Understanding Zeta Potential Measurement with DLS
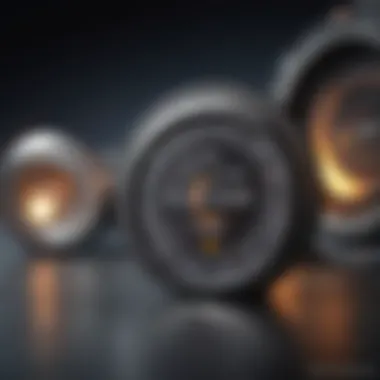
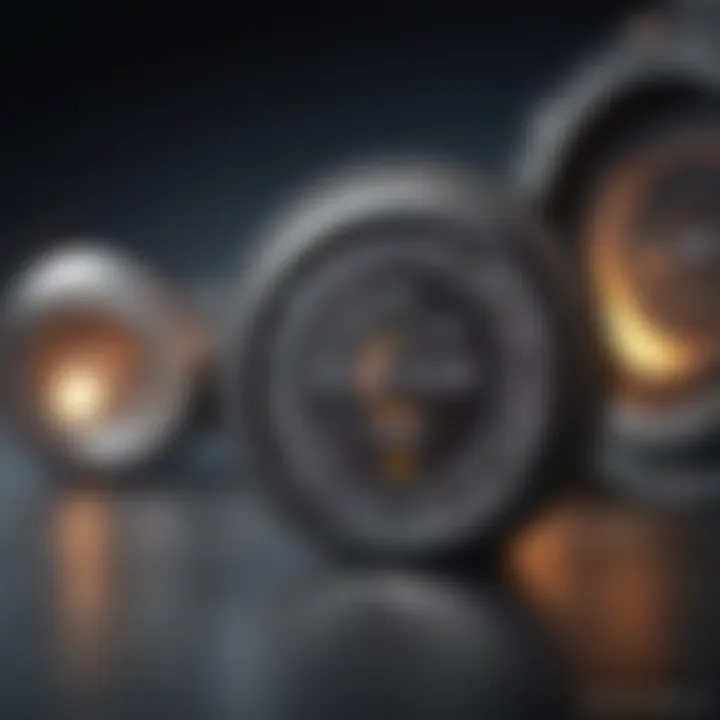
Intro
In the world of scientific exploration, measuring zeta potential carries profound significance. It acts as a barometer for stability in colloidal systems, which are essentially mixtures where tiny particles are dispersed in a liquid. This determination of zeta potential is crucial for a variety of scientific and industrial applications, from pharmaceuticals to environmental engineering. By employing dynamic light scattering—a powerful technique that can provide insights into particle interactions—and understanding the underlying principles, one can navigate the intricate landscape of colloidal chemistry effectively.
Key Concepts
Definition of the Main Idea
Zeta potential refers to the electrical potential at the slipping plane of a particle in a colloid. In simpler terms, it’s a measure of the charge that a particle possesses in a liquid medium. This charge influences how particles interact with each other, affecting their stability in suspension.
Overview of Scientific Principles
Understanding the science behind zeta potential involves delving into colloidal stability, electrokinetics, and the interaction forces at play. When particles within a suspension possess sufficient zeta potential—typically greater than ±30 mV—they tend to remain well-dispersed, indicating good stability. However, lower values can lead to agglomeration, which often results in unwanted sedimentation and can greatly impact the efficacy of a product, especially in pharmaceuticals.
Zeta potential is influenced by factors such as pH, ionic strength, and the composition of the surrounding medium. These parameters can drastically swayed the interactions between dispersed particles, making the precise measurement of zeta potential not only scientifically intriguing but also industrially vital.
Current Research Trends
Recent Studies and Findings
Current research trends emphasize the advancement of dynamic light scattering techniques used in zeta potential measurement. Recent studies have shown that improvements in DLS instrumentation lead to more accurate readings, enhancing the understanding of small-scale interactions. Furthermore, the exploration of alternative methods—like microelectrophoresis—has gained traction, offering complementary insights into particle behavior in various environments.
Significant Breakthroughs in the Field
Major breakthroughs have also been noted in areas such as nanotechnology and drug delivery, where precise control of particle stability is paramount. The advancements in DLS instruments, including the incorporation of high-speed cameras and sophisticated algorithms, have improved the detection of particle movements and allowed for real-time analysis of zeta potential. This progress not only enhances fundamental research but also promises to tackle real-world challenges, such as formulating stable suspensions for medication delivery.
"Accurate zeta potential measurements are essential for tailoring formulations in various industries, from pharmaceuticals to nanotechnology, ensuring that products perform as intended in real-world applications."
"Accurate zeta potential measurements are essential for tailoring formulations in various industries, from pharmaceuticals to nanotechnology, ensuring that products perform as intended in real-world applications."
With this foundational understanding now established, the article will further explore the methodologies employed in DLS, elaborate on the implications of zeta potential in practical scenarios, and guide readers through the nuances of interpreting results in various contexts.
Prologue to Zeta Potential
Zeta potential plays a vital role in the behavior of colloidal systems. It serves as a significant indicator of the stability of colloidal dispersions, influencing various applications ranging from pharmaceuticals to environmental science. Understanding this concept opens doors to enhancing product formulations, optimizing processes, and ensuring quality control within industries.
For researchers, grasping zeta potential assists in tailoring specific properties of formulations, ensuring desired interaction between particles. For students and educators, introducing zeta potential enriches the curriculum with practical insights into real-world applications.
Defining Zeta Potential
At its core, zeta potential refers to the electric potential at the slipping plane of a particle immersed in a liquid medium. It essentially reflects the balance of electrostatic forces acting on particles within the dispersion. When particles are dispersed in a fluid, they acquire an electrical charge—this charge leads to the creation of a double layer of ions around each particle that influences its stability within the medium.
The measurement of zeta potential provides insights into how particles interact under varying conditions, which is crucial in fields like drug delivery and material science. In simple terms, if the zeta potential is high—whether positive or negative—it indicates strong repulsive forces between particles, promoting stability. Conversely, low zeta potential values signal a higher likelihood of particle aggregation, which is often undesirable.
Historical Context and Development
The journey of zeta potential measurement is not a recent phenomenon; it has evolved over decades. The groundwork was laid in the early 20th century when scientists like Smoluchowski and Einstein made significant contributions to understanding colloidal systems. They explored the relationship between particle size, charge, and mobility, providing the foundation for later advancements.
As technology progressed, researchers developed more sophisticated methods to measure zeta potential. Initially limited to simple electrophoretic techniques, advances in instrumentation such as dynamic light scattering have revolutionized our approach. Today, scientists can accurately assess zeta potential with greater ease and precision, a far cry from earlier methods that were often cumbersome and resource-intensive.
This evolution not only reflects the advancement of technology but also the growing recognition of the importance of zeta potential across various scientific disciplines. Understanding past developments allows researchers to appreciate current techniques and anticipate future innovations.
Theoretical Foundations
Understanding the theoretical framework surrounding zeta potential is pivotal for grasping how dynamic light scattering (DLS) methods measure this important parameter. The theoretical foundations set the stage for practical applications by explaining the principles that govern the electrokinetic behavior of colloidal particles. These principles enhance our ability to interpret measurement results, guiding scientists and engineers in their respective fields.
Electrokinetic Phenomena
Electrokinetics is the study of the motion of dispersed particles under the influence of an electric field. This motion is closely tied to zeta potential, which is defined as the electrical potential at the shear plane of a particle in a fluid. The significance of electrokinetic phenomena cannot be overstated, as it influences how particles interact in various environments, particularly in colloidal systems.
When colloidal particles are suspended in a medium, they experience forces that drive them to either aggregate or remain dispersed. This stability is heavily influenced by the zeta potential. A higher magnitude of zeta potential typically indicates better colloidal stability, as particles are more likely to repel each other and avoid flocculation.
"Electrokinetic phenomena highlight the delicate balance between attractive and repulsive forces acting on particles, which is crucial for stability in colloidal systems."
"Electrokinetic phenomena highlight the delicate balance between attractive and repulsive forces acting on particles, which is crucial for stability in colloidal systems."
One key point to consider is the relation between zeta potential and the movement of particles in response to an electric field. This movement, whether it be electrophoresis (motion under an electric field) or sedimentation, informs us about the charge and size of the particles involved. For instance, when a colloid is subjected to an electric field, the speed at which particles migrate gives insight into their zeta potential, leading to a better understanding of their behavior in various applications such as drug delivery or wastewater treatment.
Understanding Charge Distribution in Colloids
Charge distribution within colloids is another integral topic under the theoretical foundations of zeta potential measurement. It directly affects the stability of colloidal systems and their interactions with external influences such as pH and ionic strength. Every particle in a colloidal suspension possesses a surface charge, and this charge plays a critical role in determining the overall stability and behavior of the particles.
The distribution of charge is influenced by several factors. Firstly, the nature of the particle surface—whether it is hydrophobic, hydrophilic, or charged—can alter how ions from the surrounding medium interact with it. For example, if particles have an affinity for water (i.e., they are hydrophilic), they will tend to attract ions, leading to different electrokinetic behavior compared to hydrophobic particles.
To deepen understanding, one could examine how parameters like pH levels and ionic strength affect charge distribution.
- At low pH, the concentration of hydrogen ions increases, leading to more positive charges on certain particle surfaces.
- At high pH, these same particles might gain negative charges, resulting in varying zeta potential readings.
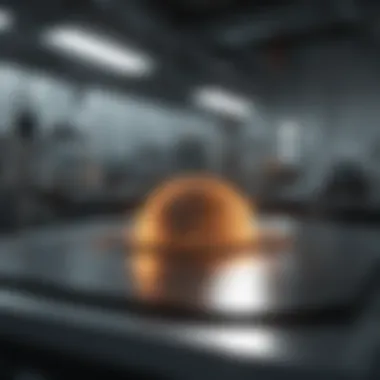
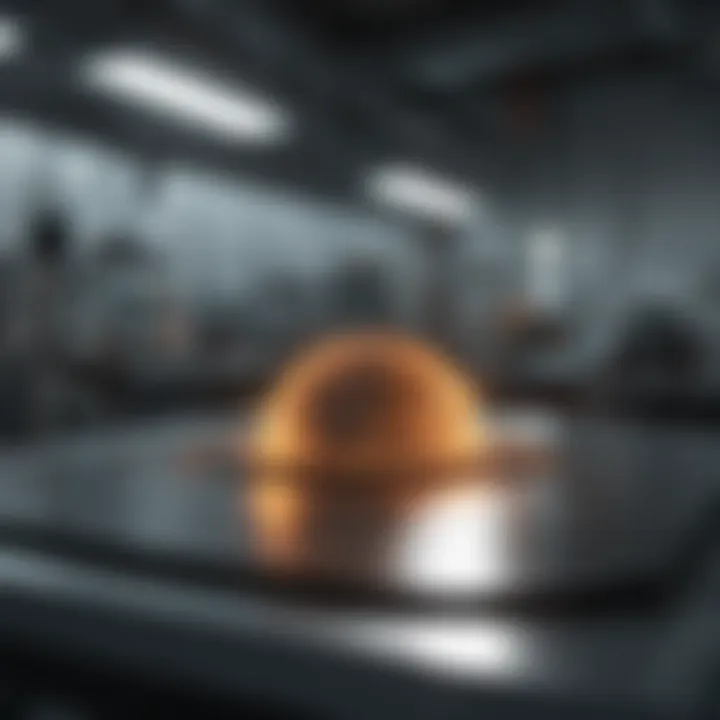
Considering these aspects allows researchers and practitioners to manipulate conditions for optimal performance in applications like drug formulation, where understanding how specific formulations behave at different pH levels is vital.
In essence, grasping the theoretical foundations of electrokinetic phenomena and charge distribution allows for a richer interpretation of zeta potential results, facilitating advancements in various scientific and industrial fields.
Dynamic Light Scattering: An Overview
Dynamic Light Scattering (DLS) represents a critical avenue in the measurement of zeta potential, particularly owing to its capacity to provide real-time analysis of particle dynamics within suspension. By gauging how the light scatters as it interacts with movable particles, DLS not only yields zeta potential metrics but also opens pathways into understanding the behavior of colloidal systems under varying conditions. This section will shed light on the principles behind DLS measurement, the instrumental setup required, and the nuances involved in its execution.
Principles of DLS Measurement
The heart of DLS rests on the principle of Brownian motion. When a laser beam hits a colloidal sample, the scattered light reflects the movements of the particles within that sample. The fluctuations in intensity of the scattered light are analyzed to obtain meaningful information regarding particle size and zeta potential. As particles disperse throughout the solution, they exhibit different speeds. Faster-moving particles will cause rapid fluctuations in the light intensity and produce a faster decay rate in correlation with their size and charge.
A key advantage of utilizing DLS for measuring zeta potential is its precision in assessing not just size distribution but also the electrical double layer surrounding particles. As zeta potential is intricately linked to particle stability in a colloidal medium, such data contributes profoundly to comprehending systems ranging from pharmaceuticals to environmental science.
Instrumentation for DLS
DLS instrumentation typically comprises several integral components, each serving an essential role in delivering reliable measurements.
Laser Sources
For DLS, laser sources are paramount. These lasers provide a coherent light source that facilitates effective scattering from particles. A common choice is the He-Ne laser, known for its stability and ease of operation.
A significant characteristic of laser sources is their wavelength, generally either in the visible spectrum or infrared range. This features crucial importance because the light's wavelength affects resolution and sensitivity. Lasers with shorter wavelengths can deliver more precise measurements, which is particularly beneficial when working with nanosized particles that are characteristic of many contemporary scientific endeavors.
However, one downside is that certain materials may absorb specific wavelengths disproportionately, thereby leading to erroneous measurements. It's thus vital to choose a laser that aligns with the properties of the sample.
Detectors
Detectors are another critical component of DLS systems, as they capture the scattered light and convert it into an electrical signal. Commonly used detectors like avalanche photodiodes (APDs) offer high sensitivity and rapid response times, thus allowing them to register fluctuations in particle movement with precision.
The key characteristic of detectors lies in their ability to differentiate between light intensity changes at different angles. This is predominantly beneficial as it enables the measurement of a broader size distribution of particles.
Still, careful calibration of the detection angle is essential because it can significantly impact the measured zeta potential values. Inaccurate angles can lead to misleading results, underscoring the need for practitioners to pay close attention during the setup.
Sample Cells
The choice of sample cells also warrants attention, as they hold the colloidal samples for measurement. Typically crafted from transparent materials like quartz or glass, these cells must be free from contamination and allow the passage of laser light without distortion.
A distinct feature of contemporary sample cells is their ability to accommodate small volumes, making DLS suitable for studies requiring limited sample quantities. This is not only economical but also conserves precious substances often used in research.
However, the geometry and cleanliness of these cells play an essential role. Impurities or irregular shapes can interfere with light scattering measurements, ultimately leading to compromised data integrity. Therefore, ensuring a high level of cleanliness is paramount.
"In the world of colloidal research, precision is not just beneficial; it is mandatory. One stray particle can alter the meaning of an entire dataset."
"In the world of colloidal research, precision is not just beneficial; it is mandatory. One stray particle can alter the meaning of an entire dataset."
Through a solid grasp of these principles and instrumental elements, researchers can effectively leverage DLS techniques in the measurement of zeta potential, unlocking insights that transcend traditional methodologies.
Practical Procedure for Zeta Potential Measurement
Getting the zeta potential measurement right involves much more than just flipping a switch on the device. The entire process is a careful dance of precision, and each step plays an important role to ensure that you get accurate, reliable data. This section aims to highlight the critical facets of the practical procedure, setting the stage for understanding what it entails. Having a solid grasp of the practical procedure not only enhances the quality of your measurements but also feeds into the interpretation and the insight you can derive from zeta potential data.
Sample Preparation Techniques
In any experimental setup, sample preparation can make or break your results. When measuring zeta potential using dynamic light scattering, achieving a uniform sample is paramount. Ideally, you want a homogenous dispersion of colloidal particles. Any agglomeration can skew your measurements.
When preparing your sample, consider the following:
- Concentration: Start with a balanced concentration. Too few particles don't provide a clear signal, while too many can lead to scattering artifacts.
- Medium Compatibility: Ensure that the sample medium is appropriate. Water might not always be the right choice; sometimes, a buffer solution is needed to maintain pH and ionic strength.
- Mixing Technique: Utilize gentle mixing to avoid bubble formation, which can interfere with light scattering.
It’s also a good idea to filter your sample prior to measurement. This removes any larger particles and debris. Think of it as cleaning your shoes before entering a freshly polished hallway—every little bit helps.
Calibration and Setting Parameters
Before jumping into measurements, calibration confirms that your equipment works correctly. It’s akin to tuning a musical instrument before performance. Without calibration, discrepancies and errors can lead to misleading zeta potential values. Here’s how to go about it:
- Standard Calibration: Use a known standard, like a latex suspension, to check if your device is delivering reliable results.
- Temperature Control: Remember that temperature can affect the mobility of particles. Make sure that your samples and the equipment are aligned in terms of temperature.
- Setting the Appropriate Parameters: Adjust laser intensity and detection angles. Each setup can be unique, and sometimes it’s necessary to tweak things just a bit to achieve the best signal quality.
- Repetitive Checks: After initial calibration, periodically check the device's performance to ensure it hasn’t drifted.
With these steps, your measurements should now be primed and ready to go, much like a race car waiting for the green light.
Conducting the Measurement
Finally, it’s time to hit the ground running with the actual measurement.
Ensure the following steps are taken:
- Sample Injection: Do this cautiously. Depending on whether you’re using a capillary cell or a batch cell, tailor your approach accordingly. Too fast an injection may introduce air bubbles.
- Measurement Duration: Monitor the time for each measurement. It can vary based on concentration but generally lasts between 1 and 10 minutes.
- Repeatability: Take multiple readings for accuracy. This ensures data reliability and helps in identifying inconsistencies.
As you progress with your measurements, remember – the devil is in the details. Attention to even the smallest aspects can significantly affect your results.
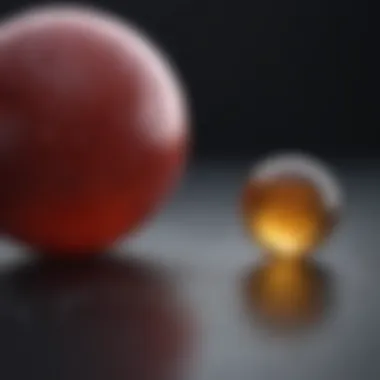
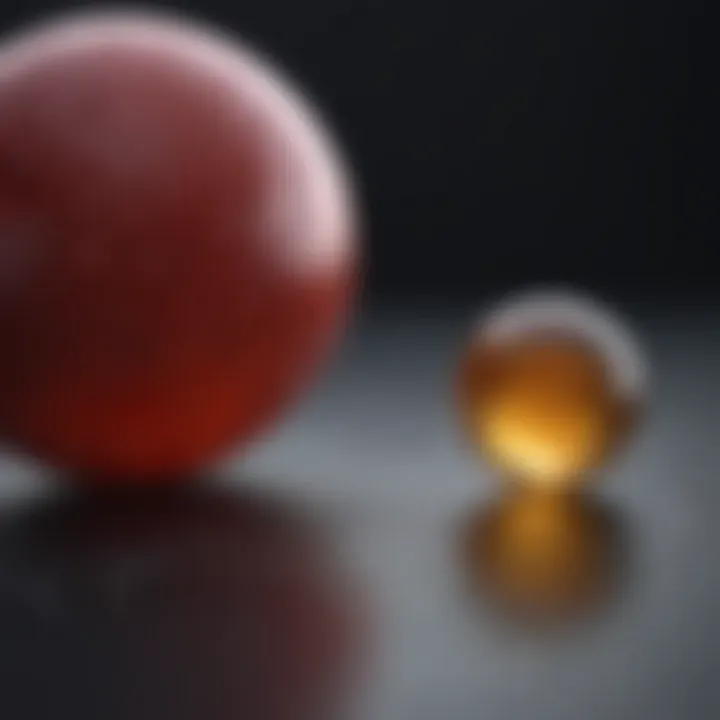
As you progress with your measurements, remember – the devil is in the details. Attention to even the smallest aspects can significantly affect your results.
The successful execution of these procedures can lead to clearer insights from your zeta potential values, significantly boosting the understanding of the colloidal systems you’re studying. Thus, every step from preparation to measurement matters more than it seems.
Interpreting Zeta Potential Results
Understanding the zeta potential is a key aspect when one is measuring and interpreting results obtained from dynamic light scattering. Zeta potential serves as an indicator of the stability of colloidal systems, playing a crucial role in various fields, from pharmaceuticals to environmental sciences. When one seeks to interpret these results, several specific elements come into play that shed light on the nuances of the measurements.
Significance of Zeta Potential Values
The measurement of zeta potential can reveal important information about the stability of a colloidal system. Generally, a high zeta potential (either positive or negative) indicates that the particles repel each other strongly and thus are more likely to remain dispersed. This is particularly valuable in formulating stable suspensions or emulsions. Conversely, a low zeta potential suggests weak repulsive forces, which may lead to aggregation or sedimentation of the particles due to van der Waals forces taking the upper hand.
Additionally, the interpretation of zeta potential values helps in assessing the quality and suitability of substances for specific applications. For example:
- Colloidal Stability: Understanding how stable a colloidal dispersion is aids in predicting its behavior in various applications.
- Product Shelf Life: Knowing the zeta potential can inform product formulators about how long their products may remain usable before integrity is compromised.
- Compatibility Issues: In formulations with multiple components, zeta potential can help predict whether certain materials will interact favorably or lead to issues.
In summation, the contribution of zeta potential values to the field is multi-faceted and essential for ensuring the efficacy and reliability of products.
Factors Influencing Zeta Potential Measurements
When interpreting zeta potential, it’s imperative to consider the various environmental and experimental factors that influence these measurements. Two of the most significant are pH and ionic strength, along with temperature effects.
pH and Ionic Strength
The pH of a solution significantly affects the surface charge on particles, subsequently influencing their zeta potential. Each material has a point of zero charge (PZC) where the zeta potential is neutral. Above the PZC, the particles tend to carry a negative charge, while below it, they carry a positive charge. Consequently, controlling pH adds a beneficial dimension to the stability of colloids. However, it also presents challenges as drastic pH changes can lead to flocculation, negatively impacting the dispersion.
The ionic strength also plays a critical role as it can screen the electrostatic interactions between charged particles. Increased ionic strength reduces the zeta potential, often leading to decreased stability. Hence, a careful balance must be maintained to find the optimal conditions conducive for a specific application, making this a powerful yet delicate tool in colloid chemistry.
Temperature Effects
Temperature significantly influences zeta potential measurements by altering the kinetic energy of the particles. Higher temperatures tend to increase the mobility of the particles, which can lead to a decrease in measured zeta potential due to less pronounced electrostatic interactions.
Thus, while conducting experiments, maintaining a consistent temperature is of utmost importance to ensure reproducibility and accuracy. A unique aspect of measuring zeta potential at varying temperatures is the ability to study the thermal stability of colloidal systems. However, the inconsistency that temperature fluctuations can introduce also adds a layer of complexity to interpretations and must be monitored closely.
Understanding how these factors interplay enhances the clarity of results obtained, offering a pathway toward robust and reliable outcomes in colloidal studies.
"The ability to interpret zeta potential accurately paves the way for innovative solutions in both research and industry."
"The ability to interpret zeta potential accurately paves the way for innovative solutions in both research and industry."
Therefore, by taking these factors into consideration, one can develop a well-rounded approach to understanding and utilizing zeta potential measurements effectively.
Applications of Zeta Potential Measurement
The relevance of zeta potential measurement spans several disciplines, making it a cornerstone in both scientific inquiry and industrial applications. Understanding how zeta potential impacts various systems plays a pivotal role in enhancing performance and stability, particularly in colloidal formulations. In essence, zeta potential serves as an indicator of stability in suspensions and emulsions, reflecting the electrostatic repulsion between particles in a liquid medium. Grasping this concept allows researchers and industry professionals to finely tune formulations to achieve desired characteristics, from drug delivery systems to paints and coatings.
Colloidal Stability in Pharmaceuticals
In the pharmaceutical sector, maintaining the stability of colloidal systems is not just advantageous; it is crucial. Zeta potential measurements shed light on the stability of suspensions, providing insights into how particles interact in a solution. A higher absolute zeta potential indicates a greater repulsive force, which typically leads to enhanced stability of pharmaceutical formulations.
This is particularly significant for injectable drugs and oral suspensions, where uniform particle distribution can impact bioavailability and therapeutic efficacy.
- Key Benefits:
- Prevents sedimentation and aggregation.
- Ensures consistent drug dosage.
- Informs formulation adjustments for improved efficacy.
For instance, when scientists are developing a new formulation of a nanoparticle-based drug, assessing the zeta potential can guide the selection of stabilizers and surfactants that enhance dispersion stability. Any fluctuation in this parameter may necessitate reformulation.
Environmental Science Applications
The applications of zeta potential measurements extend beyond the laboratory and into the realm of environmental science. For water treatment processes, understanding zeta potential can help predict the behavior of contaminants and enable more effective methods for purification.
Monitoring the charge of particles in solution provides valuable information about their propensity to agglomerate or remain suspended, which is fundamental for developing techniques to remove harmful substances from wastewater.
- Considerations:
- Influence of pH and ionic strength on zeta potential.
- The impact of natural organic matter on particle behavior.
In situations where sediments accumulate in natural water bodies, understanding these interactions can facilitate better strategies for sediment management and overall ecosystem health. Essentially, zeta potential measurements can aid in designing more effective filtration and treatment systems, thereby fostering cleaner water sources for communities.
Industrial Implications in Coatings and Dispersions
In the industrial landscape, particularly in coatings and dispersions, zeta potential plays a significant role in determining product performance. Coatings must adhere properly to surfaces without running the risk of separation or clumping during application. By measuring zeta potential, manufacturers can optimize formulations to ensure that individual particles remain adequately dispersed in the binder medium.
- Key Advantages:
- Enhances performance and longevity of coatings.
- Reduces problems associated with aggregation during mixing.
- Informs quality control processes by monitoring changes in formulations.
Additionally, zeta potential measurement can guide the selection of appropriate surfactants for achieving stability. The correct surfactant influences the dispersion of pigments or other solid components, leading to a uniform coating that exhibits superior aesthetic and functional properties.
Understanding the relationship between zeta potential and stability is crucial for industries where consistent quality matters.
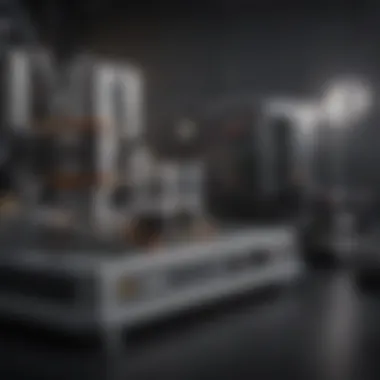
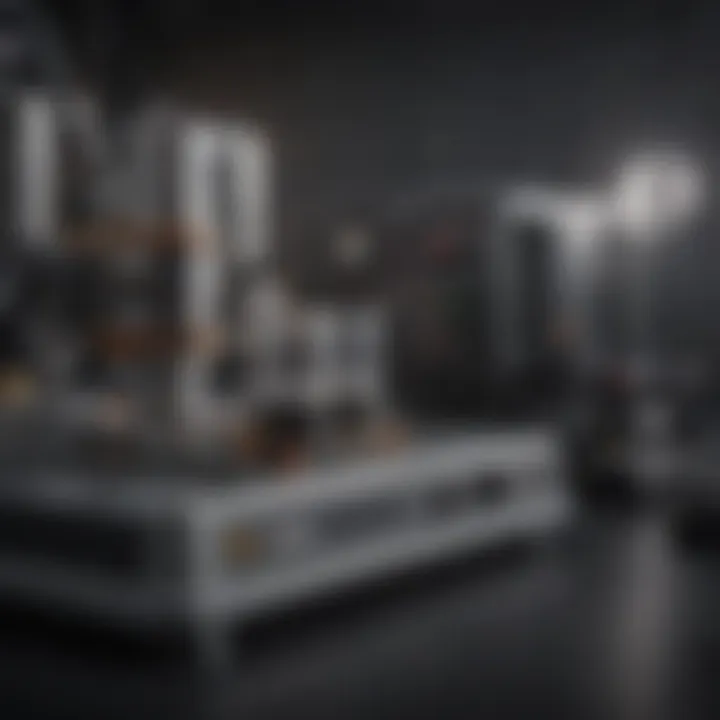
Understanding the relationship between zeta potential and stability is crucial for industries where consistent quality matters.
Limitations and Challenges
Understanding the limitations and challenges in zeta potential measurement is crucial, particularly when employing dynamic light scattering (DLS) techniques. Accurate zeta potential measurement plays a vital role in various fields, from pharmaceuticals to environmental science, but it isn’t without its hurdles. The complexities involved in obtaining precise measurements can sometimes muddy the waters, leading to misinterpretations and potentially flawed outcomes. This section dives into the common sources of error in DLS measurement and interpretational challenges, highlighting the significance of these issues in a comprehensive analysis.
Common Sources of Error in DLS Measurement
Dynamic light scattering, while powerful, is not impervious to errors, and knowing the potential pitfalls is the first step toward effective measurement. Here are some common sources of error:
- Instrumental Noise: Like a pesky fly at a picnic, noise from the equipment can impact data. Factors such as detector sensitivity and laser stability can introduce variability. If the instruments aren’t calibrated correctly, the zeta potential measurements can go off-track
- Sample Quality: The old saying "garbage in, garbage out" holds true. Poorly prepared samples, whether due to contamination or inadequate dispersal, will yield unreliable results. Having uniform colloidal samples is paramount.
- Analysis Algorithms and Settings: Different algorithms process data in varied ways. If settings are not optimized for the sample at hand, there might be discrepancies in the output. Misapplication of parameters can lead to skewed analyses.
- Environmental Factors: Conditions such as temperature and airflow can create havoc on measurements. Dyeing lights and shifty conditions can forge a path to inconsistent readings
"An aware practitioner is the best practitioner!"
"An aware practitioner is the best practitioner!"
Recognizing and addressing these sources of error enhances the robustness of measurements and the reliability of the results.
Interpretational Challenges
Even when measurements are obtained accurately, interpreting the results poses its own set of challenges. It's akin to trying to read a map while driving on a foggy road. Some key interpretational challenges include:
- The Misunderstanding of Zeta Potential Values: A zeta potential value appears as a numeric output, but it’s a lot like an iceberg; a significant amount lies beneath the surface. Variations in methodology can yield differing insights, leading to misunderstandings about stability and interactions
- Interdependence of Factors: Zeta potential doesn’t exist in a vacuum. External elements like pH, ionic strength, and temperature can alter readings. Disentangling these interdependencies can be tricky, akin to solving a jigsaw puzzle with not all the pieces in sight
- Relating Values to Practical Outcomes: Translating zeta potential data into practical applications can create confusion. For instance, what looks stable on paper may not hold true in actual conditions. A careful and thorough approach to correlation is necessary to ensure that conclusions drawn from the data are sound
- Complexities in Colloid Systems: Colloidal systems are frequently more intricate than they seem. Diverse particle types, sizes, and shapes can create varying effects on the observed zeta potential. Assessing the overall stability and behavior of colloids requires a multidimensional understanding.
Recent Advances in Zeta Potential Measurement Techniques
The realm of zeta potential measurement has seen significant evolution in recent times, primarily driven by innovative technologies and the ever-growing need for precision. With Zeta potential acting as a crucial indicator of colloidal stability and behavior, understanding these recent advances holds great relevance not only for the scientific community but also for industries where stability and functionality of dispersed systems matter. This section will focus on two main elements: the integration of nanotechnology and emerging sensing technologies, both of which are reshaping how zeta potential is measured and interpreted.
Integration of Nanotechnology
In the world of coloidal chemistry, the recent adoption of nanotechnology has ushered in a new era of zeta potential measurement. By harnessing the unique properties of nanoparticles, researchers now can achieve more precise measurements across various mediums. In conventional methods, zeta potential determination can suffer from limitations related to sample size and purity. However, the integration of engineered nanoparticles can provide more robust systems that enhance measurement accuracy.
For instance, employing nanostructured electrodes in conjunction with DLS has been shown to significantly reduce noise and improve the signal-to-noise ratio. These advancements offer numerous benefits:
- Enhanced Sensitivity: Nanotechnology allows for the detection of changes in zeta potential with a level of sensitivity previously unattainable. This is particularly valuable in monitoring drug formulations or wastewater treatments where slight variations can indicate larger trends.
- Miniaturization of Equipment: As a result of nanotechnology, measuring instruments can become smaller yet more powerful, making them more accessible and easier to use in field applications. This addresses the ongoing challenge of needing in-situ measurement tools, especially in dynamic environments.
- Improved Control of Aggregation: Tailoring nanoparticle surfaces can help control impulse aggregation, which is crucial when working with colloids. Understanding how nanoparticles influence zeta potential can lead to better stability predictions and formulations in pharmaceuticals, for example.
Emerging Sensing Technologies
Aside from the integration of nanotechnology, novel sensing technologies are rapidly changing the landscape of zeta potential measurements. One notable aspect is the development of microfluidic devices that can efficiently handle small volumes of samples while providing real-time measurements. This new approach offers a more streamlined process with several key considerations:
- Real-Time Monitoring: This feature is indispensable in applications where immediate decisions are required, such as quality control in manufacturing processes. Monitoring zeta potential in real-time can help in tweaking formulations to maintain stability dynamically.
- Cost-Effectiveness: By reducing the amount of sample needed for analysis, emerging technologies minimize waste and lower overall costs, which is vital for research institutions and industries alike.
- Versatility Across Fields: These technologies are not only limited to pharmaceuticals; they span various fields, including environmental assessments and food safety, to name a few. This versatility makes understanding zeta potential increasingly relevant across scientific disciplines and industries.
Future Directions in Research
The exploration of zeta potential measurement through dynamic light scattering (DLS) is continuously evolving, paving way for new methodologies and applications. Understanding the future directions in this field is paramount for researchers and professionals who are eager to stay on the cutting edge. It opens a realm of possibilities that can lead to enhanced accuracy, greater efficiency, and broader applications across various scientific domains.
Potential Innovations in Measurement Techniques
With technological advancements proceeding at a breakneck pace, the future of measurement techniques related to zeta potential is promising. Innovations may include:
- Super-resolution Techniques: Using advanced optics to track smaller particles with greater precision.
- Integration of AI and Machine Learning: Employing algorithms that can predict zeta potential based on extensive datasets, thus reducing human error and saving time.
- Real-time Measurements: Developing instruments capable of continuous monitoring would allow for immediate feedback during experiments.
These innovations not only enhance the measurement process but also contribute to a better understanding of the underlying dynamics involved in colloidal stability. By refining measurement techniques, researchers can attain higher fidelity data that offers deeper insights.
Holistic Approaches to Colloidal Chemistry
An integrated view of colloidal chemistry is becoming increasingly important. Rather than studying isolated aspects of zeta potential, a more comprehensive approach considers various experimental conditions that collectively influence results. This includes:
- Multimodal Analysis: Analyzing zeta potential alongside other parameters such as viscosity, particle size, and pH can lead to a more accurate understanding of the stability and behavior of colloids.
- Collaboration Across Disciplines: Bringing together expertise from chemistry, physics, and engineering enhances the research. In particular, interdisciplinary work could foster novel applications in pharmaceuticals, food sciences, and nanotechnology.
- Longitudinal Studies: Research that tracks changes over time in zeta potential and related factors is vital. Understanding how conditions such as temperature change or ionic strength adjustments impact colloids over long periods can inform better engineering of formulations.
In a nutshell, the future research landscape for zeta potential measurement is vibrant, intermingled with innovative techniques and comprehensive strategies. By harnessing these insights, professionals can strive for more reliable and applicable results, essentially pushing the envelope of what is currently known and understood in the realm of colloidal systems.
"Innovation distinguishes between a leader and a follower." – Steve Jobs
"Innovation distinguishes between a leader and a follower." – Steve Jobs
Staying abreast of these advancements will avouch that practitioners are not just participants but leaders in the unfolding narrative of colloidal research and practical applications.
Culmination
The importance of understanding zeta potential measurement using dynamic light scattering (DLS) cannot be overstated, particularly in the realms of science and industry where it plays a pivotal role. This article highlights the multifaceted applications and significance of zeta potential, underscoring its crucial impact on colloidal stability.
Summary of Key Insights
In the course of our discussion, several key insights have emerged:
- Zeta Potential as an Indicator: Zeta potential serves as a fundamental indicator of the stability of colloidal dispersions. It provides essential insights into the repulsive and attractive forces acting between particles in a colloidal system.
- Dynamic Light Scattering methodologies: We explored the intricacies of DLS, revealing how it harnesses the principles of scattering light to yield valuable measurements of particle size and zeta potential. The sophistication and precision of modern DLS equipment enhance its reliability in various applications.
- Influences on Measurement: Factors such as pH, ionic strength, and temperature can significantly influence the measured values. Understanding how these variables interact with the zeta potential is crucial for accurate interpretation.
- Industrial and Environmental Relevance: The utility of zeta potential measurement spans a broad spectrum of fields, from pharmaceuticals to environmental science, showcasing its versatility and importance in both research and industrial applications.
Implications for Future Research
Looking ahead, the implications for future research in this area are vast. Given the rapid advancements in nanotechnology and sensing methods, there’s a clear opportunity for:
- Innovative Measurement Techniques: Developing new methodologies that could yield faster and more accurate zeta potential measurements presents an exciting frontier.
- Holistic Approaches: Future work can integrate a broader context of colloidal chemistry, taking into account how various factors interrelate in complex systems.
- Interdisciplinary Collaboration: The convergence of different scientific disciplines can refine zeta potential applications, opening the door for novel findings that enhance both theoretical knowledge and practical applications.
This article ultimately serves as a foundation for a deeper understanding of zeta potential measurement techniques. By synthesizing information from diverse fields and addressing contemporary challenges, the future seems promising for both researchers and practitioners in this dynamic arena.