Cell Seeding: Techniques and Applications in Biomedicine
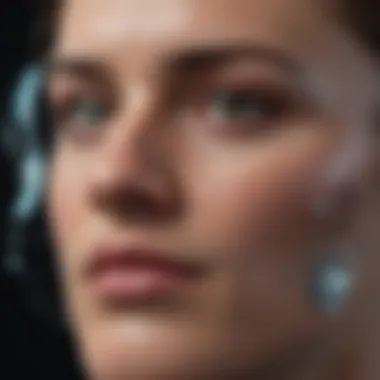
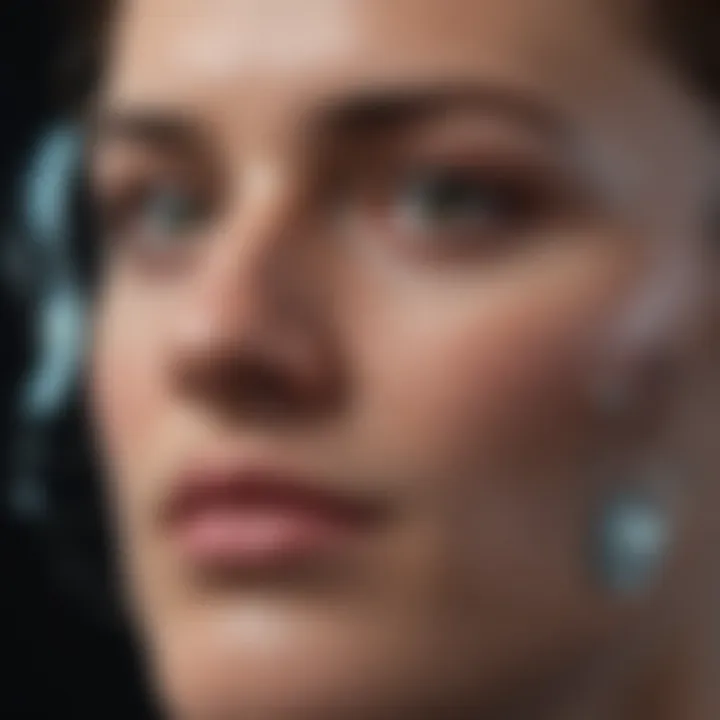
Intro
Cell seeding is a pivotal process in the realms of tissue engineering and regenerative medicine. It embodies the technique of introducing cells onto a support structure, known as a scaffold, which is essential for fostering cellular growth and subsequent development. As scientific inquiry continues to push the boundaries of our understanding, the significance of cell seeding cannot be overstated. Not only does it play a vital role in the formation of viable tissues, but it is also key to various applications spanning multiple scientific disciplines.
The methods of cell seeding vary widely, each having unique advantages and challenges. An exploration of these techniques reveals implications that extend into therapeutic applications, disease modeling, and even drug testing. This article aims to dissect these methodologies, assess their relative effectiveness, and delve into current trends shaping the future of cell culture and tissue engineering.
Key components such as biocompatibility and cell-to-cell communication will be examined in detail. The insights gained from this comprehensive examination will highlight how cell seeding is transforming biomedical research, addressing contemporary issues while anticipating the future of cell culture practices.
Key Concepts
Definition of the Main Idea
Cell seeding involves placing cells onto a substrate to initiate a process of cellular adhesion, proliferation, and differentiation. The scaffolds can be made from various materials such as hydrogels, ceramics, or biodegradable polymers. The choice of scaffold greatly influences the behavior and fate of cells during the growth period.
Overview of Scientific Principles
Understanding cell seeding requires a foundational grasp of several scientific principles. Key areas of focus include:
- Biocompatibility: The body's tolerance to the materials used in scaffolds.
- Cell viability: The ability of cells to survive and grow post-seeding.
- Cellular dynamics: The interactions and communications among different cell types.
Research indicates that the success of cell seeding hinges on optimizing these factors, which directly affects tissue development and functionality.
Current Research Trends
Recent Studies and Findings
Numerous studies have emerged in recent years that delve into innovative cell seeding techniques. For instance, recent explorations in 3D bioprinting have demonstrated remarkable advancements in precision and control during the cell placement process. The technology allows for the reconstruction of complex tissue architectures that better mimic natural conditions.
Significant Breakthroughs in the Field
Among the noteworthy breakthroughs is the development of microfluidic devices designed to improve cell distribution. These devices enhance uniformity in seeding, leading to better integration and functionality of the cells within the scaffold. The advent of these technologies exemplifies the ongoing evolution within the field and highlights the importance of interdisciplinary collaboration.
"Cell seeding not only shapes the future of regenerative medicine but also provides a platform for innovation in drug discovery and disease modeling."
"Cell seeding not only shapes the future of regenerative medicine but also provides a platform for innovation in drug discovery and disease modeling."
In summary, the journey into cell seeding is rich with scientific rigor and practical applications that continue to evolve. Understanding key concepts and staying abreast of current research trends is essential for students, researchers, educators, and professionals who aim to navigate the complexities of this vital area of study.
Prelude to Cell Seeding
Cell seeding plays a crucial role in the realm of tissue engineering and regenerative medicine. The process involves placing cells onto a scaffold. This is important for nutrition and support, promoting growth and development of tissues. Understanding cell seeding is essential not only for researchers but also for students and professionals who are involved in biomedical fields. The significance lies in the ability to create functional tissues that can be used for various applications, including drug testing and regenerative medicine.
Definition and Significance
Cell seeding refers to the method of introducing cells onto a scaffold or other substrate. This process is vital as it allows for the creation of tissue constructs that can mimic natural tissues, providing a platform for studying biological functions under controlled conditions. The significance of cell seeding is evident in its applications, which range from basic research to clinical therapies. Proper cell seeding techniques can enhance cell viability and functionality, which are critical for the success of any tissue engineering project.
Historical Context
Historically, the concept of cell seeding began with the early attempts at tissue culture in the mid-20th century. Many scientists have contributed to the evolution of cell seeding techniques. Notably, with advancements in technologies, methods have improved significantly. In the 1980s and 1990s, the focus shifted towards more defined culture conditions and the development of scaffolds that could support cell growth. As understanding of cell behavior evolved, scientists developed specific protocols to optimize cell seeding for different types of cells.
Cell Seeding in Modern Research
In modern scientific research, cell seeding has become an integral parameter for studying cellular interactions and tissue formation. It is employed across various fields, including regenerative medicine, drug development, and disease modeling. Researchers utilize advanced techniques such as bioreactors and microfluidics to enhance the efficiency and precision of cell seeding. Moreover, the current exploration into smart scaffolds is revolutionizing how cells are seeded, promoting better integration and functionality within the scaffold itself. Understanding these modern techniques will offer insights into the future of cell seeding and its underlying principles.
Fundamentals of Cell Seeding
Cell seeding forms the cornerstone of tissue engineering, serving not only as the initial step in populating scaffolds with cells but also defining the subsequent cellular behaviors and interactions. Understanding the fundamentals of cell seeding is crucial for researchers and practitioners in the field, as it directly influences the outcomes of tissue constructs. Key elements of cell seeding include the types of cells utilized, the preparation of scaffolds, and the culture conditions applied during the process. Each element plays a significant role in ensuring cell viability, functionality, and the success of engineered tissues.
Types of Cells Used
Choosing the appropriate type of cells for seeding is fundamental to achieving desired outcomes in tissue engineering. Cells can be broadly categorized into two groups: primary cells and cell lines. Primary cells are derived directly from tissues and maintain more natural characteristics; however, they have a limited lifespan. In contrast, established cell lines like HeLa or 3T3 can proliferate indefinitely but may lose some of their original properties over time.
When selecting a cell type, one must consider several factors:
- Functional Characteristics: The selected cells should match the specific functions of the tissue being engineered.
- Source Availability: Some cells are easier to obtain, such as adipose-derived stem cells, while others, like endothelial cells, might be more challenging to source.
- Regulatory Considerations: The use of certain cell types can have regulatory implications, particularly in clinical applications.
Preparation of Scaffolds
The preparation of scaffolds is integral to cell seeding, as the physical and chemical properties of the scaffold can dramatically affect cellular behavior. Scaffolds must possess specific characteristics to support cell attachment, proliferation, and differentiation. Factors to consider include:
- Porosity: Scaffolds should have an appropriate level of porosity to facilitate nutrient and oxygen transport.
- Surface Chemistry: Coating or modifying scaffold surfaces can enhance cell adhesion and proliferation.
- Mechanical Properties: Scaffolds need to mimic the mechanical environment of the target tissue to promote functional integration.
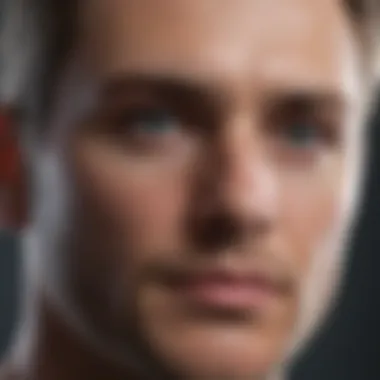
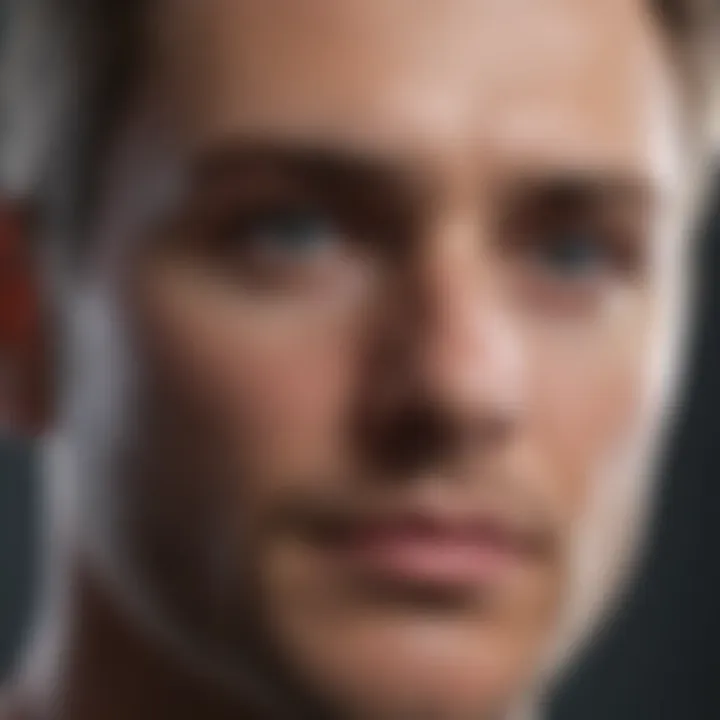
These considerations ensure that the cells can interact favorably with their environment, encouraging desired tissue growth.
Culture Conditions
Culture conditions encompass a range of environmental factors including temperature, pH, oxygen levels, and the composition of the culture medium. These conditions must be tightly controlled to optimize cell growth and metabolism.
Important aspects of culture conditions include:
- Medium Composition: The choice of a basal medium, like DMEM or RPMI, along with supplements such as fetal bovine serum (FBS), influences cell viability.
- Oxygen Concentration: Cells may require specific oxygen levels, as hypoxia can alter cellular behaviors.
- Temperature and pH: Standard conditions usually set at 37°C and a pH range of 7.2 to 7.4, but some specific cell types might need deviations.
Cell behavior is significantly affected by these parameters, hence understanding them is essential for achieving successful cell culture outcomes.
"Optimizing each of these fundamental elements not only enhances the efficiency of cell seeding but also increases the viability of engineered tissues, making it vital to their success in regenerative medicine."
"Optimizing each of these fundamental elements not only enhances the efficiency of cell seeding but also increases the viability of engineered tissues, making it vital to their success in regenerative medicine."
Techniques for Cell Seeding
The Techniques for Cell Seeding form a crucial part of the understanding of how cells can be applied in tissue engineering and regenerative medicine. These methods directly influence cell distribution, viability, and integration into the scaffolds or substrates being used. Choosing the right technique can enhance the outcomes of any experiment or application in these fields. Each of the techniques offers specific advantages and challenges that researchers must consider carefully. Understanding the intricacies of these methods supports advancements in biomedical applications.
Static Seeding
Static seeding involves placing a predetermined number of cells onto the surface of a scaffold or culture dish without applying any external forces. It is a straightforward method and has several advantages. One key aspect is its simplicity and low cost, making it accessible for labs with tight budgets. Researchers can control the number of cells seeded with relative ease, and the technique is adaptable for various types of scaffolds. However, there are limitations. This method often results in uneven cell distribution and may not adequately support cell proliferation over time. Static seeding is best suited for preliminary studies where detailed control of the environment is not as critical.
Dynamic Seeding Methods
Dynamic seeding methods introduce movements or conditions that can promote better cell attachment and distribution. Traditionally, two methods have gained particular attention: Bioreactors and Microfluidic systems.
Bioreactors
Bioreactors offer a controlled environment for cell growth and can effectively simulate physiological conditions. They provide a continuous supply of nutrients and remove waste, which is crucial for maintaining cell viability. One key characteristic of bioreactors is their ability to create shear stress, which can enhance cell attachment and proliferation. This feature makes bioreactors a popular choice for large-scale tissue engineering efforts. They can accommodate a variety of cell types and improve the overall yield of cells produced. However, the complexity and cost of bioreactors are significant drawbacks. They require careful monitoring and maintenance to ensure optimal performance.
Microfluidic Systems
Microfluidic systems represent an innovative approach to cell seeding by manipulating minute volumes of fluids to control the microenvironment. These systems allow precise control over cell placement and provide a platform for studying cellular interactions on a small scale. One of the key advantages is the minimization of reagents used, contributing to cost-effectiveness and reduced waste. Microfluidic systems allow researchers to conduct experiments with high-throughput potential, which facilitates the exploration of different parameters efficiently. However, the complexity of designing these systems can be a challenge, and not all cell types may thrive in such confined spaces.
Hanging Drop and Rotating Systems
The hanging drop technique involves suspending a droplet containing cells above a surface, allowing cells to aggregate and form spheroids. This method supports natural cell cell interactions and is particularly effective for studies aimed at mimicking tissue structures. Spheroids can offer a more physiologically relevant model than monolayers. Conversely, rotating systems facilitate the cultivation of cells in a 3D environment, providing dynamic conditions that promote better nutrient diffusion and oxygen delivery.
Electrospinning Techniques
Electrospinning allows for the creation of nanofibrous scaffolds that can closely mimic the extracellular matrix. This technique uses electrostatic forces to draw polymers into fine fibers, which can then be seeded with cells. The unique feature of electrospinning is its ability to create structures with high surface area and tunable porosity. This enhances cell attachment and growth while providing physical cues for cellular behaviors. However, challenges remain, such as the need for uniformity and the potential toxicity of certain used solvents. Despite these challenges, electrospinning techniques are seen as a viable option for the future of scaffold fabrication in tissue engineering.
"The selection of seeding techniques significantly influences the outcomes in tissue engineering, affecting everything from cell viability to scaffold integration. Each method has distinct applicability depending on research goals."
"The selection of seeding techniques significantly influences the outcomes in tissue engineering, affecting everything from cell viability to scaffold integration. Each method has distinct applicability depending on research goals."
In summary, selecting an ideal cell seeding technique is paramount for effective tissue engineering. Each method varies in its complexity, cost, and biological relevance, stressing the need for thoughtful consideration in experimental design.
Evaluating Cell Seeding Efficiency
Evaluating the efficiency of cell seeding is critical for advancing the applications of tissue engineering and regenerative medicine. The ability to determine how well cells integrate into scaffolds and exhibit functional behavior is paramount. Efficient cell seeding not only enhances tissue construct viability but also plays a vital role in the eventual success of implants and therapeutic interventions. This evaluation encompasses various assessments that reflect the viability, proliferation, and matrix interactions of the seeded cells.
Cell Viability Assessment
Cell viability assessment is an essential method for determining the proportion of living cells within a population after the seeding process. Accurate measurements of cell viability can reveal the effectiveness of different seeding techniques and conditions. Commonly used assays include MTT, and trypan blue exclusion methods, among others.
- MTT Assay: This colorimetric assay measures the metabolic activity of live cells. Viable cells reduce MTT to formazan, which can be quantified spectrophotometrically. This method excels in providing a quantitative evaluation of cell viability under various conditions.
- Trypan Blue Exclusion: This method relies on the principle that live cells exclude the dye while dead cells take it up. It offers a straightforward means of assessing cell survival and is particularly useful in quick evaluations.
By employing these assays, researchers can ensure that the seeded cells retain their viability post-seeding, which is crucial for the subsequent growth and functionality of engineered tissues.
Proliferation Studies
Proliferation studies are vital for assessing the growth rate of the seeded cells over time. These investigations are essential for understanding how well cells can expand within the engineered structure after they have attached. Assessing cell proliferation can indicate whether the seeding conditions support adequate nourishment and spatial arrangement in the matrix.
Techniques often used in proliferation studies include:
- BrdU Assay: This method labels newly synthesized DNA, thus allowing for the detection of proliferating cells.
- Flow Cytometry: This technique can analyze cell cycle stages and provide insight into the overall proliferation rate across a population.
These studies facilitate an understanding of how seeded cells interact with their environments and adapt to their scaffolds, informing future modifications and techniques for improved outcomes.
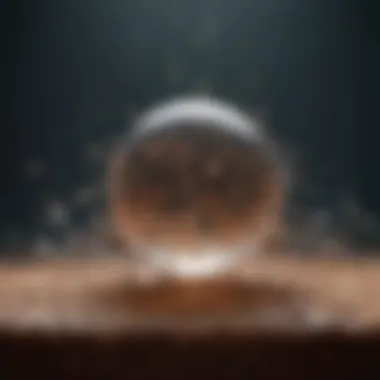
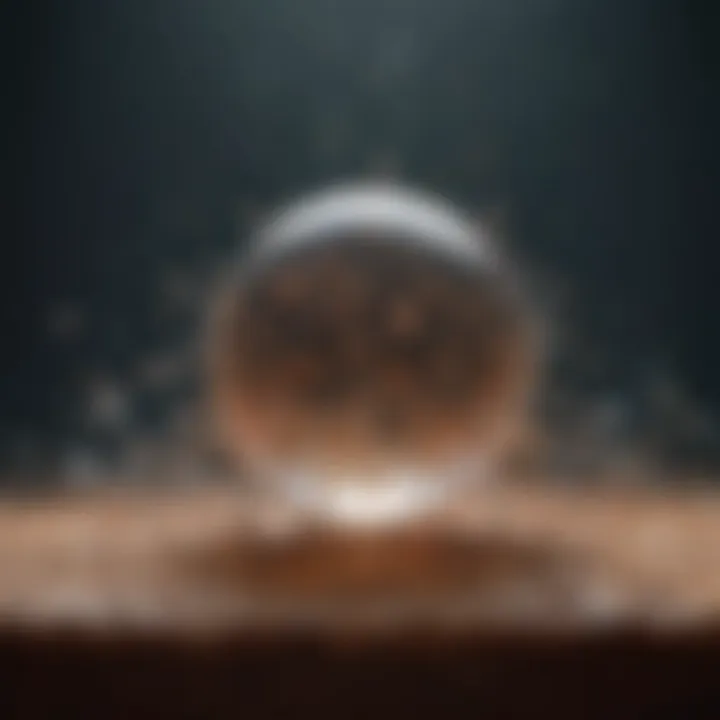
Matrix Interaction Analysis
Matrix interaction analysis focuses on the relationship between seeded cells and the extracellular matrix or scaffold. Understanding these interactions is essential for evaluating the construct's biological performance. Effective cell-matrix interactions enhance cell attachment, migration, and ultimately, tissue integration.
Several methods can be utilized for matrix interaction evaluation:
- Immunostaining: This technique can visualize the expression of specific markers indicative of cell-matrix adherence and interactions.
- Biomechanical Testing: Assessing the mechanical properties of the matrix can give insights into how well seeded cells are contributing to the tissue construct.
This analysis provides crucial data that influences the design and optimization of scaffolds and seeding strategies.
The capacity to evaluate cell seeding efficiency can directly impact the translational success of regenerative therapies and preclinical studies.
The capacity to evaluate cell seeding efficiency can directly impact the translational success of regenerative therapies and preclinical studies.
Through these assessments, researchers and practitioners can refine methods and enhance the quality of tissue engineering outcomes, ultimately leading to breakthroughs in personalized medicine and health care.
Applications of Cell Seeding
Cell seeding is a crucial aspect of modern biomedical research, with broad implications across several fields. This process is particularly significant when developing tissues, testing drugs, modeling diseases, or advancing regenerative medicine. The effectiveness of cell seeding protocols directly impacts research outcomes and the clinical viability of engineered tissues. Therefore, understanding various applications can provide insights into how to optimize these methods further.
Tissue Engineering
Tissue engineering relies heavily on cell seeding to create functional biological constructs. The aim is to mimic natural tissues and organs for transplantation or repair. In this context, the choice of cells and scaffolds is critical. It is typically essential to choose cells that are suitable for the specific tissue type being created. For instance, stem cells are often used due to their potential to differentiate into various cell types.
Scaffolds are designed to provide a three-dimensional support structure. They not only help anchor the cells but also facilitate nutrient diffusion and waste removal. Polymers, hydrogels, and ceramics are common materials used in scaffold fabrication.
Benefits of Effective Cell Seeding in Tissue Engineering:
- Enhanced cell attachment and proliferation.
- Improved tissue integration when implanted.
In summary, successful cell seeding in tissue engineering can lead to the creation of viable tissues that are critical for regenerative therapies.
Drug Testing and Development
The pharmaceutical industry often uses cell seeding to assess drug efficacy and safety. Cell-based assays can simulate human responses to drugs better than traditional methods, like animal testing. By seeding specific types of cells, researchers can study drug interactions and effects in a controlled environment.
For example, cancer research uses various cancer cell lines seeded in specific arrangements to observe responses to therapeutic compounds. Whole-cell formats, spheroids, or organoids derived from seeded cells provide more accurate data on how prospective drugs affect human tissues.
Considerations in Drug Testing:
- Selection of the right cell line is crucial for relevance.
- The culture conditions must mimic in vivo environments to ensure reliability.
In drug testing, cell seeding methods are vital for discovering effective treatments and understanding their action mechanisms at cellular levels.
Disease Modeling
Cell seeding plays a key role in disease modeling as well. Researchers are able to reproduce actual disease conditions by using patient-derived cells or stem cells to create models of specific diseases. This approach allows for the investigation of disease processes in vitro and the development of targeted studies for treatments.
Using advanced techniques like organ-on-a-chip, specific cell types are seeded in microfluidic environments to organize interactions similar to those in the human body.
Key Aspects of Disease Modeling through Cell Seeding:
- Patient-specific cells facilitate personalized studies.
- Allows for testing of multiple therapeutic approaches in one setting.
Through effective cell seeding, scientists enhance our understanding of diseases and provide innovative avenues for therapeutic intervention.
Regenerative Medicine
In regenerative medicine, cell seeding is essential to repair or replace damaged tissues and organs. The use of stem cells, for instance, offers exciting possibilities for treating conditions such as spinal cord injuries, heart diseases, or systematic degenerative disorders. In this case, the compatibility and functionality of the seeded cells are paramount.
Biomaterials that are bioactive or biodegradable can serve as scaffolding for cell seeding. For successful integration and functionality in the host environment, these materials need to facilitate cell attachment and promote cellular functions.
Challenges in Regenerative Medicine:
- Ensuring the long-term survival of seeded cells in vivo.
- Integrating newly formed tissues with the existing cellular systems of the patient.
The implications of successful cell seeding in regenerative medicine are vast, potentially leading to breakthroughs in treatment strategies for chronic diseases.
Effective cell seeding is not just for research; it is a linchpin in creating a future where damaged tissues may be overcome through engineered solutions.
Effective cell seeding is not just for research; it is a linchpin in creating a future where damaged tissues may be overcome through engineered solutions.
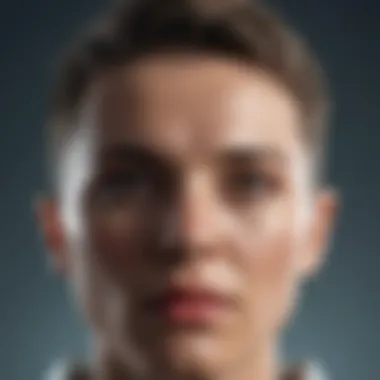
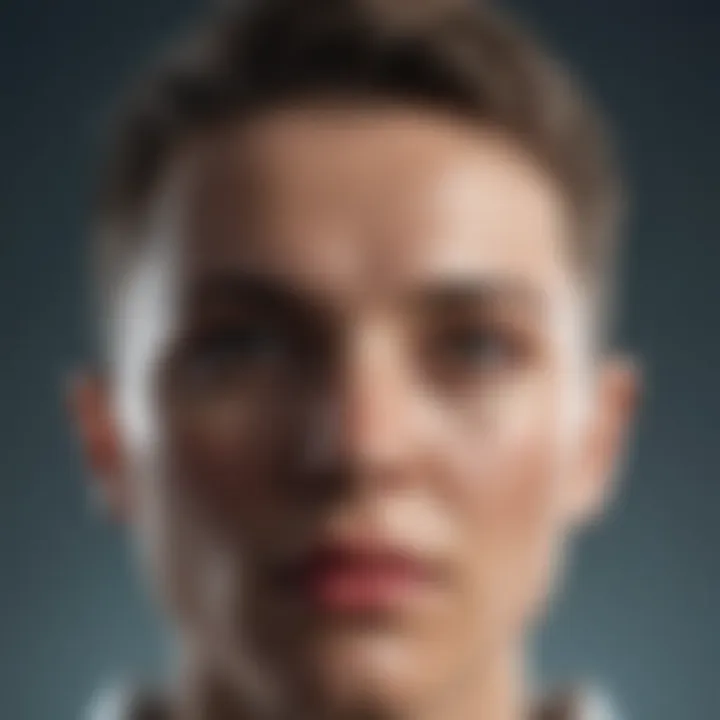
Challenges and Limitations
In cell seeding, significant challenges and limitations can inhibit the translational applications of the technology in tissues engineering and regenerative medicine. Understanding these factors is essential for researchers and practitioners aiming to optimize cell seeding techniques and improve outcomes in biomedical applications.
Biocompatibility Issues
Biocompatibility is a fundamental aspect in cell-seeding processes. Material selection for scaffolds must ensure that the cells adhere, grow, and function correctly. Commonly used materials can evoke immune responses or stimulate inflammatory processes. This can lead to cell death or dysfunction, ultimately impairing the intended purpose of the engineered tissue.
Moreover, factors like surface chemistry, mechanical properties, and degradation rates of scaffold materials significantly influence cell behavior. For example, a study indicates that poly(lactic-co-glycolic acid) exhibits differing degrees of biocompatibility depending on its formulation and processing methods.
The complexity in achieving optimal biocompatibility underscores the need for extensive screening of materials before utilization. Continuous improvements in material design that favor cell growth are necessary to tackle these issues.
Scalability Concerns
Another notable challenge is the scalability of cell seeding processes. While laboratory-scale experiments may yield promising results, the translation to larger-scale production often encounters obstacles. The uniformity in cell distribution across large scaffolds presents a significant hurdle.
Most current techniques work well at small scales. However, as the scale increases, controlling environmental parameters such as nutrient flow, oxygen levels, and waste removal becomes complicated. Bioreactors have been proposed to mitigate this. Still, they require sophisticated engineering and are often cost-prohibitive.
Scalability remains a pressing concern as research moves towards practical applications in clinical settings. Developing methodologies that ensure reproducibility and cost-effectiveness in large-scale production can significantly enhance the viability of cell-seeding methods.
Cell Type Specificity
Cell type specificity also poses a challenge in seeding applications. Different types of cells have distinct requirements for attachment, survival, and proliferation. For instance, mesenchymal stem cells and endothelial cells behave differently in the same environment. This difference can lead to inconsistent results when using a singular approach for diverse cell types.
Moreover, optimizing conditions for one cell type may create an unfavorable environment for another. This specificity reiterates the need for tailored approaches in cell seeding. Researchers often rely on a combination of techniques to accommodate the unique needs of various cell types. This adjustment may include altering scaffold characteristics or modifying culture conditions, complicating the process further.
In summary, the challenges of biocompatibility, scalability, and cell type specificity play crucial roles in the effectiveness of cell seeding. These factors must be carefully managed to improve outcomes in biomedical applications.
In summary, the challenges of biocompatibility, scalability, and cell type specificity play crucial roles in the effectiveness of cell seeding. These factors must be carefully managed to improve outcomes in biomedical applications.
Future Directions in Cell Seeding Research
The field of cell seeding is evolving rapidly, reflecting advancements in technology and a deeper understanding of biological processes. This section explores the future directions in cell seeding research, emphasizing the significance of emerging technologies, their applications in personalized medicine, and the potential of artificial intelligence integration. This is critical as it aligns with contemporary challenges and seeks to enhance the effectiveness of cell seeding methodologies.
Emerging Technologies
New technologies are shaping the landscape of cell seeding. Innovations such as 3D bioprinting and microfabrication techniques are creating scaffolds with increased complexity and functionality.
- 3D Bioprinting: This method allows for precise placement of cells in three-dimensional spaces, closely mimicking natural structures. This advancement can lead to better integration of seeded cells within tissues.
- Microfluidic Systems: These platforms enable precise control of the cellular environment, facilitating the study of cell behavior in conditions that replicate physiological settings. In turn, this can improve the accuracy of cell responses during experiments.
Each of these technologies not only enhances the seeding process but also provides insights into cellular interactions, aiding the development of more effective therapeutics.
Personalized Medicine Applications
As medicine leans towards personalization, the role of cell seeding becomes even more relevant. Personalized medicine relies on tailored approaches for treatments, and cell seeding is vital for developing patient-specific therapies, particularly in regenerative medicine.
- Tissue Constructs: By utilizing cells derived from patients, researchers can create tissue constructs that reflect the unique biology of individuals. This is crucial for understanding disease mechanisms and testing drug responses that are specific to a patient's cellular makeup.
- Advancement in Regenerative Techniques: Leveraging personalized cell seeding can improve outcomes in treatments for conditions such as heart disease or spinal cord injuries, where specific tissues need to be regenerated.
These advancements signal a shift toward individualized treatment strategies, making the research in personalized medicine highly promising.
Integrating AI in Cell Seeding Processes
The incorporation of artificial intelligence into cell seeding processes holds substantial promise. AI can analyze vast datasets, offering insights that inform experimental designs and optimize protocols for cell seeding.
- Data Analysis: AI algorithms can rapidly assess cell growth and viability, identifying the most effective conditions for culture and seeding.
- Predictive Modeling: Machine learning can help predict how different cell types will behave under various seeding conditions, thus enhancing the efficiency of experiments.
By harnessing AI, researchers can make informed decisions rapidly, greatly speeding up the research workflow in cell seeding and related applications.
By harnessing AI, researchers can make informed decisions rapidly, greatly speeding up the research workflow in cell seeding and related applications.
The End
The conclusion of this article serves as a crucial component in encapsulating the multifaceted nature of cell seeding. It synthesizes the various discussions surrounding techniques, applications, and future prospects in the domain. Understanding the significance of cell seeding is essential for both researchers and practitioners in biomedical fields, particularly in tissue engineering and regenerative medicine. This process is not merely technical; it embodies the intersection of biology, technology, and innovation.
Summary of Key Insights
Throughout the article, several key insights have emerged regarding cell seeding. Key points include:
- Diversity of Techniques: Different seeding methods, such as static seeding and dynamic systems, each have unique advantages that can be applied depending on the specific research goals.
- Importance of Cell Interaction: Successful cell growth is highly dependent on how cells engage with the scaffold and among themselves, highlighting the need for careful consideration of cell type and environmental conditions.
- Applications Across Disciplines: Cell seeding is applicable in numerous areas, from tissue engineering and drug testing to disease modeling and regenerative therapies.
- Challenges to Address: Issues such as biocompatibility and scalability continue to pose challenges in practical applications, necessitating ongoing research and refinement of techniques.
The synthesis of these insights underscores the transformative potential of cell seeding in advancing biomedical research and therapeutic solutions.
Implications for Future Research
Looking ahead, the implications of findings related to cell seeding are profound. Future research should aim to:
- Explore Emerging Technologies: As technology advances, integrating innovative approaches, such as advanced bioreactors and AI-driven methods, will likely optimize cell seeding processes and outcomes.
- Focus on Personalized Medicine: Tailoring cell seeding techniques to accommodate individual patient profiles may enhance the effectiveness of regenerative therapies.
- Address Limitations: Continued investigation into biocompatibility issues and scalability challenges must be prioritized to facilitate broader adoption of cell seeding strategies in clinical settings.
In summary, the future of cell seeding research remains promising. By addressing current challenges and leveraging emerging technologies, the field is poised for significant advancements that could reshape therapeutic practices in the years to come.