Calcium ATPase: Mechanisms, Functions, and Health Impacts
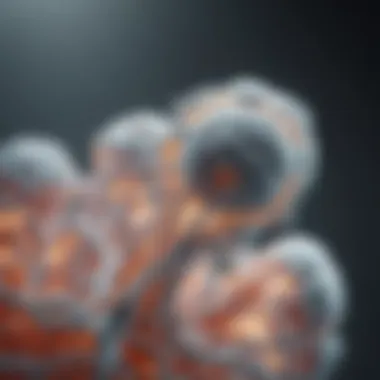
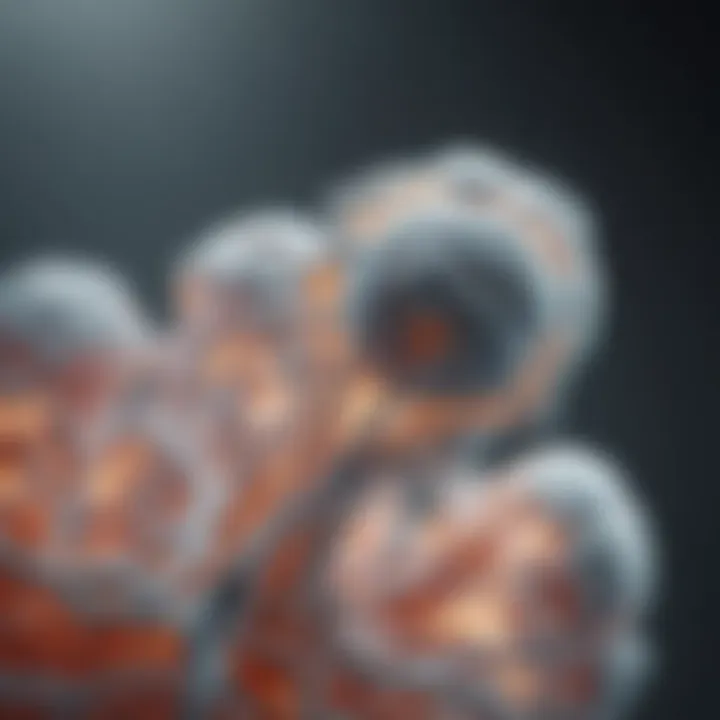
Intro
Calcium ATPase is a cornerstone enzyme that plays a pivotal role across various biological systems. It handles the transport of calcium ions, which are crucial for multiple cellular functions. Understanding at what level calcium ATPase operates opens up doors to analyzing intricate muscle movements and signal transduction pathways. It’s not just about the mechanics of ion transport; it's about grasping how these tiny units affect bigger biological processes and overall health.
This article aims to explore the complexity of calcium ATPase in depth. We’ll focus on its mechanisms, the essential roles it plays in our bodies, and the implications of its functionality. From significant advances in research to practical applications, we’ll venture into the world of cellular dynamics where calcium ATPase stands as a powerful player.
Key Concepts
Definition of the Main Idea
Calcium ATPase, at its core, is an enzyme that catalyzes the hydrolysis of ATP to pump calcium ions across cellular membranes. This movement is essential for maintaining cellular calcium levels, which are vital to many physiological functions such as contraction of muscles, neurotransmitter release, and cell signaling. Without this regulation, cells can fall into chaos, leading to dysfunction or disease.
Overview of Scientific Principles
To appreciate how calcium ATPase operates, understanding a few scientific principles is necessary. First off, ATP, or adenosine triphosphate, is our body's primary energy currency. When calcium ATPase hydrolyzes ATP, it uses this energy to transport calcium ions against their concentration gradient.
This results in a low calcium concentration inside the cell and a high concentration outside, crucial during muscle relaxation. To illustrate, think of calcium ATPase as a diligent bouncer at a club, ensuring that only a select number of calcium ions enter while maintaining control at the entrances and exits of cells.
Ultimately, calcium ATPase's function is an excellent example of an active transport mechanism, utilizing energy to maintain essential cellular conditions. This enzymatic activity is fine-tuned and reflects broader biological principles that transcend mere muscle function and signaling.
Current Research Trends
Recent Studies and Findings
Research surrounding calcium ATPase has gained traction as scientists delve deeper into understanding its role not only at a cellular level but also in broader physiological contexts. Recent investigations have shed light on how disruptions in calcium ATPase activity can lead to disorders like heart disease and neurological conditions.
For instance, studies indicated that a diminished calcium ATPase function is often present in failing heart muscle, emphasizing its importance in cardiac function. Additionally, emerging research has begun to focus on potential pharmacological agents that could modulate calcium ATPase activity, presenting possible new avenues for treatment.
Significant Breakthroughs in the Field
A notable breakthrough is the creation of molecular models that simulate calcium ATPase's operations. With advanced imaging techniques, researchers can visualize the enzyme's structure and its interactions with calcium ions and ATP. This opens up new pathways for drug design aimed at enhancing or inhibiting calcium ATPase function.
Moreover, addressing the therapeutic potentials tied to this enzyme might lead to innovative treatments for a variety of diseases. The prospect of using calcium ATPase modulators in clinical settings is highly anticipated among researchers, as it could change the landscape of how certain conditions are treated.
Foreword to Calcium ATPase
Calcium ATPase is often seen as a pivotal player in the realm of cellular physiology, and this section serves as a gateway into its intricate workings. Understanding this enzyme is essential for various fields, from biomedical research to pharmacology, given its far-reaching implications in health and disease. To truly appreciate the complexity and functionality of calcium ATPase, one must delve into its definition, historical background, and why it matters in the broader context of biological systems.
Definition and Significance
Calcium ATPase refers to a family of enzymes responsible for transporting calcium ions across cellular membranes, utilizing the energy derived from ATP hydrolysis. The significance of calcium ATPase can’t be overstated. This enzyme maintains calcium homeostasis, a vital physiological balance necessary for muscle contractions, nerve transmission, and several other critical cellular processes.
In essence, calcium ATPase acts like a bouncer at a club, determining who gets in or out while ensuring that the flow of calcium aligns with physiological needs. When it functions properly, muscle contractions are smooth, neuronal signals are clear, and overall cellular health is maintained. Conversely, any disruptions in its activity can lead to a host of pathologies.
"Calcium is a universal signaling molecule, and calcium ATPase is its diligent custodian, ensuring that its levels are just right for proper cellular function."
"Calcium is a universal signaling molecule, and calcium ATPase is its diligent custodian, ensuring that its levels are just right for proper cellular function."
Moreover, understanding calcium ATPase and its dynamics could shape future therapeutic strategies, targeting diseases linked with calcium dysregulation. For researchers and students alike, unraveling the nuances of this enzyme opens doors to exploring innovative treatments and interventions.
Historical Perspective
The exploration of calcium ATPase isn’t merely a product of modern science; it has roots tracing back several decades. The journey began in the 1950s when the fundamental nature of cellular transport mechanisms started gaining attention. Researchers like Sutherland and Rall began propelling the understanding of how cells handle calcium ions, leading to discoveries that would eventually characterize the role of ATPases.
By the 1980s, techniques such as patch-clamp electrophysiology and X-ray crystallography began uncovering the molecular architecture of calcium ATPase, allowing scientists to visualize how it operates on a structural level. These advances paved the way for recognizing distinct types of calcium ATPase, such as the Plasma Membrane Calcium ATPase and Sarcoplasmic Reticulum Calcium ATPase, each serving specific roles in various tissues.
In hindsight, the evolution of knowledge surrounding calcium ATPase reflects broader trends in biochemistry and molecular biology. From humble beginnings to sophisticated techniques adorned with Nobel accolades, the exploration of this enzyme serves as a testament to the ingenuity and persistence of the scientific community, pushing the boundaries of how we perceive cellular function.
In summary, this section lays the groundwork for a deeper understanding of calcium ATPase. Recognizing its definition, significance, and historical journey sets the stage for further exploration of its molecular mechanisms, physiological roles, and relevance in various pathologies.
Molecular Mechanism of Calcium ATPase
Understanding the molecular mechanism of calcium ATPase is not just for the biochemists in lab coats; it's crucial for anyone who wants to grasp how cells maintain their calcium balance and communicate effectively. This section brings to light the inner workings of calcium ATPase, highlighting its pivotal role in cellular activities while delving into ATP hydrolysis, the influence of magnesium ions, and the conformational changes that drive its function.
ATP Hydrolysis and Calcium Transport
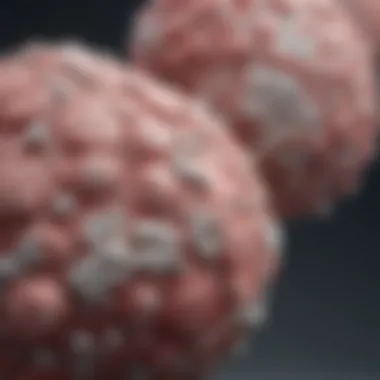
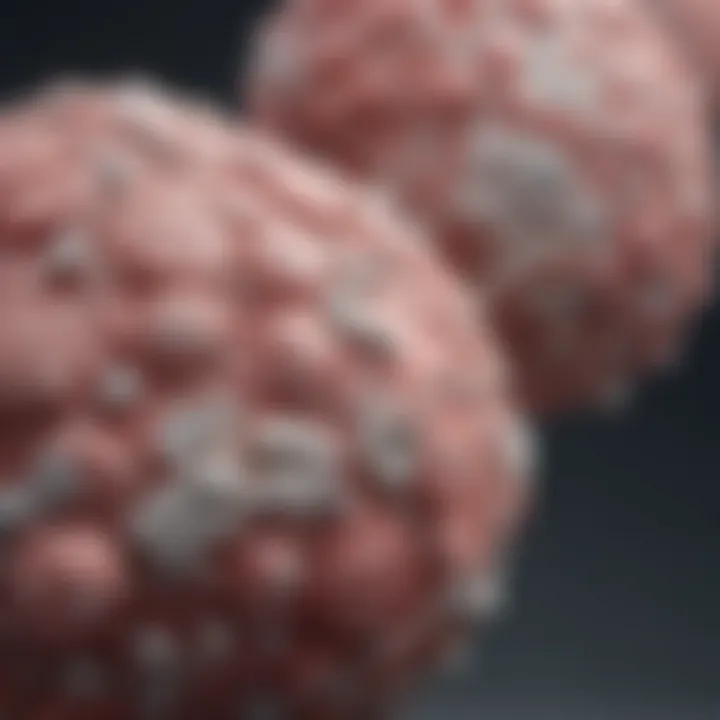
Calcium ATPase operates through a finely-tuned process that hinges on ATP hydrolysis. During this reaction, one molecule of ATP is broken down into ADP and inorganic phosphate, releasing energy. This energy is harnessed to transport calcium ions against their concentration gradient, essentially doing the "heavy lifting" for the cell.
When calcium ions need to be removed from the cytoplasm—let’s say after a muscle contraction or neurotransmitter release—ATP is hydrolyzed, converting chemical energy into mechanical work. Without this enzymatic feat, muscle cells wouldn’t relax properly and neurons could become overstimulated.
- Key points about ATP hydrolysis and calcium transport:
- ATP hydrolysis provides the energy required for the transport of calcium ions.
- Calcium ions are moved from areas of high concentration to lower concentration, which is critical for maintaining cellular homeostasis.
- This process is essential for muscle relaxation, neuronal firing, and overall cellular health.
Role of Magnesium Ions
One cannot downplay the importance of magnesium ions in the functioning of calcium ATPase. These ions are often seen as co-factors that enable the ATPase to do its job effectively. Magnesium helps stabilize the structure of ATP during hydrolysis, essentially "holding it together" so the enzyme can efficiently catalyze the reaction. In a way, magnesium is the unsung hero; without it, ATP could lose its effectiveness.
Pragmatically, when magnesium ion concentrations drop, it can lead to dysfunction in calcium ATPase activity. This imbalance may lead to numerous health issues, including muscle cramps or arrhythmias.
"Magnesium not only supports the action of calcium ATPase; it is a crucial player in the orchestra of cellular functions."
"Magnesium not only supports the action of calcium ATPase; it is a crucial player in the orchestra of cellular functions."
Conformational Changes
The operation of calcium ATPase is characterized by conformational changes, a kind of molecular choreography that transforms the enzyme as it binds, transports, and releases calcium ions. The enzyme shifts between different states during the cycle of calcium transport, which can be quite complex.
- Calcium Binding State: The enzyme first binds to calcium ions in the cytosol.
- Transition State: Once bound, conformational changes allow the enzyme to open up towards the outside, moving the calcium ions into the extracellular space.
- Release State: After releasing calcium, the enzyme undergoes further rearrangement to reset for the next cycle.
These structural transformations are not merely dramatic shifts; they are essential for the enzyme's functionality. Without these changes, calcium ATPase would find itself stuck in one position, unable to carry out its vital role in cellular signaling and muscle function.
Types of Calcium ATPase
Understanding the types of calcium ATPase is crucial to grasp fully how these enzymes operate within different biological contexts. Each variant of calcium ATPase fulfills specific roles that impact various physiological processes. This section navigates through the prominent types: Plasma Membrane Calcium ATPase (PMCA), Sarcoplasmic Reticulum Calcium ATPase (SERCA), and other variants. By dissecting their functions, benefits, and significance, we can appreciate how calcium ATPases contribute to maintaining cellular homeostasis and responding to physiological demands.
Plasma Membrane Calcium ATPase (PMCA)
The Plasma Membrane Calcium ATPase plays a vital role in regulating intracellular calcium levels, a key determinant of cellular excitability. Found in various tissues, notably nerve and muscle cells, PMCA is responsible for extruding calcium ions from cells. This action is critical in scenarios where calcium influx occurs, for instance, during muscle contraction and neuronal signaling.
In essence, PMCA ensures that excess calcium does not accumulate, preventing cellular toxicity and maintaining cellular function. This results in crucial benefits such as:
- Nerve Signal Termination: After neurotransmitter release, PMCA contributes to returning calcium levels to baseline, allowing for subsequent signaling events.
- Muscular Relaxation: By pumping calcium out of muscle cells, PMCA facilitates muscle relaxation post-contraction, restoring readiness for the next muscle action.
The efficiency of PMCA is affected by external factors, including pH and the presence of other ions. Understanding these dependencies is essential for appreciating its complex dynamics in health and disease.
Sarcoplasmic Reticulum Calcium ATPase (SERCA)
On the flip side, we have Sarcoplasmic Reticulum Calcium ATPase, primarily anchored in the sarcoplasmic reticulum of muscle cells. SERCA is crucial for absorbing calcium back into the sarcoplasmic reticulum post-stimulation. It serves as a central player in muscle contraction and relaxation cycles.
SERCA operates by utilizing ATP to pump calcium ions against their concentration gradient, a process significant for effective muscle function. Key functions include:
- Calcium Reuptake: SERCA helps restore calcium levels within the sarcoplasmic reticulum, readying the muscle for the next contraction.
- Contractility Regulation: The proper functioning of SERCA determines the strength and duration of muscle contractions, influencing overall muscle performance.
Malfunction of SERCA is closely tied to various muscle disorders, pointing to the enzyme's importance in both muscle health and pathology. Understanding SERCA's role can lead to insights into muscle-related diseases and potential avenues for intervention.
Other Variants
In addition to PMCA and SERCA, several other less-discussed variants of calcium ATPase exist. Though less prominent, they serve specialized functions in specific tissues or under certain conditions.
These may include:
- Extrusion ATPases in non-muscle cells: Various tissues utilize unique enzymes that can modulate calcium levels, contributing to cellular activities outside of typical muscle or neural function.
- Calcium ATPase in secretory cells: Certain glands implement calcium ATPases to regulate calcium-mediated exocytosis, revealing their role in hormonal and secretion pathways.
Exploring these variants sheds light on the diverse roles calcium ATPases play beyond well-known pathways, enhancing our understanding of cellular physiology.
The diversity of calcium ATPases highlights their evolutionary adaptations, enabling organisms to respond efficiently to their unique environmental and physiological challenges.
The diversity of calcium ATPases highlights their evolutionary adaptations, enabling organisms to respond efficiently to their unique environmental and physiological challenges.
Physiological Role of Calcium ATPase
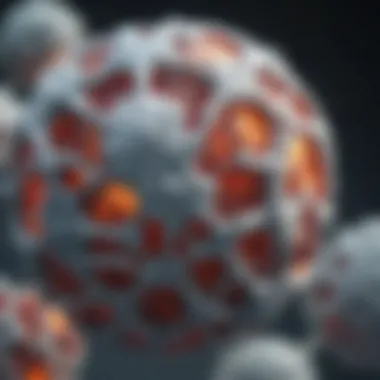
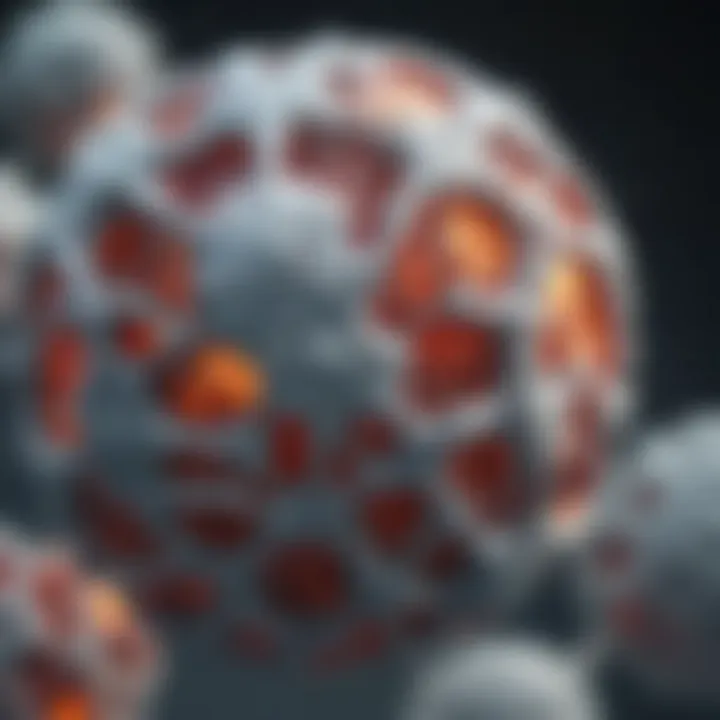
Calcium ATPase plays an essential role within the physiological framework of living organisms, influencing various cellular processes that are vital for maintaining biological homeostasis. Often regarded as the gatekeeper of calcium ion concentrations, this enzyme directly affects numerous physiological functions. It helps regulate the concentration of calcium ions in different compartments of the cell, which in turn is crucial for muscle contractions, neuronal signaling, and the overall balance of calcium within biological systems. Without its action, the finely tuned network of cellular operations would falter, emphasizing its significance in both health and disease.
Muscle Contraction Regulation
In the realm of muscle physiology, the role of calcium ATPase is pivotal. Muscle contractions are initiated by an influx of calcium ions, which stimulate muscle fibers to contract. Specifically, the sarcoplasmic reticulum calcium ATPase (SERCA) pumps calcium ions back into the sarcoplasmic reticulum after contraction, allowing the muscle to relax. If SERCA activity is compromised, it can lead to prolonged muscle contractions and even fatigue, akin to a car engine that won't turn off.
The energy generated by ATP hydrolysis is harnessed by calcium ATPase for this crucial pumping action. The rapid adjustments in calcium ions are what allow our muscles to behave responsively during athletic activities, from sprinting to lifting weights. Interestingly, studies indicate that upregulation of SERCA can improve muscle strength and performance, which could have therapeutic implications for athletes and individuals with muscle disorders.
Neuronal Function and Synaptic Activity
Calcium ATPase also plays a critical function in neuronal activity. Inside nerve cells, calcium ions act as signaling molecules. When an action potential arrives, calcium influx triggers neurotransmitter release, which enables communication between neurons. Here, plasma membrane calcium ATPase (PMCA) comes into play by exporting excess calcium from the cell, thus maintaining the balance crucial for preventing neurotoxicity.
Disruption in calcium handling can lead to severe consequences like synaptic dysfunction or even neurodegenerative diseases. Ca2+ homeostasis facilitated by calcium ATPase is thus central to synaptic plasticity—the ability of synapses to strengthen or weaken over time, a mechanism underlying learning and memory.
"Calcium ions serve as a double-edged sword; essential for signaling yet toxic in excess."
"Calcium ions serve as a double-edged sword; essential for signaling yet toxic in excess."
Calcium Homeostasis
Calcium ATPase is not just involved in muscle and neuronal activities; it is also vital for cellular calcium homeostasis. This pump facilitates the tightly controlled movement of calcium ions across cell membranes, keeping intracellular concentrations low while allowing for rapid increases when needed.
Dysregulation in calcium levels can lead to cellular turmoil. For instance, elevated calcium levels inside cells can trigger apoptosis or cell death, drawing attention to the pump’s role in mitigating such risks. Conversely, insufficient calcium can lead to impaired cellular functions and signaling failure. The interplay of calcium ATPases, alongside other ion transport mechanisms, keeps cells in a delicate balance akin to a well-tuned orchestra.
In summary, the physiological roles of calcium ATPase extend well beyond mere calcium regulation. It intricately weaves through the fabric of muscle function, neuronal signaling, and calcium balance within cells, underscoring its multifaceted importance in health and disease.
Calcium ATPase in Pathology
The role of calcium ATPase extends far beyond the simple management of calcium ions within cells. Its implications in various pathological conditions have garnered significant attention in recent research. Understanding how disruptions in calcium ATPase function can lead to disease progression aids not only in our comprehension of cellular mechanisms but also presents potential therapeutic avenues. When calcium homeostasis is affected, it can set off a domino effect, impacting everything from heart function to brain health.
Implications in Cardiovascular Diseases
Calcium ATPase is a key player in maintaining cardiac function. In a healthy heart, calcium ions trigger muscle contractions and are quickly removed to allow relaxation. When the plasma membrane calcium ATPase (PMCA) or the sarcoplasmic reticulum calcium ATPase (SERCA) are dysfunctional, the heart struggles to regulate calcium levels. Such malfunctions can contribute to conditions like heart failure and arrhythmias.
Research shows that altered expressions of the SERCA gene can decrease calcium uptake during diastole, the phase when the heart relaxes. This leads to inadequate heart filling, thus impairing cardiac output. For instance, in patients with heart failure, the SERCA2a pump is often downregulated.
"In dysfunctions related to calcium ATPase, the heart can lose its rhythm, leading to potentially life-threatening conditions."
"In dysfunctions related to calcium ATPase, the heart can lose its rhythm, leading to potentially life-threatening conditions."
Furthermore, some studies indicate that interventions enhancing calcium ATPase activity may alleviate symptoms in heart failure patients. Regarding hypertension, the relationship between calcium ATPase dysfunction and the regulation of vascular contractility cannot be overlooked.
Neurological Disorders
Calcium ATPase is crucial in the nervous system as it helps maintain calcium homeostasis within neurons. An overload of calcium ions can be toxic, leading to apoptosis or neuronal cell death. In diseases like Alzheimer's, elevated intracellular calcium levels have been linked to the degeneration of neurons. The role of specific calcium ATPases in synaptic transmission also cannot be dismissed, as they influence neurotransmitter release and uptake.
Research conducted on models of Alzheimer’s disease demonstrates that reducing calcium ATPase function exacerbates cognitive decline. Interestingly, cerebrovascular diseases such as ischemic stroke exhibit similar patterns of calcium dysregulation, implicating ATPase dysfunction in both neuronal injury and recovery phases post-stroke. By fostering an understanding of these pathways, new therapeutic targets emerge.
Cancer Development and Progression
Calcium ATPase’s role in cancer biology is a rapidly developing field. Aberrant calcium signaling is recognized as a contributor to tumorigenesis. Calcium ATPase serves as a regulator of intracellular calcium levels, and when impaired, it can result in uncontrolled cell proliferation.
For instance, elevated levels of SERCA have been observed in various tumor types, including breast cancer. On the contrary, reduced expression may correlate with other cancers like prostate cancer. This discrepancy illustrates the complexity of calcium ATPase's roles in distinct cancer environments. Moreover, targeting these pumps could lead to groundbreaking strategies for cancer treatment.
An intriguing concept includes the manipulation of calcium channels to promote apoptosis in highly proliferative cancer cells. By recognizing how calcium ATPases influence tumor growth and metastasis, researchers can better devise drugs that exploit these pathways to inhibit cancer progression.
Research Advances and Techniques
The exploration into the mechanisms governing calcium ATPase has gone hand in hand with advances in research methodologies. Whether discussing structural insights, biochemical pathways, or genetic underpinnings, each facet unveils the complexities of this enzyme and its profound implications in health and disease. Understanding these advances is essential for both researchers seeking to innovate in drug development and professionals striving to implement knowledge in clinical settings.
Structural Biology of Calcium ATPase
Structural biology plays a pivotal role in advancing our knowledge of calcium ATPase. By employing techniques like X-ray crystallography and cryo-electron microscopy, we can now visualize this enzyme in its various conformations. These methods allow us to see how calcium ATPase interacts with ions and ATP at the atomic level, opening the door to comprehending its precise mechanisms of action.
- Crystal Structures: Recent studies have revealed that calcium ATPase can exhibit multiple conformations, each tailored for specific functional states. This flexibility is crucial for its role in transporting calcium across membranes efficiently.
- Functional Domains: Understanding the specific domains of calcium ATPase elucidates how alterations in structure can lead to diverse functional outcomes. Research shows that different regions of the enzyme are responsive to various regulatory signals, underscoring the adaptability and complexity of calcium handling in cells.
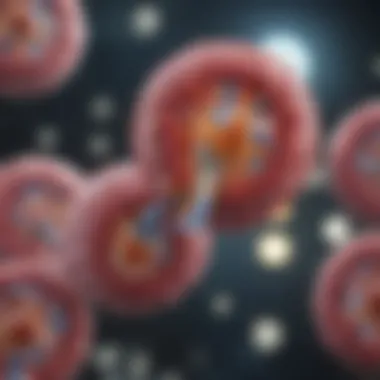
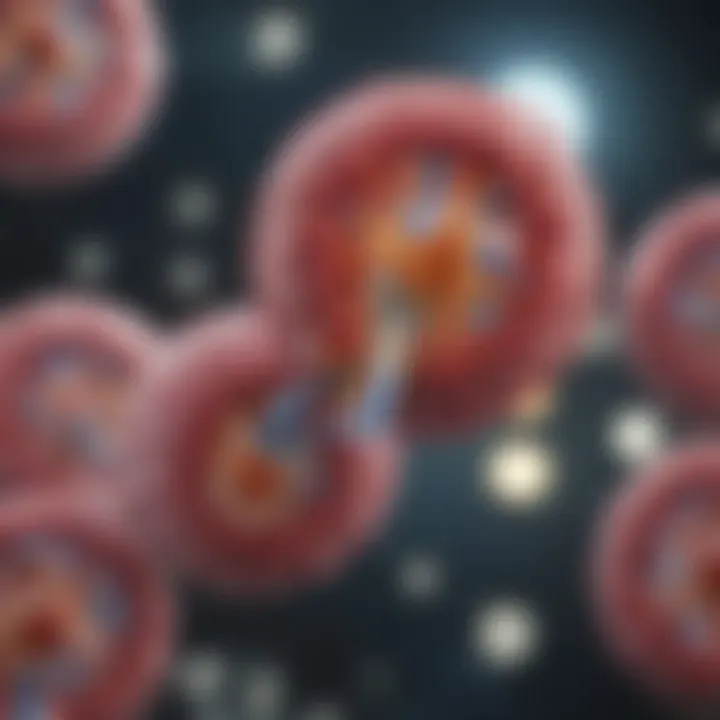
Indeed, structural biology not only enhances our comprehension but also guides rational drug design, targeting specific conformations of calcium ATPase to facilitate therapeutic interventions.
Biochemical Assays
Biochemical assays serve as a cornerstone in studying calcium ATPase functionalities. These assays allow scientists to quantify enzyme activity under various conditions, shedding light on regulatory mechanisms and physiological relevance.
Some key points in this area include:
- Activity Measurement: Using radioactive isotopes to trace ATP hydrolysis provides insights into how effectively calcium ATPase functions in transporting calcium ions.
- Inhibitor Studies: Compounds like thapsigargin are commonly used to inhibit ATPase activity. This helps researchers discern the roles of calcium ATPase in cellular processes and its potential interactions with other cellular machinery.
By establishing these assays, the scientific community can test hypotheses regarding calcium signaling pathways and even explore how pathological states alter enzyme behavior.
Genetic Approaches
Genetic techniques have transformed our understanding of calcium ATPase, allowing for exploration beyond biochemical assays and structural studies. Gene editing tools like CRISPR have unlocked new pathways for investigating the role of calcium ATPase in living organisms.
The significance of genetic approaches includes:
- Knockout Models: By creating knockout mice that lack specific isoforms of calcium ATPase, researchers can assess the direct physiological consequences of the enzyme's absence, providing valuable insights into disease mechanisms.
- Gene Expression Studies: Understanding how gene expression of calcium ATPase is regulated in different cell types can reveal potential targets for disease intervention, especially in cancer where calcium signaling is dysregulated.
Moreover, integrating genetic approaches with traditional biochemical assays can accelerate our understanding of how various genetic backgrounds affect calcium ATPase functionality.
By leveraging these research advances and techniques, we lay the groundwork for future discoveries that could lead to novel therapeutic strategies targeting calcium ATPase in various diseases. Understanding its multifaceted role implies a step towards healthier outcomes and more refined clinical practices.
Therapeutic Potential of Targeting Calcium ATPase
The significance of targeting calcium ATPase in therapeutic contexts is hard to overstate, especially given the enzyme’s multifaceted role in cellular homeostasis and signaling pathways. This section dives into the heart of how exploring calcium ATPase opens a realm of possibilities for drug discovery and development. When one thinks of therapeutic applications, it’s crucial to consider not only the direct effects of drug candidates on calcium ATPase but also the broader biological ramifications.
One of the most compelling aspects of calcium ATPase modulation is its implications for various pathologies. This enzyme effectively regulates calcium ion levels within cells, a process pivotal for normal function across different tissues including muscle, nerve, and endothelial cells. By keeping calcium homeostasis in check, calcium ATPase helps mitigate the risk of conditions such as hypertension, arrhythmias, and even neurodegenerative disorders.
Drug Development Strategies
When aiming to develop drugs that target calcium ATPase, researchers have to look at both the enzymatic function and the physiological context. There are a few strategic pathways worth considering:
- Direct Inhibitors and Activators: These compounds can either suppress or enhance the activity of calcium ATPase. For example, certain small molecules can be designed to fit into active sites, blocking calcium ion transport and subsequently impacting cellular signaling.
- Allosteric Modulators: These are subtle players that could adjust the enzyme’s activity without directly competing with the substrate. Enhancements here can provide a means to fine-tune calcium levels in a way that complements existing therapies.
- Nanotechnology Approaches: Targeting calcium ATPase through nanoparticle delivery systems can be revolutionary. For instance, these vehicles could release drugs in a controlled manner right at the cellular level where calcium flux needs to be regulated. This not only improves efficacy but also reduces side effects.
Existing pharmacological agents provide a template upon which new candidates can be designed. Digoxin, a well-known cardiac glycoside, exemplifies how calcium modulation can be utilized in treating heart failure by indirectly inhibiting Na+/K+ ATPase, which in turn influences calcium ATPase activity.
Challenges in Drug Design
While the therapeutic potential of calcium ATPase is substantial, it’s coupled with various challenges. Drug design is a tricky maze, and there are a number of considerations that must be tackled leading to fruitful outcomes:
- Selectivity and Side Effects: One critical concern is achieving selectivity. Many compounds might not distinguish between different ATPases, leading to off-target effects that could cause unintended consequences.
- Understanding Structural Complexity: The structural biology of calcium ATPase is intricate. Modeling the protein structure accurately for high-throughput screening presents hurdles, especially when considering conformational variability under different ionic conditions.
- Bioavailability Issues: Many potential therapeutic compounds face challenges in absorption and metabolism. If a drug targeting calcium ATPase can’t reach effective concentrations in tissues, it’s back to the drawing board.
- Regulatory Hurdles: The pathway from laboratory discovery to clinical application is fraught with regulatory scrutiny, demanding rigorous trials that demonstrate both efficacy and safety.
The pathway to effective therapies targeting calcium ATPase requires not only innovative science but also a nuanced understanding of physiology, pathology, and pharmacology.
The pathway to effective therapies targeting calcium ATPase requires not only innovative science but also a nuanced understanding of physiology, pathology, and pharmacology.
Closure and Future Directions
The exploration of calcium ATPase presents a significant avenue for both basic research and clinical application. Understanding its mechanics not only enhances our grasp of cellular functions but also provides insights into the implications of calcium dysregulation in various diseases. Thus, as we draw this discussion to a close, it’s crucial to reflect on two key areas: emerging trends in research and the impact on clinical practices.
Emerging Trends in Research
Research surrounding calcium ATPase has seen dynamic evolution in recent years. New techniques in structural biology, such as cryo-electron microscopy, are illuminating the intricate configurations of this enzyme in real-time. By observing calcium ATPase at various states of activity, researchers can delineate the pathway of calcium ions more accurately, shedding light on how external factors might influence this process. Additionally, advanced biochemical assays designed to measure activity under diverse environments are revealing how these ATPases respond to different physiological needs.
"Innovations in methodology are the keys to unraveling the depth of calcium ATPase functions and their role in health and disease."
"Innovations in methodology are the keys to unraveling the depth of calcium ATPase functions and their role in health and disease."
Engagement with genetic engineering tools, including CRISPR, has opened new doors to modulate calcium ATPase expression in model organisms. This not only furthers our understanding of physiological roles but also equips researchers with a mechanism to study potential therapeutic strategies against diseases linked to calcium irregularities.
Impact on Clinical Practices
The insights drawn from calcium ATPase studies directly correlate with clinical implications. For one, conditions like heart disease and certain neurological disorders exhibit a strong association with calcium mishandling, making calcium ATPase an enticing target for therapeutic strategies. If we can refine drugs that effectively modulate the activity of this enzyme, we might witness significant improvements in patient outcomes.
Moreover, as scientific knowledge grows, so does the potential for translating these findings into clinical therapies. Already, calcium ATPase inhibitors and activators are being investigated as part of treatment regimens for various conditions.
On a practical note, healthcare providers could implement screening for calcium ATPase activity in patients showing symptoms of related disorders, thus facilitating early diagnosis and tailored interventions.
In summary, the future of calcium ATPase research holds promise, presenting opportunities to develop novel treatments and refine existing approaches in clinical practice. The confluence of innovative research trends and the articulation of their clinical applications will undoubtedly shape a new frontier in the understanding and management of health-related issues connected to calcium regulation.