Understanding Quantum Frequency in Depth
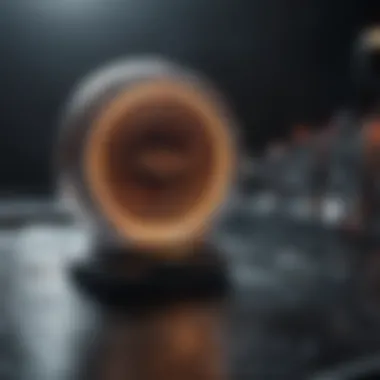
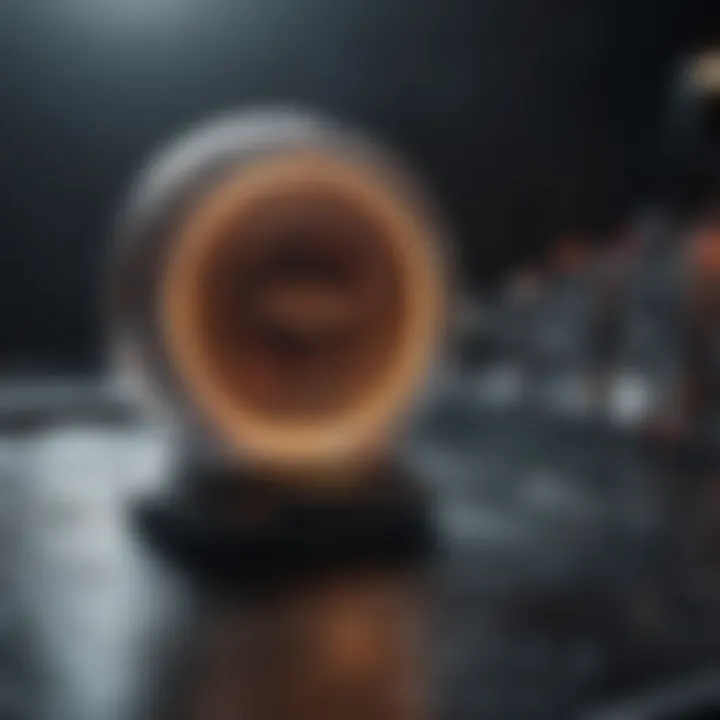
Intro
The concept of quantum frequency often seems elusive, caught between the abstract world of theoretical physics and the tangible realm of technology. Understanding this topic is essential not just for academics and students but also for anyone interested in how it shapes the future of our technological landscape. In this article, we’ll journey through the foundations of quantum frequency, shedding light on its complex characteristics, its pivotal role in quantum mechanics, and its fascinating implications in advancements like quantum computing and communication.
Key Concepts
Definition of the Main Idea
At its core, quantum frequency can be described as the frequency of a quantum system's wave functions. Unlike traditional frequency, which is measured in Hertz and pertains to cycles of a wave, quantum frequency reflects the energy levels of particles and their transitions. It's the heartbeat of quantum phenomena, dictating how particles behave at the tiniest scales.
Overview of Scientific Principles
The principles governing quantum frequency are tethered closely to quantum mechanics and wave-particle duality. When particles such as electrons transition between energy levels, they emit or absorb energy in quantized amounts, often described by Planck’s equation. Some essential principles to consider include:
- Wave-particle duality: Every particle can exhibit characteristics of waves, and quantum frequency is a result of this relationship.
- Energy quantization: Quantum systems can only have specific energy values, leading to distinct frequencies that correspond to transitions between these states.
- Uncertainty principle: Introduced by Heisenberg, it suggests limits to how precisely we can know a particle's frequency and position simultaneously.
These principles illustrate the fluid and often counterintuitive nature of quantum mechanics, setting the stage for our discussion.
Current Research Trends
Recent Studies and Findings
A myriad of research is currently being poured into understanding the implications of quantum frequency. For instance, a recent exploration delves into how quantum frequency impacts the coherence times of qubits in quantum computing. The findings underscore a direct correlation between frequency stability and computational power, making it a critical area for future advancements.
Another study addresses quantum frequency's relevance in quantum cryptography, suggesting that precise timing can enhance the security of quantum key distribution methods.
Significant Breakthroughs in the Field
Recent years have seen significant breakthroughs, particularly in the realms of technology and fundamental physics.
- Entangled states: Research has demonstrated that manipulating the quantum frequency of entangled particles can potentially be utilized for faster-than-light communication, raising eyebrows within the scientific community.
- Quantum sensing: Innovations in devices leveraging quantum frequency may provide unprecedented accuracy in measuring physical quantities, directly impacting fields like gravitational wave detection.
- Quantum networks: Efforts are underway to create quantum networks that utilize quantum frequency for improved information transfer, potentially revolutionizing internet technology.
These advances highlight the practicality of quantum frequency, making it clear that it’s more than an abstract concept; it’s a gateway into the next revolution in technology and understanding.
"Understanding quantum frequency is tantamount to opening a door to a universe of possibilities, folding reality into realms where the familiar meets the fantastical."
"Understanding quantum frequency is tantamount to opening a door to a universe of possibilities, folding reality into realms where the familiar meets the fantastical."
In the following sections, we will further dissect the implications of quantum frequency in various physical phenomena, investigating how these principles shape not only theoretical discussions but also practical applications in technology.
Understanding Quantum Frequency
Quantum frequency sits at the heart of quantum mechanics, shaping not just theoretical frameworks but also practical applications that are transforming technology. Its importance is underscored by how it interacts with fundamental concepts such as energy, waves, and particles. Understanding quantum frequency allows researchers and enthusiasts alike to grasp the nuances of phenomena that aren't easily observable but have profound impacts on the world around us.
In this discussion, we dive deep into the key elements of quantum frequency, exploring its benefits and the considerations surrounding it. Central to grasping quantum mechanics is recognizing that frequency, in the quantum sense, is not simply a measure of cycles or waves, but a reflection of the energy states of particles, which highlights its complexity and the critical role it plays in various scientific theories and technological advancements.
Additionally, comprehending quantum frequency leads to insights that can disrupt conventional views and methods. With its potential applications ranging from quantum computing to cryptography, it’s clear that a thorough understanding of this concept is not just academic but vital for future innovations.
Defining Quantum Frequency
At its core, quantum frequency refers to the rate at which a quantum system oscillates. While classical frequency might be easier to picture—such as a pendulum swinging back and forth—quantum frequency operates within the framework of quantum mechanics. Here, it intertwines with the energy states of particles like electrons and photons. Essentially, the higher the frequency, the more energy the particle possesses. This relationship is pivotal in understanding phenomena like photon emission in atomic transitions.
In mathematical terms, the connection can be expressed through the equation:
E = h * f
Where E is energy, h stands for Planck's constant, and f indicates frequency. This equation illustrates that the quantum frequency of a particle determines its energy, creating a foundation for various interpretations and applications in quantum theories. The implications of these quantum frequencies extend beyond the laboratory into areas such as telecommunications and medical imaging, where they ensure accurate measurements and efficient data transmission.
Historical Context and Development
The journey to understanding quantum frequency has been shaped by numerous milestones in physics. The work of Max Planck at the turn of the 20th century was crucial, as he introduced the concept of quantization of energy, which formed the bedrock of quantum mechanics. His revolutionary proposal that energy is emitted or absorbed in discrete packets (quanta) rather than in continuous waves laid the groundwork for later developments.
As the field evolved, figures like Albert Einstein further elucidated these ideas, especially with his explanation of the photoelectric effect, where electrons are emitted from material upon being exposed to light. This phenomenon connects back to quantum frequency by illustrating how electromagnetic waves (or light) can behave like particles with defined energy levels, each corresponding to a specific frequency.
In the decades following these foundational theories, experimental advancements have continuously validated and expanded upon initial concepts, creating a robust framework that scientists rely on today. Discussions about quantum frequency highlight not only historical milestones but also the evolution of our understanding of the universe. By recognizing this history, one can appreciate the depth and significance of quantum frequency in the broader narrative of quantum mechanics.
"The concept of frequency in quantum mechanics reshapes how we think about energy and interaction in the universe."
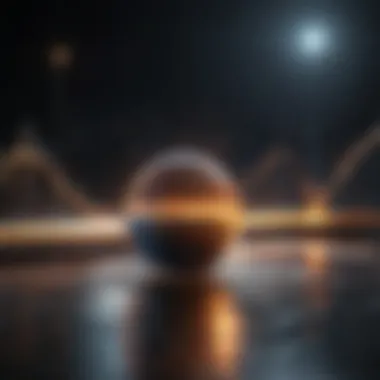
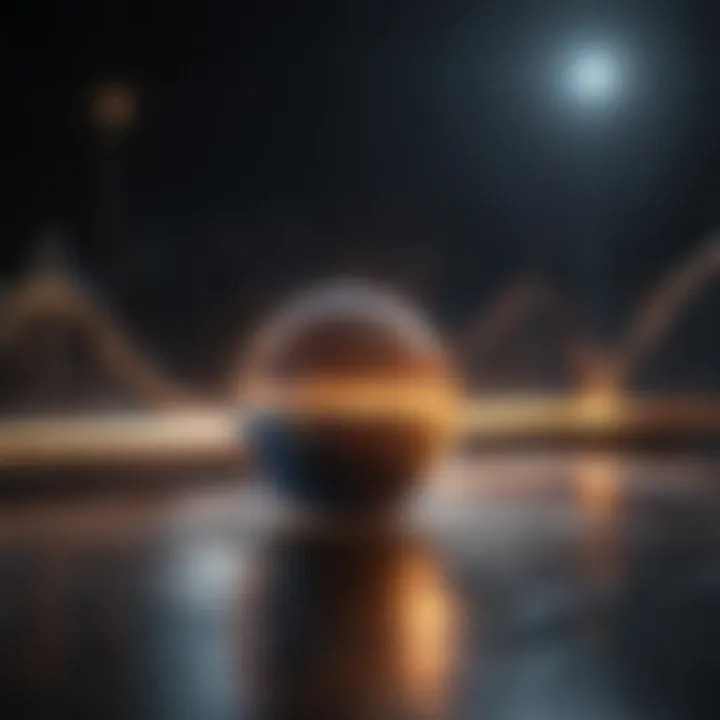
"The concept of frequency in quantum mechanics reshapes how we think about energy and interaction in the universe."
In summary, quantum frequency encapsulates a complex interplay of theoretical knowledge and practical implications. Understanding its definition and historical evolution provides a necessary context to appreciate its role in contemporary science and technology.
Theoretical Foundations
Understanding the foundations of quantum theory is like building the base of a great skyscraper; it allows for everything that comes after it to stand tall and strong. In the context of quantum frequency, theoretical grounding provides insights that help us make sense of phenomena that might, at first glance, seem unfathomable. At the heart of this discussion lies the interplay between matter and energy, and how quantum mechanics fundamentally reshapes our perspective on physical reality. This section delves into the philosophical and scientific elements of quantum theory that lend credence to the concept of quantum frequency, laying the groundwork for a more detailed exploration in subsequent sections.
Wave-Particle Duality
The principle of wave-particle duality is perhaps one of the most intriguing hallmarks of quantum mechanics. This concept argues that every particle or quantic entity may be described as either a particle or a wave. Traditionally, we might see light as either a stream of particles called photons or as electromagnetic waves; in quantum mechanics, it embodies both characteristics simultaneously. This duality is not just a quirky nuance; it has profound implications for quantum frequency.
When we measure light as a wave, we are dealing with frequencies and wavelengths, while treating it as particles brings us into the world of quantum states and energy absorption. For instance, when considering how light interacts with atoms—like a game of cosmic billiards—its behavior changes depending on whether we assess it as particles or waves. More formally, this conception of duality leads to the conclusion that quantum frequency can be viewed as an intrinsic property of a quantum state, depending on how its interactions manifest. Thus, grasping wave-particle duality lays a crucial foundation for understanding quantum dynamics and, consequently, frequency.
Quantum State and Frequency
Quantum states provide the framework within which quantum systems exist. Every particle or system of particles can be described by a quantum state characterized by a wave function, reflecting the probability distribution for various measurable quantities, including position, momentum, and yes, frequency. The concept of frequency in quantum mechanics emerges closely from the properties of these quantum states.
When analyzing a quantum state, it's essential to recognize that different frequencies correlate with different energy levels. For instance, in an atom, electrons exist in various energy states, and when they transition from one state to another, they either absorb or emit photons of particular frequencies. You could imagine it as music: each unique note corresponds to a specific frequency that resonates differently within the fabric of quantum mechanics.
The mathematical representation of quantum states reveals that frequency is not just a number; it's a fundamental descriptor of a system's behavior. Therefore, comprehending quantum states is integral for delving into the realm of quantum frequencies.
Role of Planck's Constant
No discussion of quantum mechanics and quantum frequency would be complete without addressing Planck's constant. This incredibly small number, approximately 6.626 × 10^-34 Js, serves as a bridge between classical physics and quantum mechanics. It plays a pivotal role in defining the relationship between frequency and energy, articulated through the simple yet powerful equation:
[ E = h imes f ]
Where:\n - E is energy,
- h is Planck's constant, and
- f is frequency.
This equation underscores that the energy of a photon is directly proportional to its frequency. It’s this proportionality that illuminates how quantum frequency doesn't merely exist in isolation; it is fundamentally linked to the energy dynamics at play within a quantum system.
By understanding the role of Planck's constant, researchers can also develop new technologies, especially in fields like quantum computing or quantum cryptography, where these interactions and transitions are exploited for advancement. Knowing how the smallest element of a quantum system interplays with frequency helps us unravel larger mysteries in physics and technology.
Mathematical Representation
When we venture into the realm of quantum frequency, understanding its mathematical representation becomes crucial. Math provides a framework that helps us comprehend and predict the behaviors of quantum particles, which often operate beyond the grasp of classical intuition. This section will clarify how mathematical formulations aid in grasping the complexities tied to both frequency and energy, considering that these elements are not merely abstract concepts but foundational to various quantum phenomena.
Frequency and Energy Relation
In quantum mechanics, the relationship between frequency and energy is succinctly expressed through the renowned equation:
E = hν
where E represents energy, h is Planck's constant (approximately 6.626 × 10^(-34) Js), and ν (nu) denotes frequency. This relationship asserts that energy is directly proportional to frequency. So, as frequency increases, energy does too.
To contextualize this a bit further, consider the photons of light. Light waves have frequencies that range vastly—from the lowly radio waves at a frequency of a few kilohertz to gamma rays that pulse at frequencies reaching thousands of petahertz. Such variations in frequencies contribute to the electromagnetic spectrum, influencing everything from communications to the colors we perceive.
Moreover, the energy of a photon directly affects how it interacts with matter. For instance, ultraviolet light photons carry more energy as compared to visible light photons, which explains why UV light can cause skin damage while visible light does not.
The interplay of frequency and energy forms the backbone of many quantum phenomena, revealing the fundamental nature of light and matter interactions.
The interplay of frequency and energy forms the backbone of many quantum phenomena, revealing the fundamental nature of light and matter interactions.
Calculating Quantum Frequency
While the relationship between frequency and energy is established, calculating quantum frequency involves insights from both theoretical and practical angles. To determine the frequency of a quantum state, one can rearrange the energy-frequency equation:
ν = E/h
In scenarios where the energy of a quantum system is known, the frequency can be deduced quite easily. This is particularly useful in fields like quantum optics and spectroscopy where understanding the frequency of transitions between quantum states informs us about molecular dynamics and behavior.
For example, in spectroscopy, atoms and molecules absorb or emit light at specific frequencies. By measuring these frequencies, scientists can deduce the atomic structure and electronic configurations of substances. This has significant implications in various applications, from identifying compounds in chemistry to exploring phenomena in astrophysics.
In practical calculations, various factors may be involved, such as thermal energy at certain temperatures or the influence of external fields. As such, quantum frequency calculations may have to integrate additional parameters depending on the experimental setup.
Experimental Insights
Experimental insights into quantum frequency are pivotal, as they bridge theoretical concepts with tangible observations in the laboratory setting. Understanding how quantum frequency manifests in experimental conditions not only enriches our comprehension of quantum mechanics but also demonstrates the practical implications of theory in real-world applications. The combination of experimental data and quantum theory enhances our ability to harness quantum phenomena for various technological uses, making this a crucial area of investigation.
Observations in Quantum Experiments
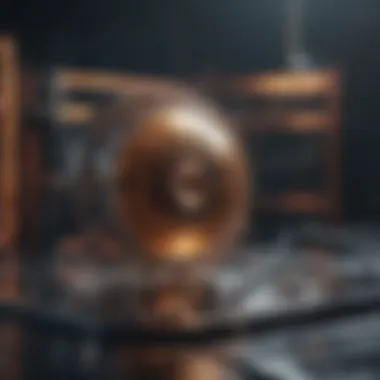
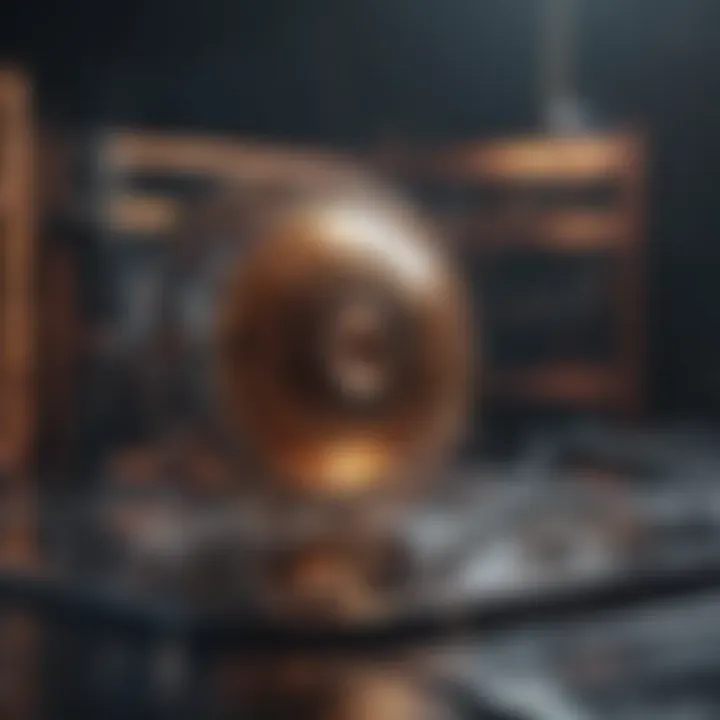
In the world of quantum mechanics, direct observations challenge many classical intuitions. Quantum experiments reveal a plethora of phenomena tied to quantum frequency, showcasing how particles behave in ways that defy everyday logic. One key observation is that particles such as electrons exhibit both wave-like and particle-like properties, depending on the measurement conditions.
These experiments often rely on sophisticated setups that can fine-tune parameters to observe frequency-related characteristics. The results from these observations have led to a deeper understanding of phenomena like superposition and entanglement. As each observation piles onto the last, scientists can begin to draw a more coherent picture of how quantum frequency operates on a foundational level.
Case Studies
Double-Slit Experiment
The Double-Slit Experiment remains a cornerstone of quantum physics, demonstrating the dual nature of matter through quantum frequency. What sets this experiment apart is its ability to show interference patterns created by particles when not observed, suggesting that particles exist in a superposition of states until measured. This characteristic provides profound insights into the principles of quantum mechanics, making it a celebrated choice for this article.
A unique feature of the Double-Slit Experiment is the transition from an observable wave-like pattern to discrete particles when measurement takes place. It highlights a key characteristic of quantum behavior: the idea that observation itself affects the state of the system. The advantages of employing this experiment in discussions of quantum frequency lie in its simplicity and visual impact, as it boldly illustrates foundational concepts in a way that is easily graspable by both students and seasoned researchers. However, its abstract nature might pose challenges for those more entrenched in classical views, as reconciling these outcomes with classical physics principles can be quite tricky.
Photoelectric Effect
The Photoelectric Effect serves as another seminal experiment that unlocks understanding around quantum frequency's role in energy transfer. When light of sufficient frequency strikes a material, it can eject electrons from that material. The crux of this effect is that it highlights the particle nature of light—specifically photons—and asserts that light frequency influences energy release, confirming the equation E = hf, where E is energy, h is Planck's constant, and f is frequency.
What makes the Photoelectric Effect particularly significant is its direct link to practical applications, especially in technology. This phenomenon is foundational for various devices such as solar panels and photodetectors. The interplay between frequency and energy offers a solid realization of theoretical principles in a physicist's toolkit. On the flip side, one potential disadvantage of this experiment in discussions around quantum frequency is that its technical aspects can become buried in equations and calculations, making it challenging for newcomers to fully appreciate its broader implications without guidance.
Applications of Quantum Frequency
Quantum frequency has immense significance across various fields, bridging the gap between fundamental physics and practical technology applications. From quantum computing to cryptography, the implications of quantum frequency extend well beyond theoretical discussions. These applications are not just theoretical constructs but are paving pathways for technological breakthroughs that can transform our world.
Quantum Computing
Quantum computing is perhaps the most talked-about application of quantum frequency. The core advantage of quantum technology lies in its ability to harness quantum bits, or qubits. Unlike classical bits, which exist in one state at a time, qubits exploit the principles of superposition and entanglement. Here, quantum frequency plays a crucial role in determining how qubits interact and operate.
- Superposition and frequency: As a qubit exists in multiple states simultaneously, the concept of quantum frequency helps in understanding how these states can be manipulated. If we consider multiple qubits together, their interaction can be optimized through specific frequency alignments, enhancing computational speed and efficiency.
- Quantum gates: These are fundamental for performing operations on qubits. Each gate's function can often be represented as a specific frequency interaction. Therefore, tuning the quantum frequency accordingly enables more complex operations, leading to advances in algorithm efficiency.
- Decoherence: The environment can affect qubit states, leading to errors in computation. Understanding quantum frequency's role helps in developing error-correction algorithms, which aim to preserve coherence over longer periods, critical for real-world applications.
The implications are profound, ranging from potentially solving problems intractable for classical computers to enabling advancements in artificial intelligence and complex modeling scenarios in various scientific disciplines.
Quantum Cryptography
On another front, quantum frequency finds a key role in quantum cryptography, which is poised to revolutionize secure communication protocols. Unlike classical encryption methods, which rely on mathematical complexity, quantum cryptography leverages the laws of quantum mechanics to secure data transmission.
- Quantum Key Distribution (QKD): This method ensures secure communication channels by employing quantum frequency. A crucial aspect is that any attempt at eavesdropping changes the frequency state of the qubits. This principle enables both parties to detect if their quantum states have been tampered with, effectively offering a level of security unattainable by classical means.
- Entangled particles: Frequency plays an essential part in entangled states where changes in one particle affect another, even when separated at large distances. This can be exploited to develop new cryptographic protocols offering highly secure transmission lines.
The perspective of quantum cryptography not only transforms the landscape of data security but also provokes thought on the ethical considerations of such powerful technologies, as they could redefine privacy in the digital age.
"As we peer into the applications of quantum frequency, we can see reflections of a future that reshapes our understanding of information, security, and computation."
"As we peer into the applications of quantum frequency, we can see reflections of a future that reshapes our understanding of information, security, and computation."
In summary, the applications of quantum frequency encompass both promising and challenging aspects of modern technology. The continuous exploration of these fields not only enhances our technical capabilities but also demands an ethical examination of their consequences in society.
Implications for Future Technology
Understanding the implications of quantum frequency on future technology is not just an exercise in speculation but a vital exploration of how emerging innovations can reshape industries. The intersection of quantum mechanics and technology holds the potential to unlock new frontiers, particularly in fields like communication, computation, and security.
Technological advancements driven by quantum frequency are poised to enhance the efficacy of various processes. For instance, consider how quantum frequency could improve information transmission. It allows for manipulation of photon states in ways that classical technologies cannot replicate, promising faster data rates and reduced latency. Quantum entanglement, a phenomenon deeply tied to quantum frequency, can enable instantaneous communication over vast distances. This is especially relevant in the development of next-generation internet systems that leverage quantum networks.
Additionally, quantum frequency plays a crucial role in enabling breakthroughs in sensor technologies. By understanding the precise frequencies at which quantum systems operate, researchers could design sensors that are sensitive enough to detect minute physical changes. This could lead to significant advancements in fields ranging from environmental monitoring to medical diagnostics.
"Quantum frequency is not merely a scientific curiosity; it could redefine how we interact with technology itself."
"Quantum frequency is not merely a scientific curiosity; it could redefine how we interact with technology itself."
Advancements in Communication Technologies
One of the most promising applications of quantum frequency lies in its impact on communication technologies. Traditional communication methods are limited by bandwidth and noise, which can affect the clarity and speed of data exchange. With the understanding of quantum frequency, we could enhance bandwidth capacity significantly and minimize signal degradation.
Some key advancements include:
- Quantum Key Distribution (QKD): Utilizing quantum frequency, QKD allows secure encrypted communication by detecting eavesdropping. Any attempt to intercept messages alters the quantum states being transmitted, alerting the parties involved.
- Entangled Photons for Communication: Techniques leveraging entangled photons can transmit information instantaneously, irrespective of distance. This could lead to a new era of secure, high-speed communication channels that are less susceptible to cyber-attacks.
- Quantum Repeaters: By bridging the gaps between distant quantum systems, quantum repeaters would use quantum frequency to bolster the range and effectiveness of quantum communication networks, making them more practical for real-world applications.
Challenges in Implementation
While the implications of quantum frequency in future technology are promising, several hurdles remain. One significant challenge is the difficulty in maintaining quantum states over time and distance. Quantum coherence can be disrupted by environmental conditions, leading to loss of information. This fragility poses obstacles in deploying quantum technologies on a large scale.
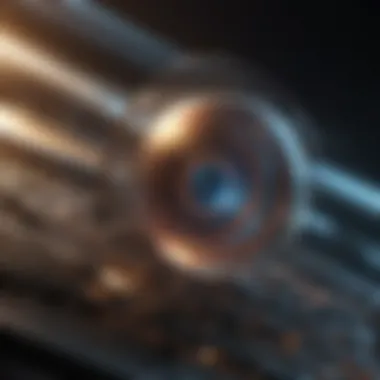
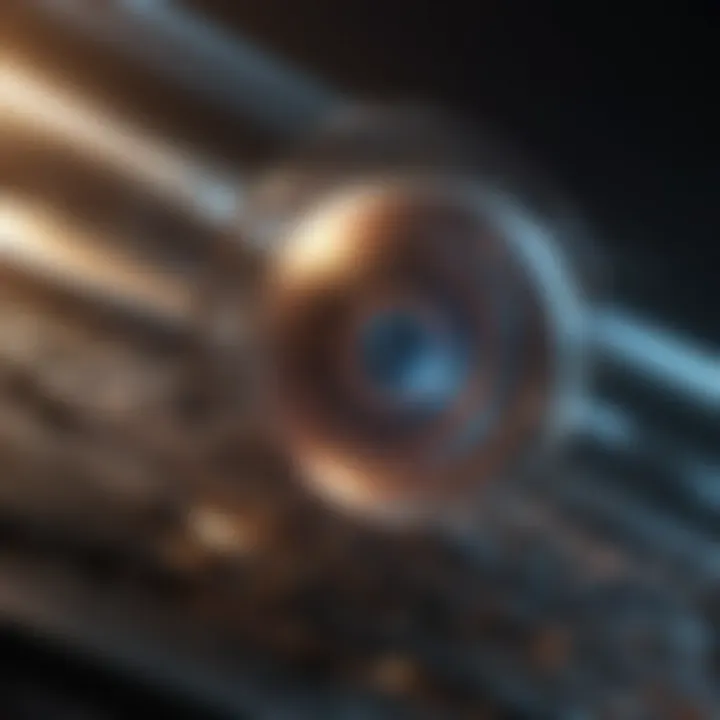
Moreover, the development of practical devices that can operate at quantum frequency requires significant resource investment. This leads to concerns about:
- Scalability: Many current quantum technologies are still in laboratory settings. Transitioning to commercial applications is not as straightforward as turning on a switch.
- Expertise Gap: As this field continues to evolve, there is a pressing need for trained professionals who can navigate both the scientific and engineering challenges associated with quantum frequency technology.
- Regulatory Concerns: With new technology comes the responsibility of addressing ethical and security issues. As quantum communication networks take shape, regulatory frameworks will need to adapt to the unique properties of quantum mechanics.
Quantum Frequency in Nature
Quantum frequency plays a fascinating role in understanding the natural world, intertwining with various phenomena that govern both microscopic and macroscopic systems. As a cornerstone of quantum mechanics, this concept delves into the vibrational patterns inherent to particles, influencing everything from the behavior of atoms to the intricacies of life itself. By studying quantum frequency, researchers unlock windows into the ways energy is exchanged and utilized in our universe, revealing its significance in both physical and biological contexts.
Role in Spectroscopy
Spectroscopy is one of the key applications of quantum frequency, enabling scientists to probe the interactions between light and matter. Essentially, spectroscopy involves studying the spectrum of light emitted or absorbed by substances, providing critical insights into their composition, structure, and dynamics. Here, quantum frequency acts as a bridge between energy levels in quantum systems and the corresponding electromagnetic radiation.
When light interacts with an atom or molecule, quantized energy levels dictate how the substance will absorb or emit photons. Different elements and compounds have unique electromagnetic signatures based on their quantum states, which can be observed through spectroscopy. For instance, when a molecule transitions from an excited state to a lower energy state, the quantum frequency of the emitted light reveals essential details about that molecule's identity and environment.
Key points to consider in spectroscopy include:
- Energy Gaps: The differences between energy levels, critically dependent on quantum frequency, determine the specific wavelengths of light absorbed or emitted.
- Applications in Chemisty: Spectroscopy aids in identifying substances and understanding chemical reactions, pivotal in drug development and environmental monitoring.
- Astronomical Insights: By applying spectroscopy to light from distant stars, astronomers glean information about cosmic chemical compositions and phenomena.
"Spectroscopy is like looking through a window into the molecular world, with quantum frequency as the key to deciphering the messages within attention-grabbing light."
"Spectroscopy is like looking through a window into the molecular world, with quantum frequency as the key to deciphering the messages within attention-grabbing light."
Connections to Biological Systems
The implications of quantum frequency extend into the realm of biology, revealing connections that may seem distant at first glance, but are in fact deeply rooted in the physical laws governing matter. A remarkable example is the process of photosynthesis, wherein plants harness light energy to produce food. Here, the quantum frequency of incoming sunlight determines how efficiently a plant can convert light into chemical energy.
This mechanism prompts several considerations:
- Efficiency in Energy Conversion: The unique properties of quantum frequency enable certain pigments, like chlorophyll, to absorb specific light wavelengths effectively. This is where quantum effects, such as coherence and entanglement, may enhance energy transfer processes, possibly leading to innovations in synthetic photosynthesis.
- Quantum Biology: A growing field highlights that the principles of quantum mechanics might govern biological phenomena such as bird navigation, where birds utilize quantum entanglement for orientation based on Earth’s magnetic field.
In summary, the study of quantum frequency in nature reveals its multi-faceted influence across various domains, from the precise characterizations of materials in laboratories to the subtle and complex mechanisms operating in living organisms. This exploration not only deepens our appreciation for quantum theory but also encourages ongoing inquiry into how nature orchestrates such remarkable phenomena.
Challenges in Quantum Frequency Research
Understanding the challenges associated with quantum frequency research is pivotal, as it shapes both the present and future of this complex field. Researchers often find themselves at a crossroads, wrestling with the implications of quantum mechanics in practical applications. There are a multitude of factors at play that can impede progress, ranging from theoretical limitations to experimental constraints.
Limitations of Current Theories
Despite the significant advancements made in quantum mechanics, the theories we possess today still exhibit notable shortcomings. For instance, when examining the quantum frequency of particles, existing models may struggle to accurately incorporate the peculiarities of wave-function behavior when scaled to macro levels. This discrepancy raises a fundamental question: how can we reconcile quantum phenomena with everyday experiences?
In addition to theoretical inadequacies, ambiguities often arise around the mathematical representation of quantum frequency. The current equations may lack robustness in certain extreme conditions or anomalies, resulting in predictions that do not always align with empirical data. This dissonance complicates efforts to develop quantum technologies that rely on precise measurements of frequency. If the mathematical models cannot capture the nuance of quantum behavior, how can engineers and scientists design effective quantum devices?
Moreover, the leap from theory to application presents its own set of hurdles. Quantum entanglement, a cornerstone of quantum frequency, has proven extremely difficult to harness in practical scenarios. The complications in manipulating entangled states restrict advancements in fields such as quantum computing and cryptography, where the reliable use of quantum frequency is essential.
Future Directions in Research
Looking ahead, the path of quantum frequency research holds promise but is fraught with challenges that will demand innovative thinking and interdisciplinary collaboration. One possible direction involves refining our current theories to enhance their explanatory power. This may mean developing new mathematical frameworks that can better account for observed phenomena, thereby bridging the gap between theoretical predictions and practical outcomes.
Another avenue worth exploration is the integration of machine learning techniques with quantum research. These technologies could help in modeling quantum systems more accurately, allowing for deeper insights and the development of new algorithms that can optimize quantum frequency applications in a more adaptive manner.
Additionally, researchers may benefit from an increased emphasis on collaborative experimental studies. By pooling resources and expertise, scientific teams could tackle complex quantum problems through shared knowledge and innovative testing approaches. This collective effort could lead to breakthroughs in understanding quantum systems, particularly in real-world implementations.
"The quest for understanding quantum frequency is as much about unraveling the microscopic mysteries of our universe as it is about shaping the technologies of tomorrow."
"The quest for understanding quantum frequency is as much about unraveling the microscopic mysteries of our universe as it is about shaping the technologies of tomorrow."
In summary, while challenges in quantum frequency research loom large, they also present an array of exciting opportunities to rethink current models and practices. By confronting these limitations head-on, the scientific community can forge pathways that enhance both our comprehension of quantum phenomena and the practical applications to which they give rise.
End: The Significance of Quantum Frequency
As we tie up our exploration into quantum frequency, it becomes clear that this concept is not just a puzzling aspect of quantum mechanics, but a cornerstone of modern physics and technology. The significance of quantum frequency lies in its ability to bridge various disciplines—from communication technologies to biological systems.
Key Benefits and Considerations
- Foundation of Quantum Technologies
Quantum frequency serves as the bedrock for innovations like quantum computing and cryptography. These technologies rely on the manipulation of quantum states governed by frequency, impacting industries beyond just tech. - Insights into Nature
Understanding quantum frequency enhances our comprehension of natural phenomena, such as the vibrational modes in molecules. Spectroscopy, for instance, leverages these frequencies to identify material composition. This application is vital in fields like chemistry and environmental science. - Interdisciplinary Nature
The implications of quantum frequency extend far beyond physics. By intertwining with biology, we uncover potential links to how living organisms utilize quantum mechanics for processes like photosynthesis. This coupling of disciplines has the potential to inspire future research directions.
"The interplay between quantum frequency and matter nudges the boundaries of our current understanding, opening doors to fascinating possibilities."
"The interplay between quantum frequency and matter nudges the boundaries of our current understanding, opening doors to fascinating possibilities."
Future Perspectives
As we look forward, challenges remain. Current theories struggle with fully explaining the complexities of quantum frequency in various systems. Yet, this serves as a catalyst for inquiry. Researchers must delve deeper, using advancements in experimental methods to unlock new dimensions of this concept. Bridging the gap between theoretical knowledge and practical applications is where the real magic happens.
In summary, quantum frequency is not merely another aspect of quantum mechanics; it is a vital piece of the puzzle. Its significance spans a wide array of fields, suggesting that our comprehension of quantum frequency influences technological advancements and scientific understanding alike. Embracing this knowledge invites us to ponder and explore further, illuminating an intricate tapestry where theory meets real-world application.