Assessing the Rate of Iron: Methods and Applications
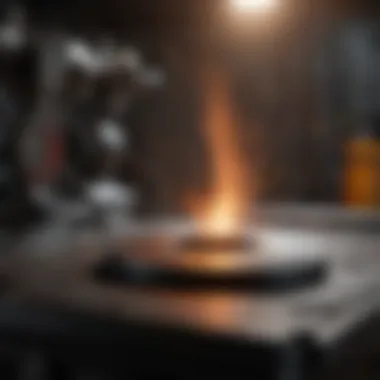
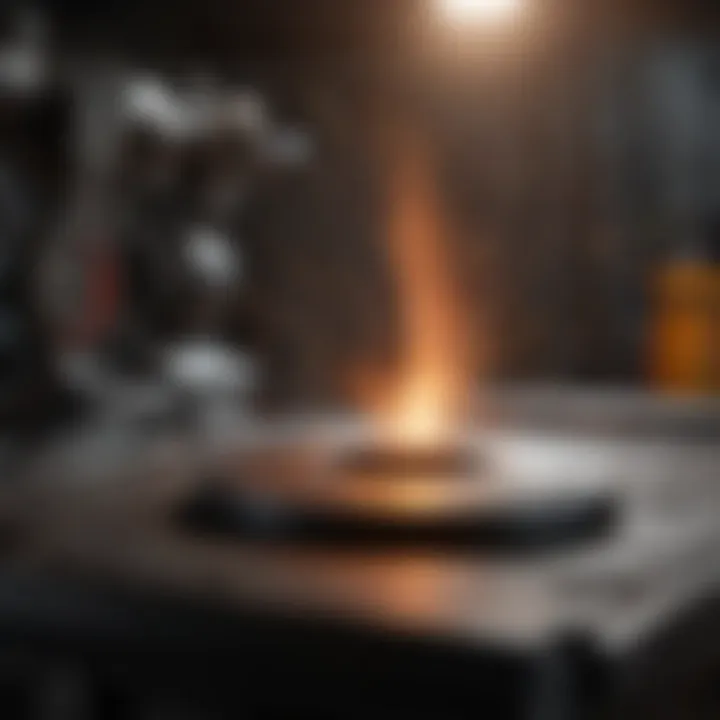
Intro
Iron plays a crucial role in various biological and industrial processes. Due to its significance, assessing the rate of iron is essential in multiple contexts, including health sciences and manufacturing. Understanding how to measure iron accurately can lead to better nutritional guidelines and improved industrial processes. This article aims to examine different methodologies and analytical techniques for determining the rate of iron, their practical applications, and the underlying scientific principles that guide them.
Key Concepts
Definition of the Main Idea
Assessing the rate of iron entails analyzing its concentration within a specific sample. This could relate to both biological systems, such as blood or tissues, and industrial applications, including materials science. Accurate measurement is necessary to understand iron's role and ensure standards are met.
Overview of Scientific Principles
Iron is abundant in nature and involved in several biochemical processes. In biological systems, it is a fundamental component of hemoglobin, the molecule responsible for oxygen transport in blood. On the industrial side, its use ranges from alloys to catalysts. The methodology for measuring iron often depends on the context, with various analytical methods available. Common techniques include spectrometry, titration, and chromatic analysis, each serving distinct purposes based on accuracy and required sensitivity.
Current Research Trends
Recent Studies and Findings
Recent studies indicate a growing focus on nanotechnology in iron assessment. These studies reveal that nanomaterials can enhance detection sensitivity and reduce the time required for analysis. Research shows promising advancements in biosensors that allow for real-time monitoring of iron levels in various contexts.
Significant Breakthroughs in the Field
One noteworthy breakthrough is the development of portable spectrometers, which enable on-site measurements without the need to transport samples. This technology has far-reaching implications for both clinical and environmental testing. With easier access to accurate data, professionals can make informed decisions in both health and industrial settings.
Understanding iron's dynamics is essential to improving health and boosting industrial performance. Accurate assessment methods are key in this journey.
Understanding iron's dynamics is essential to improving health and boosting industrial performance. Accurate assessment methods are key in this journey.
This article will elaborate on the specific methods employed in assessing the rate of iron, the challenges faced, and potential solutions to improve accuracy and efficiency.
Prelims
The evaluation of iron levels in various contexts holds significant importance in both health and industry. Iron’s role as a vital nutrient in human physiology cannot be overstated. It is a key component in the formation of hemoglobin, facilitating oxygen transport in the blood. Proper iron levels are essential for maintaining energy levels and overall health. On a broader scale, iron is also foundational in industrial applications, particularly in steel production and various manufacturing processes.
In this article, we aim to articulate the methodologies used in assessing the rate of iron. Through this exploration, we will examine the analytical techniques applied in both biological and industrial settings. Furthermore, understanding these methods will help to highlight the relevance of accurate iron measurement, driving its significance in preventing health issues and enhancing manufacturing efficiency.
By discussing the factors affecting iron levels, ranging from biological to environmental influences, we provide a holistic view of the challenges faced in iron assessment. With the integration of case studies and current technological advancements, we endeavor to present a comprehensive guide that bridges the gap between scientific understanding and practical application. This examination of iron serves to underscore its multifaceted role as both a nutritional necessity and an industrial asset.
Understanding Iron
Iron is an essential element prevalent in all living organisms. It exists in various forms, primarily categorized into heme and non-heme iron. Heme iron, found in animal sources, is generally more bioavailable than non-heme iron, which predominantly comes from plant sources. This difference in bioavailability is crucial when discussing dietary sources and the implications for iron deficiency in diverse populations.
The body's iron stores are regulated by a sophisticated interplay of absorption, utilization, and excretion. The liver plays a central role in storing iron as ferritin, releasing it into circulation as required. This complex regulation can be disrupted by various factors, leading to deficiencies or excesses that can pose health risks.
Moreover, iron participates in numerous biological reactions, including those involving enzymes responsible for energy production and DNA synthesis. Understanding these biochemical roles provides critical insight into why maintaining appropriate iron levels is central to good health.
Importance of Iron Rate Assessment
Assessing iron levels is vital for a range of important reasons. First, it aids in diagnosing iron deficiency anemia, a common condition affecting many individuals worldwide. Symptoms can range from fatigue to impaired cognitive function, severely impacting quality of life. Accurate measurement of iron facilitates timely intervention, potentially reversing deficiencies and preventing long-term health issues.
In industry, monitoring iron content ensures the quality and reliability of products. For instance, in steel manufacturing, maintaining the correct iron levels is critical to achieving desired material properties. In pharmaceuticals, accurate iron measurement can influence drug formulation and stability, particularly in iron supplement production.
Accurate assessment can prevent issues related to both over-supplementation and insufficiency, making it a cornerstone of health management and quality control in various fields.
Accurate assessment can prevent issues related to both over-supplementation and insufficiency, making it a cornerstone of health management and quality control in various fields.
Various analytical methods exist for measuring iron, each with its strengths and limitations. Understanding these methods not only enriches scientific knowledge but also empowers professionals in healthcare and industry to make informed decisions based on precise data. As we delve deeper into these methodologies, the significance of iron assessment will become even clearer.
Types of Iron
Understanding the different types of iron is crucial in the assessment of its rate. Iron exists in various forms, and its classification into heme and non-heme iron is significant for multiple reasons.
First, heme iron is generally more bioavailable compared to non-heme iron. The body absorbs heme iron more efficiently, making it an essential component of diet for individuals at risk of iron deficiency. On the other hand, non-heme iron, while widely present in plant-based foods, presents absorption challenges. This consideration impacts dietary planning particularly for vegetarians and those with health conditions requiring careful iron monitoring.
Second, the different sources of these iron types affect their role in industry and research. Heme iron is primarily found in animal products like meat and fish, thus playing a key role in nutrition research. Non-heme iron from plants is essential for agricultural studies focusing on soil health and crop yield.
Moreover, understanding these types helps delineate appropriate assessment methods. Tools and techniques for measuring heme iron differ from those applied to non-heme iron, making clarity in types essential for precision in analytical chemistry.
Consequently, distinguishing between heme and non-heme iron not only influences dietary recommendations but also informs industrial applications. This understanding enhances the relevance of iron assessment methodologies discussed throughout this article.
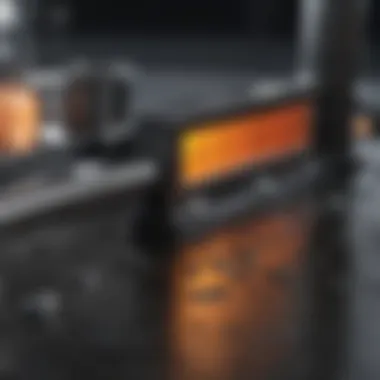
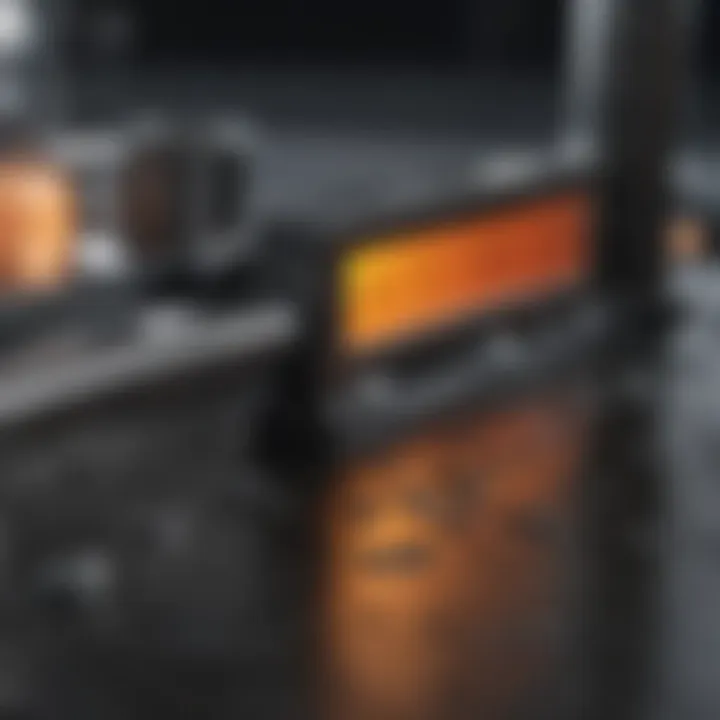
Heme Iron
Heme iron, derived primarily from hemoglobin and myoglobin in animal tissue, plays a significant role in the human diet. This form of iron accounts for around 15% of dietary iron intake, yet its absorption rate can be as high as 25%. The presence of heme in red meat, poultry, and fish contributes to its efficiency in elevating iron stores in the body.
The importance of heme iron extends beyond nutrition. In medical research, measuring heme iron concentrations assists in diagnosing various conditions, including anemia and other hematological disorders. Analytical methods for examining heme iron often involve spectrophotometric techniques, which provide quick and precise results.
Non-Heme Iron
Non-heme iron, on the other hand, constitutes the majority of iron found in plant-based foods and fortified products. It is significantly less absorbable, often reliant on the presence of enhancers like vitamin C or the absence of inhibitors such as calcium. This disparity in absorption rates means that non-heme iron remains a critical area of study, especially in addressing iron deficiency in populations with predominantly plant-based diets.
In terms of measurement, the analytical approach for non-heme iron can involve various methods, including chromatography and mass spectrometry. These diverse methodologies ensure accurate assessment, taking into account the complex interactions influencing non-heme iron bioavailability.
In summary, a clear understanding of heme and non-heme iron is vital as it affects both personal health and broader industrial applications. Recognizing their differences strengthens our ability to assess iron accurately, ensuring both health and industrial standards are met.
Factors Influencing Iron Levels
The assessment of iron levels is complex, as various factors contribute to its regulation within biological systems. Understanding these factors is crucial for both health and industrial applications. Iron levels are not static; they fluctuate due to numerous influences. Identifying these influences can help improve diagnostic accuracy and optimize iron usage in industries, thus highlighting their importance.
Biological Factors
Age
Age plays a significant role in determining iron levels in the body. Infants, children, adults, and the elderly each have distinct iron requirements and absorption mechanisms. For example, infants often require more iron due to rapid growth, while older adults may have decreased absorption due to various physiological changes.
One key characteristic of age is that dietary iron needs shift over the lifespan. This variability makes age a beneficial choice for assessment in the article. Unique features of age include the hormonal changes that influence iron metabolism. These age-related factors offer advantages in tailoring dietary recommendations or medical interventions, ensuring that populations receive optimal care and monitoring.
Gender
Gender also significantly impacts iron levels. Generally, women have higher iron requirements than men due to menstruation and pregnancy. This difference highlights the need for gender-specific assessments. One important characteristic of gender is its impact on health statistics; for instance, iron deficiency is more prevalent among women of reproductive age.
Gender is beneficial for this article because it allows for deeper exploration into tailored solutions in the health context. A unique feature of gender discussions is the examination of societal roles that may affect diet and accessibility to iron-rich foods. Such considerations present advantages in addressing iron deficiency effectively through targeted interventions.
Health Conditions
Health conditions can drastically influence iron levels. Individuals with chronic diseases, such as diabetes or kidney disorders, often experience altered iron metabolism. Another important aspect of health conditions is their underlying causes, which can lead to either iron overload or deficiency.
Health conditions are vital for this article because they demonstrate the complex interplay between pathology and nutrition. Here, a unique feature is the potential for misdiagnosis, where low iron levels may be incorrectly interpreted as a deficiency when in fact, it is a secondary effect of another condition. Understanding this relationship allows for better patient care and improved outcomes.
Environmental Factors
Geological Composition
Geological composition of the local environment significantly affects iron availability in soil and water. Regions rich in iron ores tend to have higher ambient iron levels, influencing both agriculture and health in the vicinity. A key characteristic of geological composition is its direct impact on dietary sources of iron for local populations.
This consideration is beneficial for the article because it connects environmental science with public health. One unique feature is the variance in iron content across geographical regions, which can lead to population-level deficiencies or excesses. Thus, a detailed understanding of geology provides a context for assessing iron status in different communities.
Pollution
Pollution is another critical environmental factor that can alter iron levels. Contaminants in air and water can affect the bioavailability of iron, either positively or negatively. One key characteristic of pollution's impact is how industrial activities can release iron-rich particles that temporarily increase local availability, while long-term contamination may reduce overall dietary iron through soil degradation.
Pollution is an important aspect to consider in this article as it emphasizes the interconnectedness of environmental health and iron levels. A unique aspect of pollution is its potential to prompt public health interventions aimed at environmental cleanup, thereby improving community iron intake. Understanding pollution's role can lead to better policies that address both iron deficiency and environmental sustainability.
The interplay between biological and environmental factors in iron levels underscores the need for comprehensive approaches in both healthcare and industry.
The interplay between biological and environmental factors in iron levels underscores the need for comprehensive approaches in both healthcare and industry.
Analytical Techniques for Measuring Iron Rate
Measuring iron levels is crucial in various fields, including biology, environmental science, and industrial applications. Analytical techniques for measuring iron rate provide reliable methods to assess the concentration and distribution of iron in different samples. The choice of technique often depends on the sample type, the required accuracy, and specific application needs. Understanding these techniques allows researchers and professionals to make informed decisions regarding iron analysis.
Spectrophotometry
Spectrophotometry is a widely used analytical technique for measuring iron concentrations in various samples. This method depends on the principle that substances absorb light at specific wavelengths. In terms of iron, certain wavelengths correlate with iron-containing compounds, making it possible to determine the concentration based on light absorption.
The process typically involves preparing a sample, which might include dissolving iron in an appropriate solvent and reacting it with specific reagents to form a colored complex. This complex can then be measured using a spectrophotometer. The intensity of the color directly relates to the iron concentration, which allows for accurate quantification.
Some advantages of spectrophotometry include its relative simplicity, low cost, and non-destructive nature. However, it is important to consider possible interference from other substances within the sample, which could skew results. Proper sample preparation and calibration are crucial to minimize these issues.
Chromatography
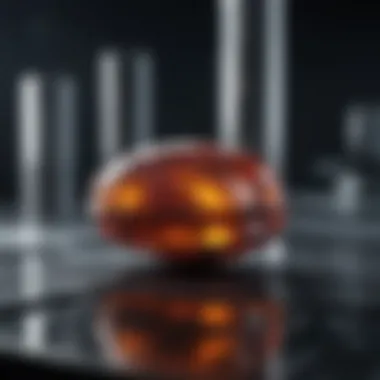
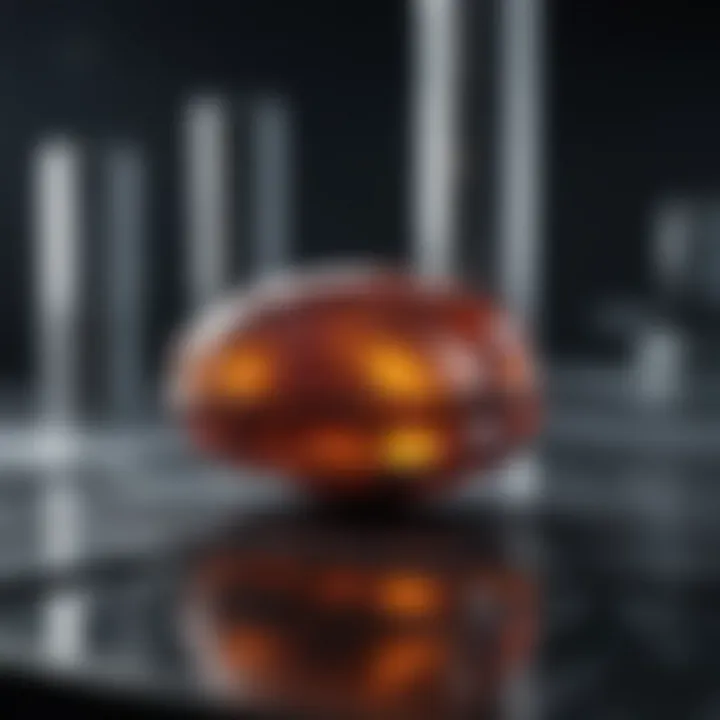
Chromatography encompasses a group of techniques used to separate and identify components within a mixture. When it comes to measuring iron, liquid chromatography is often employed. This method enables the separation of iron forms, such as heme and non-heme iron, based on their chemical properties.
In liquid chromatography, a sample is passed through a column filled with a stationary phase. Different iron species interact with the stationary phase at varying degrees, which leads to their separation. Afterward, a detection method, such as UV-VIS spectrophotometry or mass spectrometry, can be employed to quantify the different iron species.
This technique is particularly useful for its specificity. It allows for the analysis of complex biological samples, like blood, without the need for extensive sample preparation. However, it requires advanced equipment and may demand technical expertise to interpret the results correctly. Despite these challenges, liquid chromatography remains a powerful tool in iron analysis.
Mass Spectrometry
Mass spectrometry is a highly sensitive analytical technique that can provide detailed information about iron levels in various forms. This method works by ionizing chemical species and measuring their mass-to-charge ratios. Mass spectrometry excels in its ability to detect trace amounts of iron, making it an essential tool in both research and industrial applications.
In practice, samples are ionized and introduced into the mass spectrometer. The resulting ions are then separated based on their mass-to-charge ratio, and their relative abundances are measured. This data allows for not only the quantification of iron but also insights into its molecular structure and composition.
One of the strong suits of mass spectrometry lies in its resolution. It can distinguish between different isotopes and oxidation states of iron, which is important in biological systems where iron's role can vary depending on its form. However, this high level of detail comes with challenges. The technique is complex, requiring significant expertise and expensive instrumentation, which may not always be feasible for every laboratory setting.
"A combination of these techniques can often provide the most reliable insights into iron levels, capitalizing on their respective strengths and compensating for weaknesses."
"A combination of these techniques can often provide the most reliable insights into iron levels, capitalizing on their respective strengths and compensating for weaknesses."
Iron in Biological Samples
Iron is an essential element in biological systems. Its measurement in biological samples is crucial for understanding various physiological processes and diagnosing health conditions. Accurate assessment of iron levels can reveal insights into metabolic health, nutritional status, and disease progression. In various contexts, such as clinical and research settings, analyzing iron concentrations in biological samples is instrumental in identifying deficiencies or overloads.
Blood Iron Levels
Blood iron levels provide significant information about the body’s iron status. The primary forms of iron present in blood are heme iron, which is part of hemoglobin, and non-heme iron, which is found in serum. Measuring these levels helps healthcare professionals evaluate an individual’s iron reserves and overall nutritional health.
The most common tests include serum ferritin, which indicates stored iron, and transferrin saturation, which depicts the available transport capability of iron within the bloodstream. An abnormality in these levels can suggest conditions such as iron deficiency anemia or hemochromatosis, a disorder leading to excess iron accumulation.
In clinical practice, laboratories usually employ spectrophotometric methods for assessing blood iron levels. This technique allows for sensitive and specific quantification of iron concentrations, ensuring that correct diagnostic decisions are made.
Tissue Iron Measurements
Tissue iron measurements provide further insight into the body’s iron utilization and storage. Unlike blood tests, which reveal immediate iron status, tissue analysis helps to assess long-term iron distribution across various organs. Organs such as the liver, heart, and bone marrow are critical sites where iron is stored and metabolized.
One common approach for tissue iron measurement is the use of biopsy samples, specifically from the liver. This gives a clear picture of iron overload, particularly in conditions like thalassemia and sideroblastic anemia, where the body’s regulatory mechanisms are impaired.
Modern tools, such as magnetic resonance imaging (MRI), can also non-invasively assess iron in tissues, offering a less disruptive means for tracking iron status over time. The development of these technologies highlights the importance of accurately measuring iron levels in biological samples to ensure effective treatment of iron-related disorders.
Iron is an indispensable nutrient, yet its absorption and usage by the body must be finely balanced to avoid adverse health effects.
Iron is an indispensable nutrient, yet its absorption and usage by the body must be finely balanced to avoid adverse health effects.
Thus, understanding iron levels in blood and tissues is vital in both clinical and research scenarios. Recognizing the unique roles of blood and tissue measurements allows for a more comprehensive approach to iron assessment.
Industrial Applications of Iron Measurement
Iron plays an essential role in many industries. The measurement of iron levels is critical not only for ensuring product quality but also for optimizing processes within various applications. Accurate assessment of iron content can influence the properties of materials, ensuring that they meet specified standards. This section will delve into two key applications: steel production and pharmaceutical applications.
Steel Production
The steel industry heavily relies on the precise measurement of iron. Iron is the major component in the production of steel, and its purity significantly impacts the mechanical properties of the final product. High-quality steel requires low levels of impurities and accurately knowing the iron content ensures that the material meets industry standards.
To achieve the desired steel characteristics, manufacturers often perform various tests to determine the iron content. Common methods include spectrophotometry and mass spectrometry for real-time analysis.
Some key benefits of precise iron measurement in steel production include:
- Quality Control: Consistent iron levels lead to reliable quality in steel products.
- Production Efficiency: Knowing the right amount of iron can help optimize raw material usage, reducing waste and costs.
- Safety: Steel components often serve critical roles in construction and manufacturing. Ensuring correct iron levels is crucial for the safety of structures.
Pharmaceutical Applications
In pharmaceuticals, iron measurement has its own significance. Iron plays a vital role in various biological processes and is often used in supplements and formulations. Accurate assessment of iron content in medicines is essential to avoid potential toxicity and ensure efficacy.
Iron can exist in both heme and non-heme forms. The pharmaceutical industry typically favors non-heme iron due to its higher stability and lower side effects when consumed in supplements.
Key considerations in this sector include:
- Dosage Accuracy: Proper measurement ensures that products deliver precise dosages of iron for therapeutic effects.
- Regulatory Compliance: Pharmaceutical products must comply with strict regulations that dictate iron content. Accurate assessment helps in meeting these requirements.
- Research and Development: Understanding the behavior of iron in formulations can aid in the development of new drugs, making its measurement crucial in ongoing research.
"Effective iron measurement opens doors for innovations in both industry and healthcare, ensuring safety and compliance."
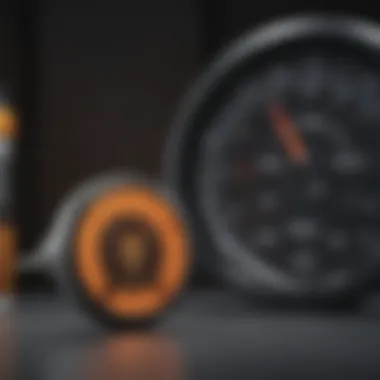
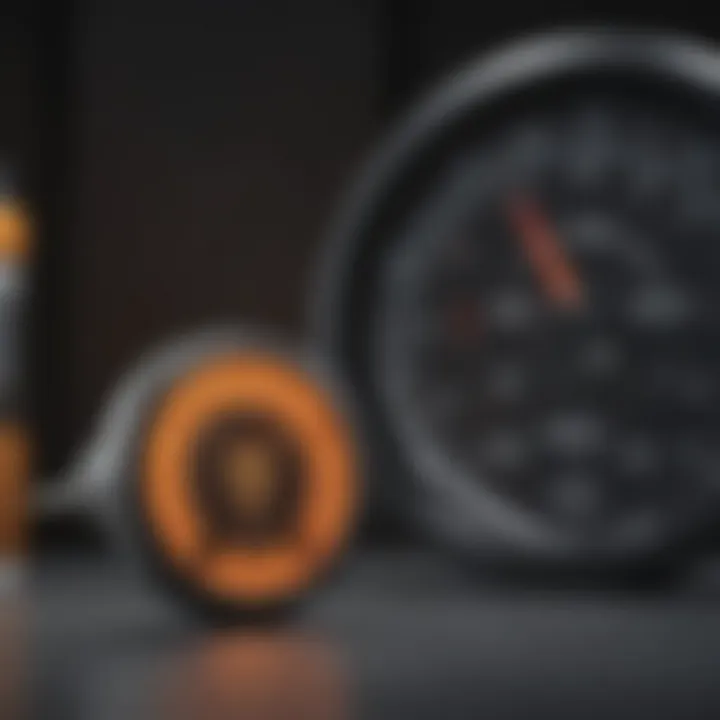
"Effective iron measurement opens doors for innovations in both industry and healthcare, ensuring safety and compliance."
The insights gained from careful iron measurement can lead to significant advancements in both steel production and pharmaceutical applications. As the industry evolves, new analytical methods and technologies will continue to refine these processes, ensuring higher standards of accuracy and reliability.
Limitations of Current Methods
Understanding the limitations of analytical techniques used for measuring iron is critical in both research and industrial contexts. Despite advances in technology, several challenges persist that can affect the accuracy and reliability of iron assessments. Highlighting these limitations allows researchers and professionals to adopt better practices, ensure precision, and understand potential pitfalls in their methodologies. Thus, it becomes essential to examine these limitations to improve the assessment of iron rates in various applications.
Contamination Issues
Contamination is a significant concern when measuring iron levels in biological and environmental samples. Even trace amounts of foreign substances can skew results, leading to incorrect interpretations regarding iron concentrations. For instance, materials in the laboratory environment or from the tools used can contaminate samples. For example, iron filings from a steel workbench might be introduced into a sensitive blood iron measurement. Moreover, cross-contamination between samples during handling can also create misleading data.
To mitigate contamination, strict protocols should be adhered to, such as using certified clean rooms or employing single-use equipment. Additionally, validated cleaning procedures for instruments and sample containers can help reduce the risk of contamination. Ensuring that samples remain isolated from external influences is essential for achieving reliable results in iron measurements.
Calibration Challenges
Calibration is another area where challenges frequently arise. Properly calibrated instruments are crucial for coherent and valid measurements. Techniques such as spectrophotometry, chromatography, and mass spectrometry require frequent calibration against known standards to ensure their accuracy. However, obtaining quality calibration standards can be complex due to variations in source material and environmental factors that can alter standards over time.
Moreover, the process of calibrating instruments can be time-consuming and may introduce human error. Regular monitoring and recalibrating can be neglected, especially in busy lab settings, leading to biased or flawed results. It is essential to develop a routine for calibration checks as part of good laboratory practices to enhance data integrity.
Accurate and reliable calibration is essential in any analytical measurement. Inaccurate calibrations can lead to substantial errors in determining iron levels.
Accurate and reliable calibration is essential in any analytical measurement. Inaccurate calibrations can lead to substantial errors in determining iron levels.
Future Directions in Iron Rate Research
The field of iron rate research is rapidly evolving, with new methodologies and technologies enhancing our understanding of iron's role in various contexts. This section delves into the future of iron assessment, specifically focusing on innovative technologies and interdisciplinary approaches that promise to advance research and application in this arena. Such developments not only improve measurement accuracy but also pave the way for comprehensive understandings across multiple fields.
Innovative Technologies
Emerging Instrumentation
Emerging instrumentation offers significant advancements in how researchers measure iron levels. One noteworthy technology is the increase in miniaturized devices such as portable spectrometers and lab-on-a-chip systems. These instruments can rapidly analyze samples with minimal preparation, providing real-time data. A key characteristic of this instrumentation is its ability to operate in diverse environments, making it suitable for both field and laboratory settings.
The benefit of using such technologies lies in their accessibility and flexibility. They allow for on-site analysis, which eliminates the need for transporting samples and potentially altering their integrity. The unique feature of lab-on-a-chip technology is its capacity to perform multiple analyses simultaneously, saving time and resources. However, these systems do face challenges, particularly concerning sensitivity and calibration, which require ongoing research and development.
Improved Data Analysis
Improved data analysis techniques are also fundamental to the future of iron rate research. The integration of machine learning and artificial intelligence (AI) into analytical methods allows for more sophisticated interpretation of complex datasets. These techniques enhance the predictive capabilities of iron assessments, providing richer insights into iron dynamics in various contexts.
The key characteristic of improved data analysis is the ability to process large datasets efficiently. This is particularly useful in studies that involve numerous variables, where traditional analysis might fall short. One unique feature of AI-driven analysis is its capacity to identify patterns and relationships that might be overlooked by human researchers. Nonetheless, reliance on technology necessitates a careful understanding of its limitations, including the quality of input data and algorithm bias, which could potentially skew results.
Interdisciplinary Approaches
Interdisciplinary approaches are essential for advancing iron rate research, bridging gaps between different scientific domains. Collaboration among chemists, biologists, environmental scientists, and data analysts can foster innovative solutions to long-standing challenges in iron measurement. Such partnerships can lead to comprehensive studies that not only evaluate iron levels but also examine the broader ecological and health-related impacts.
Consequently, interdisciplinary projects often result in more robust findings, as they incorporate diverse methodologies and perspectives. This trend highlights the importance of synthesizing knowledge from various fields, creating a more nuanced understanding of iron's complexity.
Overall, the future of iron rate research is promising, with emerging technologies and transformative collaborations paving the way for enhanced methodologies and applications in health and industrial contexts.
Finale
The conclusion of this article serves a critical role in summarizing the extensive discussions surrounding iron rate assessment. It encapsulates the key insights derived from the methodologies and applications of measuring iron, underscoring its significance across various fields, from healthcare to industrial sectors. The various analytical methods provide essential data for understanding iron's role, especially in biological samples and industrial contexts.
A clear understanding of iron levels can aid in diagnosing health conditions, monitoring nutritional status, and ensuring the quality of steel in manufacturing processes. Moreover, recognizing limitations in current methods allows for the identification of areas needing attention, fostering advancements in technology and techniques.
In essence, this conclusion reinforces the relevance of rigorously assessing iron rates, highlighting the need for precise measurements in both biological and industrial applications. This exploration leads to better health outcomes and improved industrial efficiencies.
Summary of Key Points
- Analytical Methods: Various techniques such as spectrophotometry, chromatography, and mass spectrometry are crucial in accurately assessing iron levels.
- Impact on Health: Understanding iron levels is vital for diagnosing anemia and other health conditions related to iron deficiency or overload.
- Industrial Relevance: In steel production and pharmaceuticals, precise iron measurement ensures product quality and efficiency, impacting economic aspects.
- Limitations: Current methods face issues like contamination and calibration difficulties, necessitating ongoing research and innovation.
Implications for Research and Industry
The implications of iron rate assessment are broad and significant, affecting both ongoing research and practical applications in industry. For researchers, understanding the methodologies improves the accuracy of studies involving iron, paving the way for groundbreaking discoveries in health science and material science.
In industry, the accurate measurement of iron influences production processes, ensuring regulatory compliance and product integrity. Innovations in measurement techniques could lead to reduced costs and increased safety, benefiting both businesses and consumers.
Furthermore, interdisciplinary approaches encourage collaboration between fields, driving the development of advanced technologies. This synergy can yield more robust analytical methodologies, enhancing both academic research and industrial practices.
"The quest for precision in measuring iron rates is not merely an academic exercise; it embodies the intersection of health and industry, where science meets practical application."
"The quest for precision in measuring iron rates is not merely an academic exercise; it embodies the intersection of health and industry, where science meets practical application."
With ongoing advancements, the future of iron assessment holds promise for improved methodologies, better health outcomes, and enhanced industrial practices.