Construction of cDNA: Techniques and Innovations
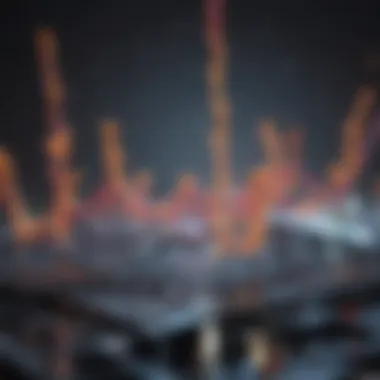
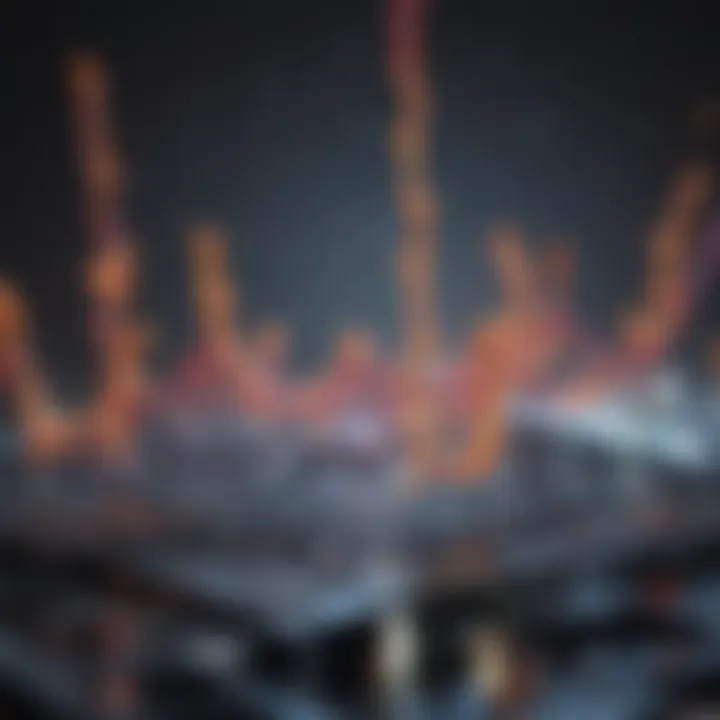
Intro
The journey of understanding genetic expression has been revolutionized by the advent of complementary DNA, commonly known as cDNA. In essence, cDNA serves as a mirror reflection of the RNA from which it originates, enabling researchers to dive deep into the complexities of gene activity. This process of constructing cDNA is not merely a step in a lengthy protocol; it is a gateway to exploring how genes function, how they turn on and off, and how their expression is influenced by various stimuli.
Within the realm of molecular biology, where every detail matters, the construction of cDNA plays a consequential role. It paves the way for various applications, from understanding diseases to developing therapies. Through this article, we will embark on an exploration of the techniques, applications, and innovations that underscore the significance of cDNA. Here, the spotlight will not just shine on methodologies, but also on how these techniques contribute to our grasp of biology in a profound way.
By laying out the nuts and bolts of cDNA synthesis, we aim to demonstrate why cDNA is an essential tool in both academic and clinical research. Let’s delve into the key concepts that will frame our understanding of this intricate process.
Understanding cDNA
The construction of complementary DNA (cDNA) is a fundamental concept in molecular biology that serves as the cornerstone for many applications across a range of scientific disciplines. Gaining a solid grasp of cDNA is essential for students, researchers, and professionals who wish to navigate the increasingly complex arena of genetic studies and biotechnology.
cDNA provides a bridge between RNA and DNA, allowing scientists to understand gene expression by creating a stable, double-stranded DNA version of a single-stranded RNA template. This transformation opens doors to numerous practical applications, from cloning genes to developing expression vectors used in various studies.
Definition and Characteristics of cDNA
Complementary DNA (cDNA) is synthesized from a messenger RNA (mRNA) template through a process called reverse transcription. This process converts the mRNA back into a form of DNA, which serves as a durable copy of the genetic information originally present in the RNA. One of the defining characteristics of cDNA is that it is lacking introns; because it is derived from mRNA, it reflects only the expressed portions of a gene, making it a valuable tool for studying gene expression levels and patterns. By focusing on these expressed genes, researchers are better able to understand the roles they play in cellular processes, disease states, and developmental changes.
cDNA is typically produced using reverse transcriptase enzymes that catalyze the synthesis of DNA from RNA. These enzymes vary in their properties and efficiency, which can significantly impact the quality of the cDNA produced. Thus, the choice of reverse transcriptase and conditions may determine the success of downstream applications like cloning or sequencing.
In addition to its synthesis, cDNA is characterized by its stability and ability to easily multiply during amplification processes, such as polymerase chain reaction (PCR). This robustness ensures that researchers can generate enough material for their experiments, which is especially important when working with limited RNA samples.
Comparison between cDNA and Genomic DNA
When comparing cDNA to genomic DNA, several key differences emerge. While both are forms of DNA, their origins, structure, and purpose vary noticeably.
- Source: cDNA is synthesized from mRNA, reflecting only those genes expressed in a given context. In contrast, genomic DNA represents an organism's complete genetic material, including exons, introns, and regulatory sequences.
- Structure: Since cDNA arises from mRNA, it contains only the coding sequences (exons) and lacks the non-coding intronic regions found in genomic DNA. This makes cDNA ideal for studying active gene expression without the noise of inactive or spliced-out regions.
- Function: The primary role of cDNA is to serve as a stable template used in experimental procedures, such as cloning or analysis of gene expression. Genomic DNA, on the other hand, is a more comprehensive entity that encompasses an entire organism's genetic blueprint, often with interests in both functional and regulatory elements leading to phenotypes.
cDNA, in its essence, simplifies the complexities encapsulated in genomic DNA into a manageable form that focuses exclusively on what is actively being expressed in specific cellular environments. This distinction highlights the significance of cDNA in studies related to functional genomics and the role of genes in various biological contexts.
"Understanding the nature of cDNA versus genomic DNA is pivotal for interpreting experimental results, paving the way for innovations and breakthroughs in biomedical research."
"Understanding the nature of cDNA versus genomic DNA is pivotal for interpreting experimental results, paving the way for innovations and breakthroughs in biomedical research."
Through this elucidation of cDNA and its comparative aspects with genomic DNA, we lay a foundation for delving deeper into methodologies for constructing cDNA and exploring its applications in the fields of research and clinical practice.
Importance of cDNA in Molecular Biology
cDNA, or complementary DNA, plays a crucial role in molecular biology, particularly in elucidating the mechanisms behind gene expression. Its construction is a fundamental process, allowing researchers to convert mRNA information into a stable form that can then be studied in depth. By understanding how genes are expressed within various biological contexts, scientists can unlock insights into cellular functions, disease mechanisms, and potential therapeutic targets.
One significant advantage of cDNA is its ability to reflect the actively expressed genes in a given cell or tissue type at a specific time. This provides a snapshot of gene activity, which is invaluable for studies investigating differential gene expression. With the advent of high-throughput sequencing technologies, cDNA has become even more pivotal. Researchers can generate comprehensive profiles of gene expression across different conditions, paving the way for discoveries in fields ranging from cancer research to regenerative medicine.
Furthermore, the versatility of cDNA is a game changer in functional genomics. By utilizing cDNA libraries, scientists can archive and explore the vast repertoire of expressed genes. These libraries serve as vital resources for understanding the interactions between various genes and their protein products. In therapeutic contexts, cDNA is instrumental in developing gene therapies that seek to correct malfunctioning genes or compensate for lost functions due to genetic disorders.
Role of cDNA in Gene Expression Studies
The role of cDNA in gene expression studies cannot be overstated. By converting RNA molecules back into DNA, researchers obtain a reliable template for analysis. This transformation effectively sidesteps many of the challenges associated with direct RNA manipulation, primarily due to RNA's inherent instability and susceptibility to degradation.
In typical gene expression experiments, mRNA is isolated from cells and then reverse transcribed to form cDNA. This cDNA can be amplified through PCR, enabling the detection of low-abundance mRNAs. Here are a few key aspects of cDNA's role in gene expression studies:
- Quantitative Analysis: cDNA allows for quantitative polymerase chain reaction (qPCR), enabling precise measurements of gene expression levels.
- Comparative Evaluations: By synthesizing cDNA from mRNA isolated under various experimental conditions, researchers can compare expression levels and identify changes linked to specific stimuli or treatments.
- Functional Insights: Analyzing the cDNA helps unravel the functional implications of expressed genes, assisting in understanding pathology and guiding therapeutic development.
Auhtoritative studies often highlight the reliance on cDNA, underscoring its significance in mapping gene regulatory networks. When coupled with advanced bioinformatics tools, cDNA has the potential to uncover intricate relationships between genes, environmental factors, and cellular responses. Thus, cDNA stands as a linchpin in modern bio-research frameworks, broadening the horizon of molecular biology.
cDNA in Functional Genomics
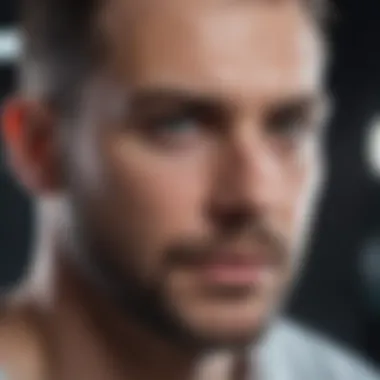
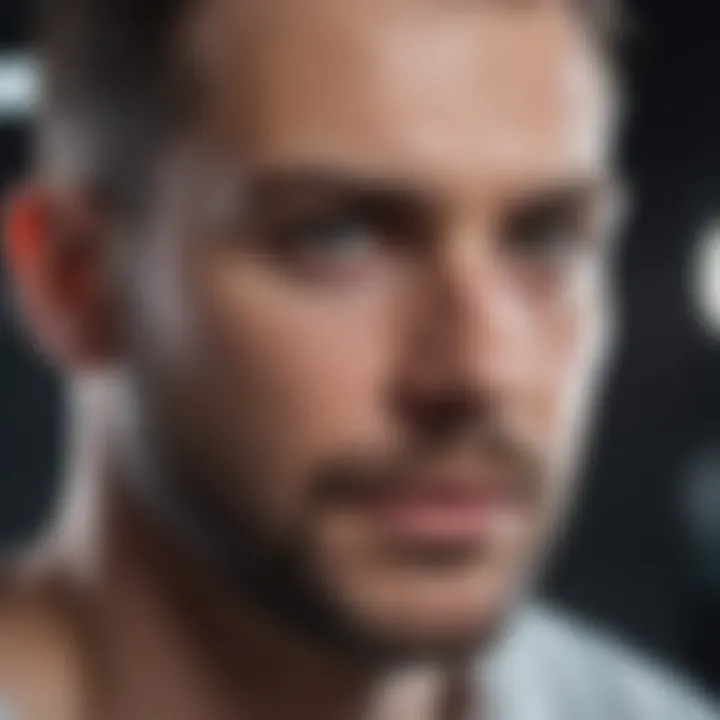
In the realm of functional genomics, cDNA emerges as a foundational component for studying gene functions systematically. The ability of cDNA to represent expressed genes in certain cells enables investigations into their roles in various biological processes. By utilizing cDNA, researchers can dissect gene interactions and evaluate the consequences of disrupting specific genes.
Constructing cDNA libraries not only serves as a method for storing genetic information but also facilitates high-throughput screening. Here are some pertinent applications:
- Gene Cloning: cDNA libraries provide a tool for cloning genes of interest, enabling detailed functional studies of these genes in model organisms.
- Expression Profiling: Genetic profiling using cDNA allows for the identification of gene clusters involved in specific disease phenotypes, leading to targeted research.
- Functional Annotation: The data derived from cDNA can be instrumental in annotating functions of previously unexplored or poorly understood genes.
Moreover, as technology advances, so do the methodologies surrounding cDNA construction. Innovations aimed at improving cDNA synthesis and analysis techniques are continually shaping the landscape of functional genomics, ensuring that cDNA remains a vital resource for unlocking the complexities of gene functions.
“The journey of understanding biology is ever-evolving, and cDNA is a cornerstone that anchors many of these explorations.”
“The journey of understanding biology is ever-evolving, and cDNA is a cornerstone that anchors many of these explorations.”
Methodologies for cDNA Construction
The process of constructing complementary DNA (cDNA) is a cornerstone in molecular biology. It serves not only as a pivotal tool for studying gene expression but also advances our understanding of genetic elements across various fields. The methodologies used for cDNA construction have evolved over time, showcasing a mix of both traditional and innovative approaches.
Reverse Transcription Processes
Reverse transcription is the first and perhaps the most crucial step in cDNA construction. This process involves synthesizing cDNA from an RNA template. Here we delve into the enzymatic mechanisms and various types of reverse transcriptases that make this process possible.
Enzymatic Mechanisms
The heart of reverse transcription lies in the action of enzymes, particularly reverse transcriptases. These enzymes catalyze the conversion of RNA into cDNA by incorporating complementary nucleotides. One key characteristic of these mechanisms is their ability to exhibit high fidelity, which is vital when one aims to produce accurate copies of RNA sequences. The accuracy here is crucial, as slight misrepresentations can lead to downstream errors in gene expression studies.
One of the beneficial attributes is the capability of these enzymes to work with impure RNA samples, making the process robust. However, it can have its downsides too; for example, a tendency to create errors while synthesizing cDNA from degraded RNA. The unique feature of enzymatic mechanisms lies in their adaptability to various conditions, allowing researchers to optimize the process for specific applications.
Types of Reverse Transcriptases
When it comes to selecting a reverse transcriptase, researchers face a plethora of options. Each type, like M-MLV or AMV reverse transcriptases, come with their own unique attributes. A notable feature of these transcriptases is their varying processivity and thermostability. This means some enzymes perform better at higher temperatures, which could be advantageous in certain experimental conditions.
The advantage of these specialized transcriptases is that they significantly increase the yield of cDNA, particularly in cases with lower concentrations of starting RNA. On the flip side, certain types may exhibit less efficiency in the presence of inhibitors, which could complicate matters further. Choosing the right type becomes essential given the diversity in sample quality.
Template Selection and Preparation
Selecting a suitable RNA template is another crucial aspect of cDNA synthesis. It’s where quality reigns supreme at every inch of the process. From RNA extraction techniques to the thorough quality assessment of RNA, every step is significant in ensuring reliable results.
RNA Extraction Techniques
One cannot underestimate the importance of RNA extraction techniques in this context. These techniques are designed to isolate RNA from a variety of biological samples as efficiently as possible. A prominent characteristic of these techniques, like TRIzol extraction, is their ability to yield high-purity RNA. This is vital for downstream applications where contamination could lead to unreliable data.
These techniques are advantageous as they provide relatively quick turnaround times. However, they come with challenges related to the potential degradation of RNA during the extraction process, which can compromise the quality of the resultant cDNA. Utilizing extraction methods that safeguard RNA integrity is therefore critical.
Quality Assessment of RNA
Quality assessment of RNA is perhaps the final gatekeeper in cDNA synthesis. This process ensures that the RNA used for reverse transcription is of high quality, which directly influences the fidelity of cDNA produced. Methods like spectrophotometry and gel electrophoresis are commonly employed to gauge the integrity of the RNA.
One key aspect of quality assessment is the use of specific RNA quality metrics, such as the RNA Integrity Number (RIN). This metric provides a quantifiable measure of RNA quality, allowing researchers to make informed decisions regarding the usage of RNA in cDNA synthesis. Notably, ensuring high-quality RNA not only enhances the efficiency but also minimizes the risk of erroneous cDNA synthesis, making it an indispensable part of the cDNA construction workflow.
Effective methodologies for constructing cDNA are essential for advancing genomic research and applications in functional genomics.
Effective methodologies for constructing cDNA are essential for advancing genomic research and applications in functional genomics.
In summary, each element of cDNA construction—from the reverse transcription processes to the meticulous assessment of RNA quality—plays a decisive role in the fidelity and reliability of the resultant cDNA. These methodologies not only enhance the validity of research findings but also set the stage for groundbreaking discoveries in molecular biology.
Amplification of cDNA
Amplifying complementary DNA (cDNA) is an essential aspect of molecular biology that significantly impacts various fields, including genetics, microbiology, and biomedicine. At its core, cDNA amplification serves to increase the quantity of cDNA, enabling in-depth analysis of gene expression and facilitating experimentation. As cDNA is typically synthesized in small amounts, amplification methods ensure that researchers can obtain sufficient quantities for downstream applications such as sequencing, cloning, and qPCR.
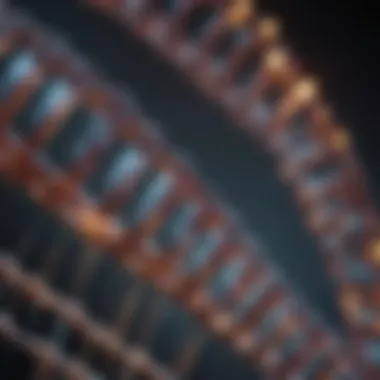
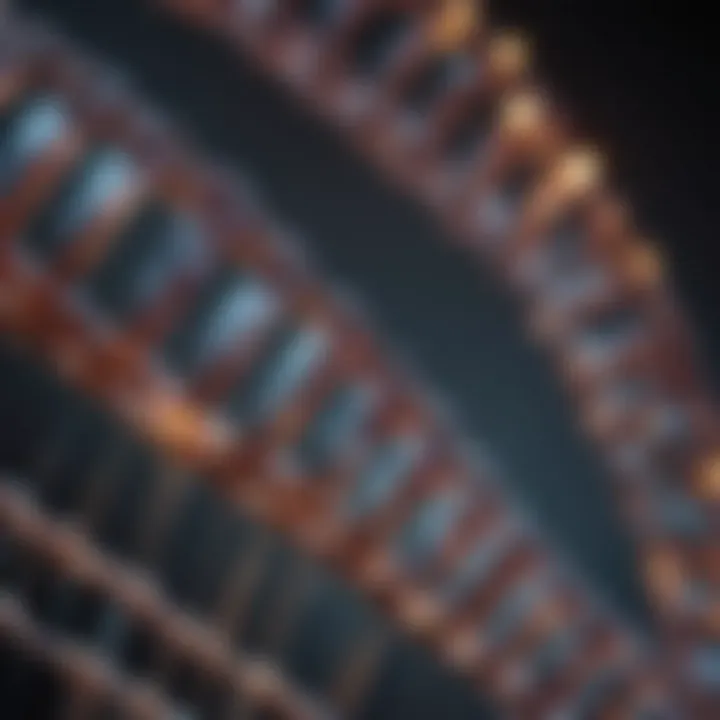
One of the primary techniques utilized for cDNA amplification is the polymerase chain reaction, or PCR, which allows for the exponential replication of specific DNA fragments. Understanding the methods and technologies associated with this process is vital for researchers who wish to explore gene expression patterns and develop applications that could lead to significant scientific advancements.
Polymerase Chain Reaction (PCR) Applications
Conventional PCR
Conventional PCR has been a cornerstone in molecular biology since its introduction. This technique allows for the amplification of targeted cDNA sequences through repetitive cycles of denaturation, annealing, and elongation. The process is relatively straightforward and economical, making it a popular choice among researchers.
One of the key characteristics of conventional PCR is its ability to generate millions of copies of a specific DNA segment within a few hours. This feature is particularly advantageous when working with limited starting materials, such as RNA derived from small tissue samples.
However, while conventional PCR is effective, it does have its limitations. It relies on gel electrophoresis for analyzing amplified products, which can be time-consuming and requires additional reagents. Despite this, it remains compelling for initial screening of cDNA constructs and verifying the success of cDNA synthesis.
Real-Time PCR Techniques
Real-time PCR, often referred to as quantitative PCR (qPCR), represents an evolution of conventional methods by allowing for the real-time monitoring of DNA amplification. This technique utilizes fluorescent dyes or probes to quantify the cDNA as it is synthesized, considerably enhancing the sensitivity and precision of measurements.
The key characteristic that sets real-time PCR apart is its ability to provide quantitative data on gene expression. This means researchers can not only confirm the presence of cDNA but also assess its relative abundance in different samples. This feature is especially useful in studies comparing gene expression across various conditions or treatments.
Nonetheless, real-time PCR requires specialized equipment and may involve higher costs than conventional PCR. The accuracy of the results is highly dependent on the careful design of primers and probes, as any missteps might lead to unreliable data.
Hybridization Techniques for cDNA
Hybridization techniques play an essential role in studying cDNA by providing methods to detect and quantify specific sequences. These methods utilize complementary nucleic acid strands to form stable hybrids, allowing researchers to pinpoint gene expression with precision. Arrays, probes, and PCR products are typical tools in the hybridization toolbox that facilitate these explorations.
By combining amplification methods with hybridization techniques, scientists can dramatically enhance their understanding of genetic regulation and expression. This dual approach allows for a comprehensive analysis of cDNA, making it a vital tool in molecular biology.
Quality Control of cDNA
Quality control in the construction of complementary DNA (cDNA) plays a crucial role in ensuring that the synthesized material is both reliable and suitable for subsequent applications in molecular biology. Given that cDNA serves as a fundamental building block in gene expression studies, functional genomics, and various other research fields, maintaining high standards of quality is paramount. Without proper quality control measures, the validity of experimental results can be thrown into disarray, leading to incorrect conclusions that may affect further research or clinical decisions.
Assessing cDNA Quality and Yield
When it comes to assessing the quality of cDNA, scientists often utilize several key metrics. These assessments typically involve evaluating both the integrity and quantity of the cDNA produced. Here are some methods that researchers commonly employ:
- Agarose Gel Electrophoresis: This technique allows for the visualization of cDNA fragments. By staining the gel and comparing the bands against a marker, it’s possible to assess the size distribution and integrity of the cDNA.
- Spectrophotometry: Using tools like a NanoDrop, researchers measure the absorbance at specific wavelengths to estimate the concentration of the cDNA. This can also give insights into the purity of the sample by assessing the ratios of absorbance at 260 nm and 280 nm.
- qPCR: Real-time quantitative polymerase chain reaction (qPCR) not only amplifies cDNA but also quantifies it, providing additional data on yield and purity. The efficiency of amplification can be crucial to ensure the cDNA is competent for downstream applications.
In all these methods, it’s vital to establish standardized protocols to ensure reproducibility. Higher quality not only improves the reliability of results but also saves time and resources by reducing the need for repetitive experiments due to failed assays.
Troubleshooting Common cDNA Synthesis Issues
Even with diligent quality control, researchers may face challenges during cDNA synthesis. Common issues can stem from various processes, such as reverse transcription, purification, or amplification. Here’s how to approach some of these common pitfalls:
- Low Yield: A frequent problem is obtaining low cDNA yields, which can arise from subpar RNA templates or inefficient reverse transcription. Ensuring that the RNA is intact and free from contaminants can help alleviate this problem. The use of high-quality reverse transcriptases can also greatly improve yields.
- Presence of Contaminants: Residual contaminants can inhibit enzymatic reactions. It’s crucial to ensure that RNA extraction methods are optimized to remove all potential inhibitors, such as proteins or phenol.
- Ineffective Primer Design: Inefficient primers can lead to poor amplification outcomes. Checking the specificity and performance of primers through iterative testing can identify potential failures before undertaking larger experiments.
- Poor Quality RNA: Sometimes the RNA starting material might not be of adequate quality, which will directly influence cDNA synthesis. Regular assessment of RNA integrity using capillary electrophoresis can safeguard against this issue.
Ultimately, having robust quality control processes in place is not just a box to tick—it’s an insurmountable part of ensuring integrity in genetic research. Prioritizing cDNA quality safeguards the pathway from hypothesis to discovery, illuminating the shadows of uncertainty that often accompany molecular biology.
Applications of cDNA in Research
The applications of complementary DNA (cDNA) in research represent a cornerstone in molecular biology, enabling scientists to dissect the complexities of gene expression and functional genomics. Understanding how genes are expressed in various conditions—healthy or diseased—offers tremendous insights necessary for advancing biological knowledge and therapeutic development.
cDNA acts as a tangible bridge between the raw genetic code and its practical implications. By synthesizing cDNA from the messenger RNA (mRNA) of interest, researchers can create tools that facilitate various forms of experimentation, leading to discoveries that range from basic science to applied clinical breakthroughs. The following sections detail two primary and noteworthy applications of cDNA in research: the construction and use of cDNA libraries, and the role of cDNA in RNA sequencing studies.
cDNA Libraries: Construction and Use
Creating cDNA libraries is like building a repository of genetic information; it allows for the storage and retrieval of specific sequences of interest. This process begins with the extraction of mRNA from a chosen tissue or cell type, which will then serve as the template for cDNA synthesis. It enables researchers to assess which genes are active at various stages or under diverse conditions.
Key Steps in cDNA Library Construction:
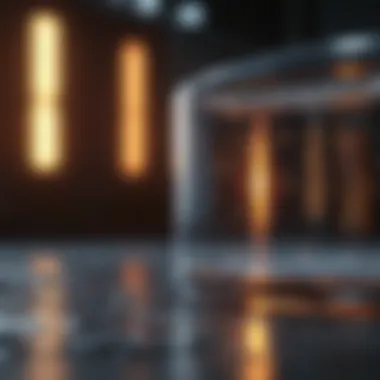
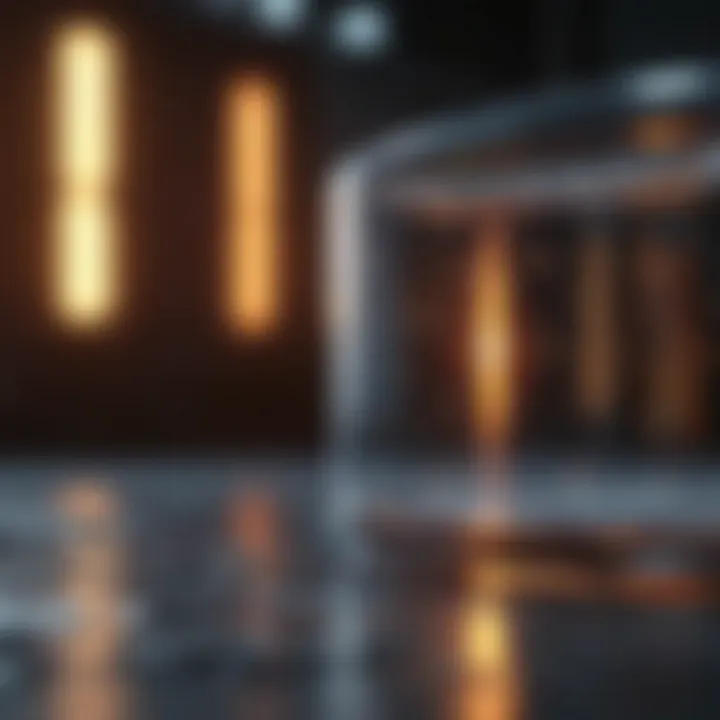
- mRNA Isolation: The initial step is isolating high-quality mRNA from the biological sample. This step is critical as the purity and integrity of mRNA directly impact the success of downstream applications.
- Reverse Transcription: The next step involves reverse transcription, where the isolated mRNA is converted into cDNA using reverse transcriptase enzymes. This enzyme plays a pivotal role by synthesizing complementary DNA strands from the RNA template.
- Amplification and Cloning: Once the cDNA is synthesized, it can be amplified via polymerase chain reaction (PCR). The amplified cDNA can then be cloned into vectors to create cDNA libraries. Each clone carries a unique cDNA insert that represents a specific mRNA molecule from the original sample.
cDNA libraries are instrumental for many applications including gene expression analysis, functional analysis of genes, and identifying novel genes. They serve as invaluable resources in discovering the roles of various genes within different cellular contexts. The versatility of cDNA libraries makes them vital for researchers hoping to dive deeper into the transcriptions that dictate cellular behavior.
cDNA in RNA Sequencing Studies
Another significant application of cDNA is in RNA sequencing (RNA-seq). This high-throughput technique allows for the comprehensive assessment of the transcriptome, which consists of all RNA molecules, including mRNA, present in a biological sample. cDNA is crucial in RNA-seq because it converts RNA into a stable format that's easier to sequence.
Steps Involved in Using cDNA for RNA Sequencing:
- Preparation of cDNA: Following the isolation of RNA, researchers convert it into cDNA. This includes fragmentation of RNA, reverse transcription, and library preparation that’s ready for sequencing.
- Sequencing: The constructed cDNA libraries undergo sequencing, typically through next-generation sequencing (NGS) technologies. This process generates vast amounts of data on gene expression levels, splicing variations, and even novel transcriptional units.
- Data Analysis: Post-sequencing, data is aligned and analyzed to derive meaningful insights about the transcriptome under investigation. These can reveal how gene expression varies in different tissues or conditions, serving as a potent tool for understanding disease mechanisms and potential therapeutic strategies.
By leveraging cDNA in RNA sequencing, researchers can unravel the complexities of gene expression, leading to insights that have far-reaching implications in health, disease, and beyond. In summary, the applications of cDNA are not just a mere technicality but a powerful vehicle driving forward our understanding of the intricate world of molecular biology.
Future Directions and Innovations in cDNA Research
The landscape of molecular biology is evolving at a pace that is nothing short of breathtaking. At the core of this evolution lies the construction and application of complementary DNA (cDNA). Understanding future directions in cDNA research is vital for both seasoned researchers and newcomers alike. The innovations in this field can lead to breakthroughs that span diverse scientific realms, offering insights not just into gene expression but also into the intricacies of genetic manipulation and therapeutic developments.
Emerging Technologies in cDNA Synthesis
Recent advancements in cDNA synthesis have opened up new avenues for efficiency and specificity. New methods promise to streamline the synthesis process, reducing time without sacrificing quality. One such advancement is the use of Nanopore technology, which allows for real-time sequencing of cDNA, showing how molecules behave under various conditions. This can lead to a more nuanced understanding of gene regulation.
Another groundbreaking technology is CRISPR-associated RNA (Cas RNA), which leverages the precision of CRISPR gene-editing tools for cDNA synthesis. With this technique, researchers can selectively amplify specific RNA segments, thereby creating highly targeted cDNA constructs.
Some trends to keep an eye on include:
- Automation of cDNA synthesis: Utilizing robotics to minimize human error and standardize protocols.
- High-throughput sequencing: Facilitating the simultaneous synthesis of multiple cDNA samples for larger population studies.
- Single-cell cDNA synthesis: Enabling an examination of gene expression in individual cells, rather than averaged responses across populations, which can mask critical variations.
These technologies are reshaping how scientists approach cDNA synthesis. The ability to synthesize cDNA quickly while retaining accuracy opens doors to complex studies in disease models, functional genomics, and more.
Potential Advances in Clinical Applications
As we look ahead, the clinical implications of cDNA research shine like a beacon. The potential advances are not just theoretical; they resonate with real-world applications that could revolutionize healthcare practices. For instance, the integration of cDNA synthesis in personalized medicine holds significant promise. By analyzing cDNA derived from a patient’s individual RNA, clinicians can tailor treatments based on their unique gene expression profiles.
Moreover, advancements in gene therapy are heavily reliant on cDNA. The capacity to produce customized cDNA allows for targeted interventions in genetic disorders, potentially correcting dysfunctional gene activity.
Important Considerations
While the future looks bright, it's imperative to tread carefully. The ethical implications of cDNA manipulation cannot be overlooked. As researchers push the boundaries, they must grapple with questions about genetic privacy, consent, and the societal impacts of genetic alterations. Regulatory frameworks are still catching up to the speed of advances, and navigating this landscape will require vigilance from the scientific community.
In summary, the future of cDNA research is full of promise and complexity. The innovations in synthesis technologies can enhance our understanding of genetic mechanisms, while the potential clinical applications pave the way for significant improvements in healthcare. As researchers continue to explore these directions, the importance of ethical considerations will also stand at the forefront, ensuring that the benefits of this research can be realized responsibly.
Ethical Considerations in cDNA Research
The topic of ethical considerations in the construction of cDNA is paramount in this evolving field of molecular biology. As researchers delve deeper into gene manipulation and expression studies, it becomes essential to navigate the intricate moral landscapes associated with genetic material. This section examines the major ethical elements involved, the benefits of stringent ethical protocols, and the considerations that accompany cDNA research.
Ethics of Genetic Material Manipulation
Genetic material manipulation, particularly through the synthesis of cDNA, brings forth a host of ethical dilemmas. Primarily, there’s the question of consent when sourcing genetic material. Human-derived RNA and subsequent cDNA must be obtained ethically and with informed consent from donors. The implications of using such materials can be far-reaching, and any oversight in this area can lead to significant ethical repercussions.
Moreover, the manipulation of genetic material could raise concerns around equity and accessibility. Those who conduct research must consider who benefits from the advancements in cDNA technology. Are these benefits exclusively reserved for a select few, or are they accessible to a broader community? Efforts should be made to ensure equitable access to the applications of cDNA research, such as in developing therapies or diagnostics, ultimately promoting fairness in scientific progression.
Additionally, the potential for misuse of such technologies cannot be overlooked. The ability to edit genes or create cDNA poses risks for nefarious applications, such as bioweapons or unethical enhancements. Researchers are called to act responsibly, weighing the societal impacts of their work alongside their scientific endeavors.
Navigating Regulatory Frameworks
Regulatory frameworks surrounding cDNA research are pivotal for ensuring ethical adherence and safeguarding against potential misuse. These frameworks guide researchers through the maze of legal considerations while providing a structured approach to ethically sound research practices.
Governments and organizations often implement guidelines that stipulate the parameters of research involving genetic material. For instance, the National Institutes of Health (NIH) in the United States and similar bodies worldwide have established regulations to oversee genetic research. Their roles include:
- Review and Approval: New projects typically undergo rigorous review processes where ethical implications are assessed. This step ensures that all research adheres to established ethical standards.
- Monitoring: Continuous oversight of ongoing research helps detect any deviations from regulatory guidelines, enabling proactive measures.
- Public Engagement: Sharing information and fostering discussions around genetic research helps build trust within the broader community, allowing the public to voice concerns and expectations.
In addition to national guidelines, international bodies like the World Health Organization (WHO) offer ethical directives that shape cDNA research on a global scale. Adhering to these frameworks not only protects the integrity of the research but also enhances the credibility of the findings.