygen Permeable Membranes
Oxygen permeable membranes play a significant role in various fields, from healthcare to environmental science. These specialized membranes allow for the selective passage of oxygen while blocking other gases. This unique characteristic can lead to improved care in medical applications, efficient environmental controls, and innovative industrial solutions. As the demand for advanced technologies continues to grow, understanding the principles and applications of these membranes becomes essential.
Definition and Importance
Oxygen permeable membranes are materials engineered to selectively permit oxygen to pass through while restricting the flow of other substances. These membranes are vital in numerous applications. In the medical field, they are integral to wound healing, aiding in oxygen transport to tissues, which is crucial for recovery. In environmental sectors, they assist in reducing pollutants and improving air quality.
The importance of these membranes also extends to manufacturing and packaging, particularly in preserving food products. By allowing oxygen to circulate while keeping out unwanted materials, companies can enhance the shelf life of products. The intricacies of how these membranes function and their material composition are key to maximizing their benefits.
Historical Context
The development of oxygen permeable membranes dates back several decades, originating from the need for more effective medical and industrial solutions. Early research focused on the natural properties of materials that can pass oxygen while filtering other elements. The technological breakthroughs in polymer science have propelled this area forward.
In the 1970s and 1980s, significant progress was made in synthesizing polymer-based membranes, leading to advances in both laboratory settings and commercial products. Over time, the invigorating fusion of chemistry and engineering has produced membranes that are not only more efficient but also scalable for large-scale productions.
Later advancements brought more intricate designs, including the integration of composite materials, which enhanced permeability while maintaining durability. This historical progression has laid a foundation for the current diversity of oxygen permeable membranes available today, with implications in diverse sectors.
Principles of Gas Permeability
The principles of gas permeability are fundamental to understanding how oxygen permeable membranes function. These principles define the mechanisms through which gases pass through these membranes and explain the factors that affect this movement. Recognizing these elements is essential as they not only guide the design of membranes but also influence their applications in various fields, such as medicine and environmental science.
Diffusion Mechanisms
Diffusion is a key mechanism in the transport of gases through membranes. In simple terms, diffusion occurs when molecules move from an area of higher concentration to an area of lower concentration. This movement is driven by the concentration gradient and continues until equilibrium is reached. For oxygen permeable membranes, this process is critical. Oxygen molecules enter the membrane's surface, typically where the concentration is higher, and migrate through the membrane to areas of lower concentration.
Membranes can be categorized based on their structure. Porous membranes contain tiny holes allowing gas molecules to pass through easily, while dense membranes require gas molecules to dissolve in the membrane material before diffusing through.
In addition to diffusion, other processes such as convection and permeation can occur in specific scenarios.
Factors Influencing Permeability
Several factors influence the gas permeability of membranes. Understanding these factors is essential for optimizing membrane performance. Such factors include:
- Material Composition: The type of material used in the membrane significantly affects its permeability. Different polymers or composites display distinctive diffusion properties. For example, silicone-based membranes may allow for more oxygen to pass compared to polyethylene.
- Temperature: Generally, an increase in temperature enhances gas permeability. Higher thermal energy allows gas molecules to move more freely, which improves diffusion rates within the membrane.
- Pressure Gradient: A higher pressure gradient can also drive more efficient gas transfer. When there is significant pressure difference between the two sides of the membrane, the movement of gas molecules increases.
- Thickness of the Membrane: Thicker membranes may reduce permeability since gas has to travel a longer distance. Thus, optimizing membrane thickness is crucial in design.
The interplay of these factors can lead to considerable variations in membrane performance, necessitating careful material selection and environmental control.
The interplay of these factors can lead to considerable variations in membrane performance, necessitating careful material selection and environmental control.
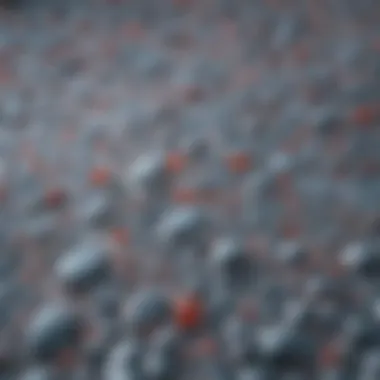
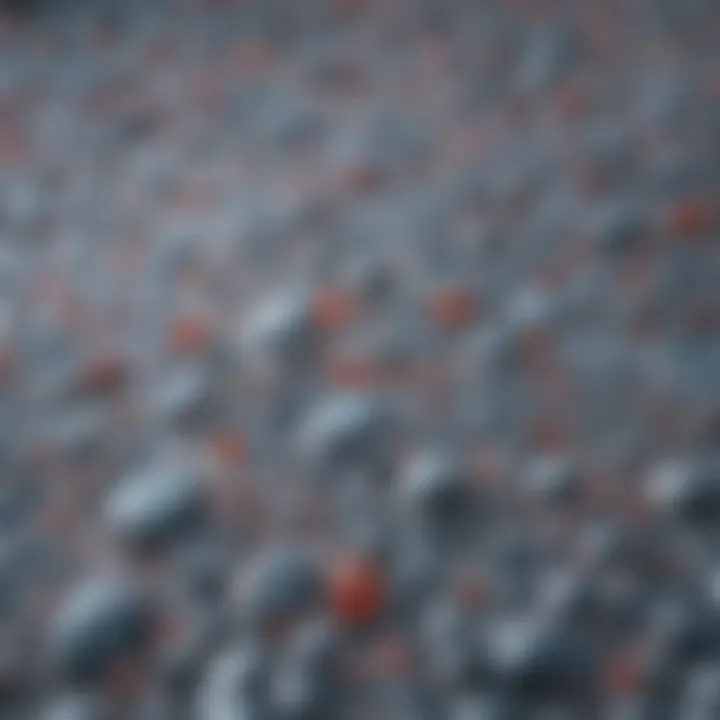
This understanding of the principles and factors of gas permeability is pivotal in advancing technologies that rely on oxygen permeable membranes, ensuring they meet industry standards and application needs.
Material Science of Membranes
The material science of membranes is a crucial component in understanding oxygen permeable membranes. The choice of materials directly affects the function, efficiency, and application scope of these membranes. Scientists and engineers look for specific properties, such as mechanical strength, flexibility, and gas permeability. These factors play a pivotal role in determining the performance and durability of membranes in various settings.
Moreover, material selection impacts the cost-effectiveness and scalability of production. As industries increasingly rely on these membranes for diverse applications, the importance of innovative material science rises.
Common Materials Used
Oxygen permeable membranes are made from a variety of materials, each offering unique properties. Some common materials include:
- Polymeric Materials: Polymers such as polyethylene and polyurethane are popular due to their flexibility and ease of processing. They can offer good gas permeability while maintaining structural integrity.
- Ceramic Membranes: Ceramics like zirconia are known for their high thermal stability and chemical resistance. These materials are suitable for high-temperature applications, making them valuable in industrial settings.
- Metallic Membranes: Stainless steel and other metal membranes are applicable in environments where durability is essential. They often exhibit excellent gas separation properties but can be less flexible than non-metallic counterparts.
Each of these materials has to be carefully considered based on the specific needs of the application they are intended for.
Innovative Composite Structures
The field is witnessing exciting developments in composite membrane structures. These combinations of materials can enhance performance significantly. Composite membranes leverage the strengths of different materials to overcome limitations that single materials often face. Key benefits include:
- Improved Selectivity: By combining materials, it is possible to create membranes that selectively allow oxygen to pass while blocking other gases. This selectivity is crucial in applications like respiratory devices.
- Enhanced Mechanical Properties: Composite membranes often exhibit better mechanical properties compared to their individual components. This leads to increased durability in challenging environments.
- Tailored Permeability: Innovations allow for adjustments in the permeation rates of gases through engineering the composite structure. This results in more effective membranes adapted for specific purposes.
"In pursuing advanced applications, the understanding and innovation in material science are vital for developing oxygen permeable membranes that meet the growing demands of technology and industry."
"In pursuing advanced applications, the understanding and innovation in material science are vital for developing oxygen permeable membranes that meet the growing demands of technology and industry."
Applications in Medicine
Oxygen permeable membranes find significant importance in the medical field. These membranes play a crucial role in enhancing various therapeutic and diagnostic procedures. Their unique ability to facilitate the controlled passage of oxygen makes them invaluable in several applications. Key areas of focus include wound healing technologies and respiratory assist devices. Each application leverages the distinctive properties of these membranes, which results in improved patient outcomes and treatment efficacy.
Wound Healing Technologies
Wound healing is a complex process that can be hampered by factors such as infection and inadequate oxygen supply. Oxygen permeable membranes are designed to mimic the body's natural healing processes. They can provide a moist environment that promotes cellular regeneration and scabbing. This moisture retention accelerates healing while preventing the formation of scars. Furthermore, by allowing for the diffusion of oxygen directly to the wound site, these membranes can mitigate hypoxia, a condition known to hinder recovery.
- Benefits include:
- Enhanced oxygen delivery to tissue
- Reduction in infection rates
- Improved overall healing times
These membranes are often incorporated into dressings or employed in advanced therapies, such as bioengineered skin grafts. Effective usage requires careful material selection and consideration of permeability rates to match the specific wound type.
"Oxygen availability is critical for cellular function, making oxygen permeable membranes an essential tool in modern wound care."
"Oxygen availability is critical for cellular function, making oxygen permeable membranes an essential tool in modern wound care."
Respiratory Assist Devices
In the realm of respiratory care, oxygen permeable membranes are integral to devices such as oxygen concentrators and continuous positive airway pressure (CPAP) machines. These devices assist patients suffering from respiratory ailments. They enable the efficient transfer of oxygen while filtering out carbon dioxide. This function is vital for patients with conditions like chronic obstructive pulmonary disease (COPD) or those requiring supplemental oxygen.
The membranes' design ensures a fine balance between gas exchange and mechanical stability. This is essential, especially in life-support situations, where reliability is paramount.
- Key characteristics of respiratory devices include:
- High permeability for optimal gas exchange
- Biocompatibility for patient safety
- Durability to withstand rigorous use
Role in Environmental Science
Oxygen permeable membranes play a fundamental role in environmental science, acting as crucial tools to address significant challenges related to pollution and water management. Their unique characteristics enable various applications that help mitigate environmental degradation. Understanding these applications is key to both current practices and future innovations in environmental technology.
Pollution Control
Oxygen permeable membranes are essential in managing industrial emissions and reducing pollutants in the air. These membranes facilitate the selective transport of gases, allowing oxygen to permeate while blocking harmful substances. This feature makes them valuable in processes such as flue gas treatment systems.
The main benefits of using these membranes for pollution control include:
- Increased Efficiency: Membranes can enhance separation processes, making it easier to remove pollutants from gas streams.
- Cost-Effectiveness: Utilizing oxygen permeable membranes can lead to lower operational costs compared to traditional scrubbers or adsorbers.
- Reduced Footprint: Membrane systems often occupy less space than other treatment technologies, making them suitable for installations where space is limited.
Incorporating these membranes can also lead to significant improvements in the recycling of gases in industrial settings. By capturing valuable components, industries can minimize waste while maximizing resource utilization.
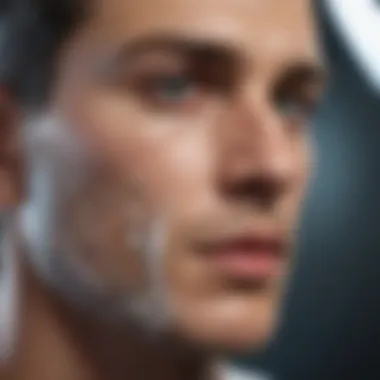
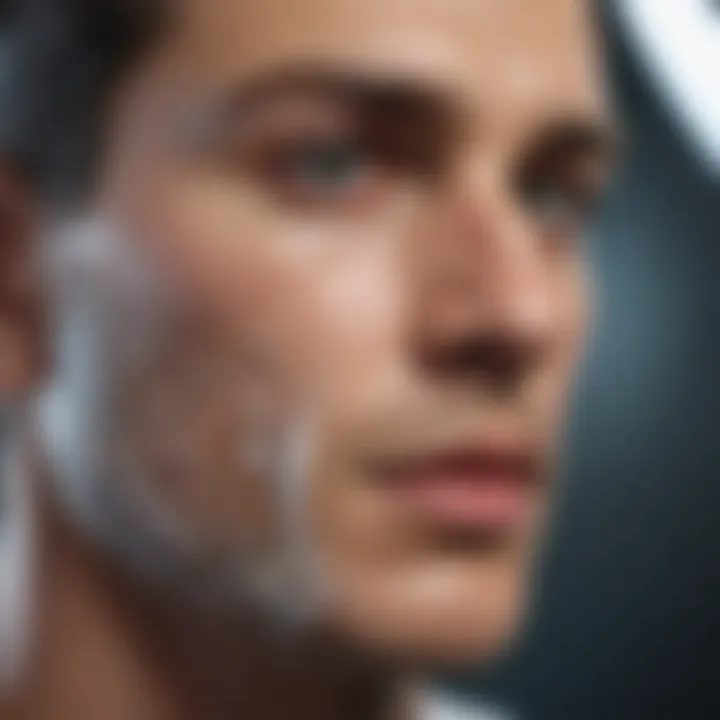
"Oxygen permeable membranes enhance gas separation efficiency, paving the way for cleaner technologies and sustainable methods in pollution control."
"Oxygen permeable membranes enhance gas separation efficiency, paving the way for cleaner technologies and sustainable methods in pollution control."
Water Treatment Technologies
In the realm of water treatment, oxygen permeable membranes find diverse applications aimed at improving the quality of drinking water and managing wastewater. The membranes can support processes like membrane aeration, where they provide oxygen to microorganisms involved in biological treatments. This further enhances the breakdown of organic materials, which is crucial in wastewater facilities.
Key aspects of water treatment technologies using these membranes include:
- Enhanced Biological Activity: By supplying oxygen, these membranes optimize conditions for aerobic processes, which can lead to quicker and more efficient waste degradation.
- Reduction of Chemical Use: The reliance on oxygen permeable membranes can lessen the need for external chemicals, allowing for a more sustainable approach to water treatment.
- Modular Systems: Membrane technologies can be designed modularly, allowing for extensive setups that can be adjusted to specific treatment needs, enhancing flexibility in various environmental applications.
The integration of oxygen permeable membranes into water treatment systems signifies a move towards more sustainable methods, ensuring better water quality for communities and reducing environmental impacts. It emphasizes the ongoing need for research and innovative solutions in the field of environmental science.
Industrial Applications
Oxygen permeable membranes have substantial relevance in various industries. Their ability to selectively allow the passage of oxygen while blocking other gases presents unique advantages. These membranes are not just critical in maintaining atmospheric conditions but also enhance product shelf life and improve energy efficiency across several domains.
Food Packaging Solutions
Food preservation is one of the key areas where oxygen permeable membranes are utilized. These membranes regulate the oxygen exposure in packaged foods, allowing for a controlled environment that extends freshness. One of the main benefits is the reduction of spoilage due to aerobic bacteria and fungus.
- Modified Atmosphere Packaging (MAP): This technique is crucial in the food industry. By adjusting oxygen and carbon dioxide levels using membranes, the prolongation of food freshness becomes achievable.
- Barrier Properties: Oxygen permeable membranes offer excellent barrier properties, which helps in keeping the food safe from external contaminants.
- Sustainability: Using these membranes can significantly reduce food waste, aligning industrial practices with sustainability goals.
Energy Generation Technologies
In the energy sector, the role of oxygen permeable membranes can be pivotal. They are used in processes that require efficient gas separation, particularly in fuel cells. The functionality of these membranes impacts the sustainability and efficiency of energy production.
- Solid Oxide Fuel Cells (SOFC): These technologies depend on oxygen ionic conductivity. The membrane's quality directly influences the overall efficiency and performance of the fuel cell.
- Biomass Energy Harvesting: Utilizing membranes in the gasification of biomass leads to better oxygen management, which enhances energy yield.
- Cost Reduction: By improving the efficiency of energy-generating systems, oxygen permeable membranes can contribute to lowering operational costs for industrial energy solutions.
"The integration of oxygen permeable membranes in industrial applications is a significant step toward optimizing processes and enhancing product quality."
"The integration of oxygen permeable membranes in industrial applications is a significant step toward optimizing processes and enhancing product quality."
The advancements in membrane technology continue to evolve, leading to better industrial practices. The key lies in understanding how the properties of oxygen permeable membranes can be harnessed effectively across different industrial applications.
Recent Advances in Research
Recent developments in research on oxygen permeable membranes have contributed significantly to the understanding and functionality of these materials. Investigating the latest methodologies and technologies can provide insights that have far-reaching implications for various sectors. Research in this area is essential not only for theoretical advancements but also for practical applications that can enhance existing technologies.
Nanotechnology and Membranes
Nanotechnology has reshaped the landscape of membrane technology by enabling the manipulation of materials at the molecular or atomic level. This approach enhances the performance and efficiency of oxygen permeable membranes in several ways. By creating membranes with nano-sized pores, researchers can improve gas selectivity and permeability. The introduction of nanomaterials, such as carbon nanotubes or graphene, leads to membranes that possess high strength and flexibility, meeting industrial demands.
These advances also extend to the surface modification techniques employed to regulate the interaction between membranes and various gases. For instance, functionalizing membranes at the nanoscale allows for tailored responses to environmental changes, which is highly beneficial in applications like controlled drug delivery systems. The increased surface area provided by nanotechnology contributes to better gas exchange, which is crucial in medical and environmental applications.
Key points regarding nanotechnology in membranes include:
- Enhanced permeability: Smaller pores allow for more efficient gas transport.
- Selective filtration: Tailored properties can exclude unwanted gases.
- Durable structures: Nanomaterials often have superior resistance to wear and degradation.
Biomimicry in Membrane Design
The application of biomimicry in membrane design has opened new avenues for creating responsive and efficient oxygen permeable membranes. By studying biological systems, researchers can adopt designs that have evolved over millions of years, optimizing performance for specific applications.
For example, natural membranes, such as those found in certain plants or animals, exhibit excellent gas exchange capabilities. Mimicking these structures allows for the development of membranes that can replicate these efficiencies. Researchers draw inspiration from the structure of human lungs or aquatic organisms, leading to innovative designs that capitalize on natural phenomena.
Biomimetic membranes are often characterized by:
- Enhanced gas exchange: Designs inspired by nature can facilitate improved oxygen transport.
- Environmental adaptability: These membranes can respond to varying conditions, much like natural systems do.
- Sustainability: Many biomimetic designs incorporate eco-friendly materials, reducing environmental impact.
Both nanotechnology and biomimicry represent pivotal trends in the evolution of oxygen permeable membranes. Understanding these advances will not only help to elevate current practices but also encourage future innovations that align with technological and ecological needs.
Recent advancements in nanotechnology and biomimicry in membrane design highlight the potential to revolutionize applications across industries. Both approaches promise enhanced performance and sustainability.
Recent advancements in nanotechnology and biomimicry in membrane design highlight the potential to revolutionize applications across industries. Both approaches promise enhanced performance and sustainability.
Challenges and Limitations
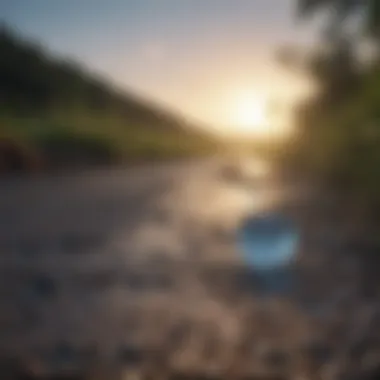
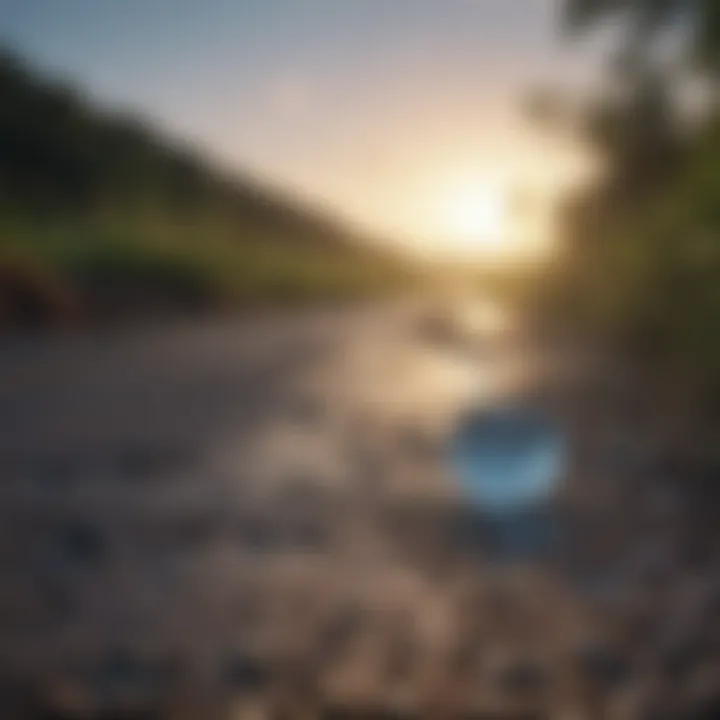
Understanding the challenges and limitations surrounding oxygen permeable membranes is crucial. These membranes hold promise in various applications, but they are not without hurdles that researchers and industries must navigate. Addressing these issues is essential for optimizing their use and expanding their applicability in advanced technologies.
Material Durability
Material durability is one of the primary challenges faced by oxygen permeable membranes. Over time, exposure to environmental factors such as moisture, temperature fluctuations, and chemical interactions can degrade membrane performance. The degradation may result in reduced gas permeability, impacting functionality and effectiveness in applications.
This durability concern leads to various considerations:
- Design Choices: Selecting the appropriate materials is vital. For instance, membranes made of polyurethane or silicone often show different durability profiles. Manufacturers must evaluate performance requirements against environmental conditions to make informed choices.
- Longevity Testing: Regular testing helps predict the lifespan of membranes. Establishing testing protocols helps in understanding how different materials behave under stress.
- Preventive Measures: Applying coatings or additives can enhance durability. These modifications can help membranes resist wear, thus prolonging their active lifespan.
Scalability Issues
Scalability presents another significant limitation. Transitioning from lab-scale production to industrial-scale manufacturing often reveals unforeseen obstacles. These challenges include:
- Economic Factors: The cost of materials and production can be prohibitive. Economies of scale may not be easily achievable for some membrane types. Hence, the initial investment may not align with market expectations.
- Production Techniques: Current manufacturing techniques may limit membrane scalability. Processes that work well at a smaller scale may encounter inefficiencies or technical difficulties when scaled up.
- Regulatory Hurdles: Ensuring compliance with industry regulations is vital. The additional steps required to meet these standards can slow down the production ramp-up process.
Future Trends and Directions
The landscape of oxygen permeable membranes is evolving rapidly. Understanding these shifts is critical for researchers and professionals in various fields. This section will discuss the emerging technologies revolutionizing the industry and the potential integration of these membranes in smart systems. Both elements present notable advantages and implications for future applications.
Emerging Technologies
Recent advancements in technology are significantly impacting the development of oxygen permeable membranes. A prime focus has been on nanotechnology. By manipulating materials at the nanoscale, researchers can enhance the properties of membranes, resulting in increased efficiency and improved functionality. Various nanostructured materials are being integrated into membrane designs, leading to superior gas exchange capabilities.
Moreover, bio-inspired materials are gaining traction. These are materials that mimic the natural processes found in biological systems. For instance, designing membranes that replicate the selective permeability of cell membranes can drastically improve performance.
In addition, there is a strong interest in advanced manufacturing techniques, including 3D printing. This allows for greater control over membrane structure and microarchitecture. Tailored designs can be created to meet specific requirements in various applications such as medicine and environmental science.
Key benefits of these emerging technologies include:
- Enhanced Performance: Increased efficiency in gas separation processes.
- Cost-Effectiveness: Reduced production costs through innovative techniques.
- Customization: Ability to design membranes for specialized applications.
Integration in Smart Systems
The integration of oxygen permeable membranes in smart systems marks a new phase in their applications. Smart systems, which utilize connectivity and data processing, can greatly benefit from these membranes. For instance, incorporating them into sensors and monitoring devices can lead to real-time feedback on environmental conditions.
The use of IoT (Internet of Things) technologies in conjunction with oxygen permeable membranes has the potential to revolutionize various industries. In agriculture, for example, smart farming techniques can leverage these membranes to monitor soil quality and optimize oxygen levels for plant growth. This approach can significantly enhance productivity and sustainability.
A few considerations for this integration include:
- Energy Efficiency: Reducing energy consumption in industrial applications.
- Scalability: Ensuring that technologies can be implemented on a larger scale.
- Data Management: Managing data effectively in smart applications.
The future direction of oxygen permeable membranes lies in their adaptability to emerging technologies and smart systems. This adaptability can open new avenues for research and practical use in various fields.
The future direction of oxygen permeable membranes lies in their adaptability to emerging technologies and smart systems. This adaptability can open new avenues for research and practical use in various fields.
Finale and Implications
The exploration of oxygen permeable membranes signifies a pivotal advancement in various fields, ranging from medicine to environmental science and industrial applications. Understanding the implications of these membranes is crucial. They are not just materials but also integral components that support the development of innovative solutions to complex problems. The benefits they provide are extensive, including improved healing processes in medical applications, enhanced food preservation in packaging, and effective pollution control technologies.
In recent years, the focus on competence and adaptability of these membranes has augmented their significance. They serve essential functions by allowing controlled release and uptake of oxygen and other gases, which is indispensable in several contexts. The integration of advanced materials and novel designs enhances the performance and durability of these membranes. This, in turn, directly impacts their real-world applicability, demonstrating the need for ongoing research to refine and improve their characteristics.
Furthermore, during the ongoing discussion about sustainability, materials with specific gas permeability can support eco-friendly technologies. Oxygen permeable membranes offer benefits that align with environmental protection goals, elevating them as key players in the sustainable development agenda.
The applications of oxygen permeable membranes are becoming a transformative force in modern technology, merging science and practical needs.
The applications of oxygen permeable membranes are becoming a transformative force in modern technology, merging science and practical needs.
Understanding nuanced aspects such as scalability, material choice, and long-term reliability must be highlighted when considering future applications. As industries continue to evolve, the implications of advanced membrane technologies will grow even more significant, marking the membranes as critical elements in many applications.
Summary of Findings
This article delves into the multifaceted nature of oxygen permeable membranes, outlining their structure, function, and wide-ranging applications. Key findings include:
- Materials and Composition: The success of membranes is heavily reliant on their composition. Common materials such as silicone and polyvinylidene fluoride are frequently employed due to their excellent gas permeability.
- Medical Applications: In healthcare, these membranes are instrumental in wound healing technologies and respiratory assist devices, showing promising results in patient recovery and care.
- Environmental Impact: Membranes contribute significantly to pollution control efforts and water treatment technologies, showcasing their potential in promoting environmental sustainability.
- Industrial Uses: The industrial applicability of oxygen permeable membranes spans food packaging and energy generation technologies, highlighting their versatility.
- Research and Development: Recent advances in nanotechnology and biomimicry are paving the way for future improvements in membrane efficiency and functionality.
These findings solidify the importance of oxygen permeable membranes in modern science and technology. They also serve as a basis for further inquiry into their capabilities and potential applications.
Significance for Future Research
Future research in the realm of oxygen permeable membranes is not merely beneficial; it is fundamental for innovation across various sectors. The continuous evolution of technologies mandates a fresh perspective on membrane applications. Key areas of focus include:
- Material Innovation: Researchers should investigate alternative materials that enhance permeability and durability while maintaining low environmental impact.
- Interdisciplinary Approaches: Collaboration between disciplines such as chemistry, biology, and materials science could yield breakthroughs that expand the functionality of these membranes.
- Scalability and Manufacturing: Addressing scalability issues remains a significant concern. Research into more efficient manufacturing processes can help overcome barriers to large-scale application.
- Integration with Smart Systems: As smart technology advances, the incorporation of oxygen permeable membranes into such systems could lead to newly designed products that are both functional and adaptive.
- Environmental Sustainability: Future studies must continuously assess the role of these membranes in promoting sustainable practices and their long-term effects on the environment.