Enhancing Resolution in Scientific Imaging Techniques
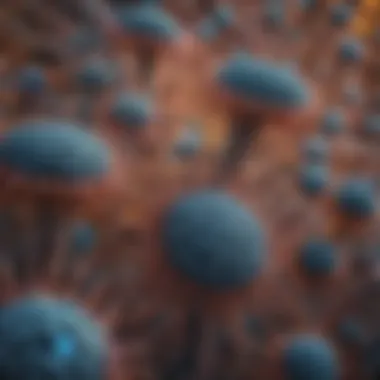
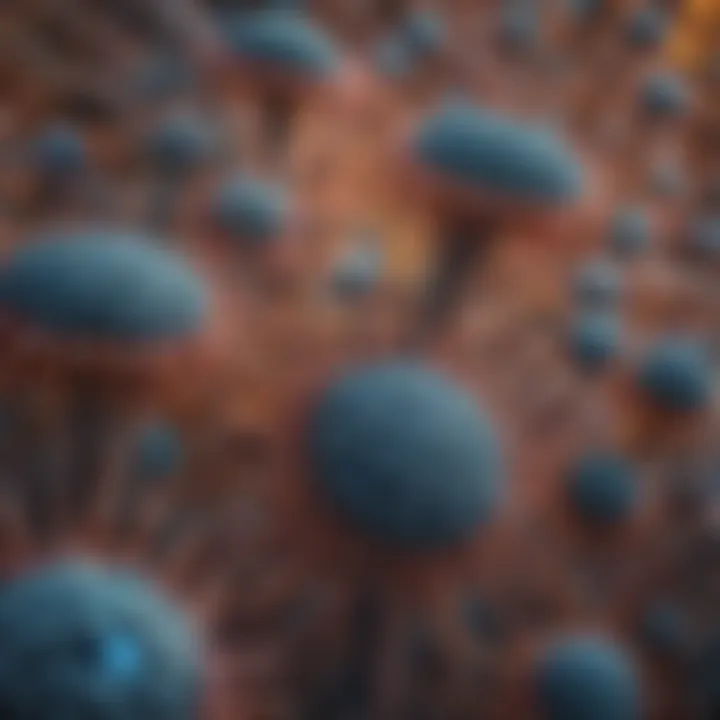
Intro
Enhancing resolution in scientific imaging is a critical area that has significant implications across various fields, including biology, chemistry, and materials science. High-resolution imaging allows researchers to discern fine details that may otherwise be obscured, leading to improved data accuracy and deeper insights into complex phenomena. This article will explore various methodologies and technologies that contribute to resolution enhancements, highlighting the importance of these advancements in scientific discovery.
Key Concepts
Definition of the Main Idea
Resolution in scientific imaging refers to the ability to distinguish between two closely spaced objects. This concept is fundamental to effectively capturing and analyzing images. Higher resolution leads to clearer images, enabling more precise measurements and analyses. In scientific practices, resolution is often quantified by parameters such as spatial resolution and spectral resolution, each serving distinct purposes across different imaging contexts.
Overview of Scientific Principles
Scientific imaging technologies operate on principles derived from physics and optics. Techniques like microscopy rely on specialized lenses and light manipulation to magnify images, while spectroscopy utilizes light properties to characterize materials. Understanding these principles aids in comprehending how different methodologies enhance resolution. For instance, optical microscopes, electron microscopes, and advanced imaging techniques such as super-resolution microscopy, significantly vary in resolution capabilities depending on their underlying principles.
Current Research Trends
Recent Studies and Findings
Recent studies have shown remarkable progress in enhancing resolution through both optical and computational methods. For example, innovations in super-resolution fluorescence microscopy have enabled scientists to visualize biomolecular complexes at nanometer-scale precision. Research published in Nature Methods demonstrates the effectiveness of techniques like STORM and PALM in overcoming the diffraction limit of light. Such advancements allow for detailed investigations into cellular processes.
Significant Breakthroughs in the Field
The integration of artificial intelligence into imaging analysis represents a significant breakthrough. Machine learning algorithms have been developed to improve image clarity and extract relevant patterns. Additionally, techniques like cryo-electron microscopy have emerged, allowing researchers to observe biological structures in their native states, thereby providing more accurate data. These breakthroughs highlight a growing synergy between traditional imaging techniques and modern computational advancements.
"Investment in resolution enhancement technologies is not just beneficial; it is essential for the future of scientific inquiry."
"Investment in resolution enhancement technologies is not just beneficial; it is essential for the future of scientific inquiry."
Prologue to Resolution in Scientific Contexts
Resolution is a fundamental concept in scientific imaging and analysis. It refers to the ability to distinguish between two closely spaced objects or events in an image. Understanding resolution is crucial for researchers and practitioners, as it directly impacts the quality of data they gather. In various scientific fields like biology, chemistry, and materials science, high resolution enables more accurate interpretations of images and measurements, thus facilitating deeper insights into structures and processes.
Defining Resolution
In scientific imaging, resolution can be defined as the smallest distance between two points that can be distinctly identified. It encompasses several dimensions, including spatial resolution, temporal resolution, and spectral resolution, each essential to specific types of analysis. Spatial resolution relates to the ability to resolve detail in a physical space. Temporal resolution addresses how quickly one can capture changes over time, while spectral resolution refers to the ability to resolve different wavelengths in light.
The measurement of resolution often depends on the instruments used. For example, in optical microscopy, resolution is limited by the wavelength of light. Conversely, electron microscopy can achieve significantly higher resolutions due to shorter wavelengths associated with electrons. This difference illustrates why defining resolution in a scientific context includes the specific methodology applied.
Importance of Resolution in Science
The significance of resolution in science cannot be overstated. High-resolution imaging allows scientists to visualize and analyze structures at a molecular or atomic level. This capability leads to breakthroughs in understanding biological processes, material characteristics, and chemical reactions. Resolution improvements can grant access to previously unresolvable features, giving rise to new hypotheses and theories.
Increase in resolution is also linked to enhanced data accuracy. In fields like medical imaging, for instance, higher resolution can lead to more precise diagnoses and better patient outcomes. It is essential for detecting diseases earlier and developing targeted therapies.
Moreover, resolution plays a key role in the reproducibility and reliability of scientific results. Higher resolution means clearer and more detailed information, critical for corroborating findings in peer-reviewed research. In result, increased resolution is not just a technical advance; it represents a paradigm shift in how scientists approach investigation and interpretation of data.
“Resolution improvements bridge gaps in scientific understanding, facilitating discoveries that redefine existing knowledge.”
“Resolution improvements bridge gaps in scientific understanding, facilitating discoveries that redefine existing knowledge.”
Types of Resolution in Scientific Imaging
Understanding the various types of resolution is crucial in scientific imaging because they define how much detail can be captured in an image. Each type of resolution—spatial, temporal, and spectral—serves distinct purposes, affecting the interpretation and application of the data collected. This section explores these types and their significance in enhancing our understanding of scientific phenomena.
Spatial Resolution
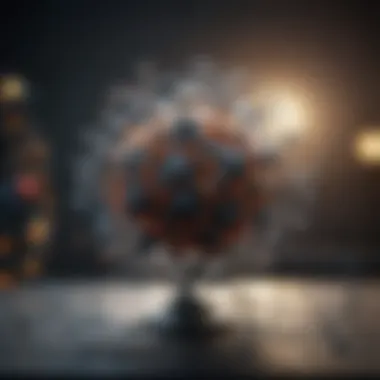
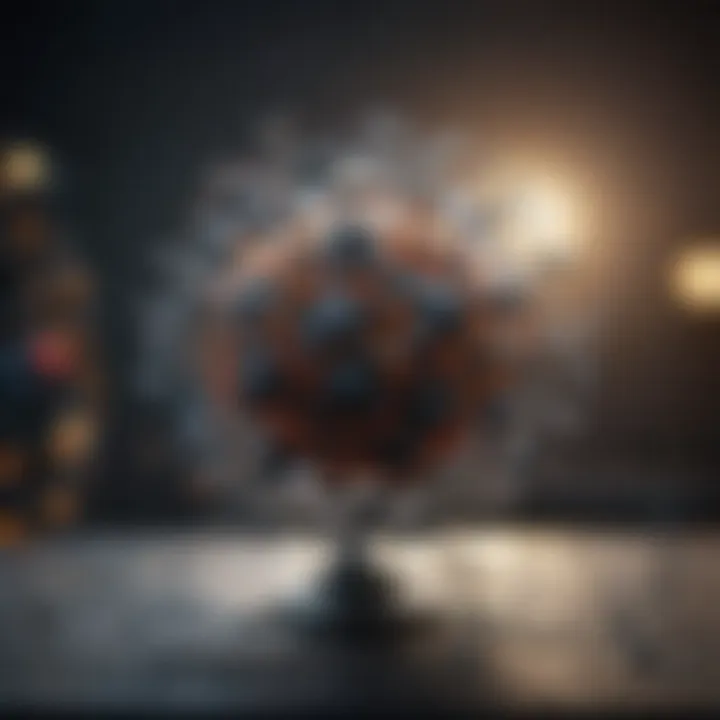
Spatial resolution refers to the smallest discernible detail in an image. It is fundamentally linked to the concept of how fine the imaging system can resolve its subjects. High spatial resolution provides clearer images and allows for the identification of small features that may be crucial for analysis.
In imaging systems such as optical microscopes, spatial resolution is often limited by diffraction, which describes the spreading of light waves. However, advancements like super-resolution microscopy techniques can bypass these limitations. These methods enable scientists to visualize structures at the nanometer scale, offering insights that were previously unattainable. Furthermore, higher spatial resolution is essential in fields such as
- Biological Studies: Understanding cellular structures and their functions.
- Materials Science: Observing defects in materials or the arrangement of atoms.
Temporal Resolution
Temporal resolution indicates how frequently an imaging system can capture data over time. High temporal resolution is critical when analyzing dynamic processes. For example, in biological systems, observing cellular activities in real-time—like cell division or movement—depends on capturing images at rapid intervals.
In methods like video microscopy, the frame rate can significantly affect how phenomena are perceived. High frame rates can help in recording brief events with more accuracy, allowing researchers to gain a comprehensive view of the dynamics involved. Fields such as fluid dynamics also benefit from high temporal resolution by capturing the transient behaviors of substances in motion.
Spectral Resolution
Spectral resolution involves the ability to differentiate between various wavelengths of light. This type of resolution is particularly vital in techniques such as spectroscopy, where understanding the composition of materials based on their spectral fingerprints is essential.
A higher spectral resolution enables finer distinctions between closely spaced spectral lines, crucial in applications such as:
- Chemical Analysis: Identifying compounds based on characteristic absorption or emission spectra.
- Environmental Monitoring: Discerning different substances in the atmosphere or body fluids.
In summary, spatial, temporal, and spectral resolutions each bring unique strengths to scientific imaging, enabling more detailed and accurate analyses across various fields. This foundation allows for groundbreaking advancements and discoveries that rely heavily on the quality of resolution achieved.
Imaging Techniques and Their Resolution Limitations
Understanding the limitations of different imaging techniques is critical in the pursuit of higher resolution in scientific imaging. Each method used to capture images of materials or biological specimens has unique strengths and weaknesses. These limitations can directly impact the quality and accuracy of scientific data obtained from imaging. In many cases, advancements in resolution are contingent upon overcoming these challenges to achieve a more detailed and informative picture of the subject being studied. While seeking to enhance resolution, it is equally vital to comprehend how these techniques function and where their boundaries lie.
Optical Microscopy
Optical microscopy is one of the foundational techniques in scientific imaging. It employs visible light to magnify and visualize specimens, allowing researchers to observe cellular structures, microorganisms, and other materials at different magnifications. The resolution of optical microscopes is typically limited by the wavelength of light used. Generally, the achievable resolution is around 200 nanometers, primarily due to diffraction limitations.
The simplicity and accessibility of optical microscopy make it a popular choice, but its limitations necessitate the use of advanced techniques for improved resolution. Techniques like phase contrast, fluorescence, and confocal microscopy enhance image quality and depth by utilizing specialized optics and fluorescent dyes. However, these methods still grapple with inherent resolution barriers that stem from the properties of light itself.
Electron Microscopy
Electron microscopy represents a significant leap forward in imaging resolution. Utilizing a beam of electrons, it can achieve resolutions much greater than optical microscopy. The resolution can reach as fine as 1 nanometer due to the much shorter wavelength of electrons compared to visible light. This form of microscopy is invaluable for examining materials at the nanoscale, providing insights into atomic structures and surface features.
Despite its high resolution, electron microscopy comes with its own set of limitations. The requirement for a vacuum environment can restrict the types of samples that can be examined. Moreover, the preparation process often leads to artifacts that can alter the true representation of the specimen. Therefore, while electron microscopy offers immense detail, careful sample preparation and interpretation are crucial to ensure accurate results.
Scanning Probe Techniques
Scanning probe techniques, such as atomic force microscopy (AFM) and scanning tunneling microscopy (STM), operate by feeling the surface of a sample with a probe tip. These techniques achieve exceptionally high resolutions, often at the atomic level. AFM, for instance, can produce images with resolutions of just a few nanometers, making it suitable for biological and material sciences.
However, the operational principles bring forth challenges. For example, scanning probe microscopy can be significantly slower than other techniques, making it less practical for imaging larger areas. Additionally, surface conditions can considerably influence the quality of the obtained images. Researchers must carefully consider sample conditions and probe interactions to prevent spatial artifacts or distortions in high-resolution images.
"Understanding the limitations associated with various imaging techniques is crucial for selecting the appropriate method for a specific application."
"Understanding the limitations associated with various imaging techniques is crucial for selecting the appropriate method for a specific application."
In summary, while optical, electron, and scanning probe techniques each offer unique benefits and advancements in resolution, they also present notable limitations. The choice of an imaging technique should consider these limitations alongside the desired outcome. By exploring these factors, researchers can better navigate the landscape of scientific imaging to enhance resolution effectively.
Advancements in Resolution Enhancement Techniques
In the ever-evolving field of scientific imaging, advancements in resolution enhancement techniques are critical. These methodologies not only allow scientists to observe finer details but also enable new discoveries across various fields. Improved image quality aids in better data interpretation, which is essential for both research and application. Here, we will explore three notable techniques that have made significant contributions to enhancing resolution.
Super-Resolution Microscopy
Super-resolution microscopy has fundamentally changed our ability to visualize finer structures in biological specimens. Traditional optical microscopy is limited by the diffraction of light, making it difficult to observe features smaller than 200 nanometers. Super-resolution techniques, such as STORM (Stochastic Optical Reconstruction Microscopy) and PALM (Photo-Activated Localization Microscopy), break this barrier using various strategies.
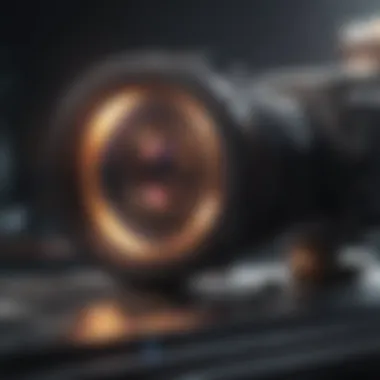
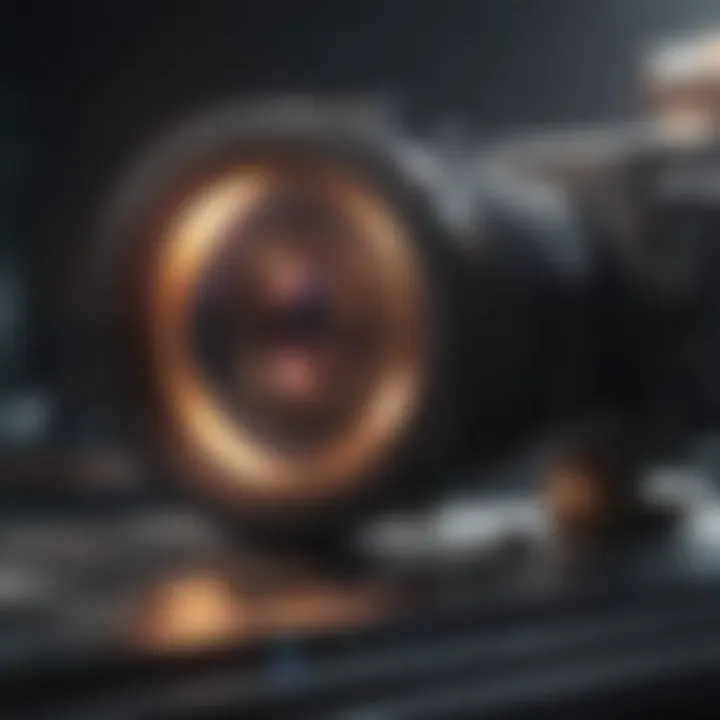
These methods utilize fluorescence to pinpoint molecular locations with precision. By capturing multiple images and processing the data algorithmically, researchers can reconstruct images with resolutions down to tens of nanometers. This opens new avenues in biological research, such as visualizing protein interactions in live cells. The overriding benefit here is clarity, which allows researchers to track dynamic processes in real-time.
Computational Imaging Approaches
Computational imaging blends the hardware of imaging systems with advanced algorithms to enhance image resolution. Techniques like phase retrieval and iterative reconstruction transform raw data into high-resolution images. These approaches rely on mathematical models that can extrapolate details not directly captured by the sensors.
For instance, tomographic imaging in materials science often includes incomplete data sets due to physical constraints. Computational imaging techniques can fill in gaps by leveraging information from various angles. Furthermore, these approaches are not limited to just static images; they can also be applied in time-lapse imaging, providing insights into dynamic phenomena. This integration of computation into imaging processes emphasizes the importance of algorithm development alongside hardware advancement.
Machine Learning in Imaging
The application of machine learning in imaging is rapidly rising. Through training on large datasets, machine learning algorithms can identify patterns and enhance image quality. Techniques such as convolutional neural networks (CNNs) are used to improve signal-to-noise ratios, essentially making clearer images from noisy data.
Researchers are employing machine learning for anomaly detection in industrial applications and medical diagnostics. By automating the interpretation of high-resolution images, these algorithms reduce human error and speed up analysis, making them invaluable in several sectors. As machine learning continues to advance, its role in improving resolution will become more pivotal.
Key Takeaway: The integration of super-resolution microscopy, computational imaging, and machine learning signifies a transformative era in scientific imaging, where clarity and detail enable deeper understanding and discovery across diverse scientific disciplines.
Key Takeaway: The integration of super-resolution microscopy, computational imaging, and machine learning signifies a transformative era in scientific imaging, where clarity and detail enable deeper understanding and discovery across diverse scientific disciplines.
Applications of Enhanced Resolution in Key Scientific Fields
Enhanced resolution in scientific imaging is not merely a technical advancement; it fundamentally transforms research and applications across various fields. The importance of improving resolution lies in its capability to reveal details that were previously hidden, yielding insights that can lead to groundbreaking discoveries. When resolution is increased, the precision of observations also improves, enhancing both the quality and reliability of data produced in scientific investigations.
Moreover, enhanced resolution plays a critical role in bridging theoretical concepts with practical implications. It allows researchers to visualize phenomena at a microscopic level, thus facilitating the understanding of complex interactions within different systems. This article examines three key scientific fields where resolution enhancement is imperative: biological research, materials science, and environmental science. Each area demonstrates unique applications and distinct benefits derived from improved imaging techniques.
Biological Research
In biological research, enhanced resolution is essential for studying living organisms at a cellular and molecular level. Advanced imaging techniques, such as super-resolution microscopy, allow scientists to observe cellular structures in unprecedented detail. For example, the ability to discern individual proteins within a cell opens avenues for understanding cellular functions and mechanisms of diseases.
The applications include:
- Cellular Imaging: Identifying cellular processes, such as protein interactions and cellular signaling, which contribute to overall cell behavior.
- Disease Diagnosis: Early detection of abnormalities like cancer through high-resolution imaging of tissues can lead to timely interventions.
- Drug Development: Enhanced imaging helps in tracking how drugs interact at cellular levels, contributing to more effective therapeutic strategies.
In summary, enhanced resolution in biological research not only enriches academic knowledge but also paves the way for practical medical advancements.
Materials Science
Materials science benefits profoundly from enhanced imaging resolution. It allows for the characterization of materials at nanoscales, leading to innovations in technology and engineering. High-resolution imaging contributes to the understanding of material properties, allowing scientists to design materials with specific functionalities.
Applications in materials science encompass:
- Nanotechnology: Observation of nanoscale materials provides insights into their unique properties, enabling tailored applications in electronics and medicine.
- Structural Analysis: Understanding the internal structure of materials can predict how they respond under different conditions, thus informing better material design.
- Failure Analysis: High-resolution imaging helps investigate failure mechanisms in materials, leading to improvements in safety and reliability.
Thus, the implications of enhanced resolution extend beyond science and foster advancements in various industries where materials are fundamental.
Environmental Science
In environmental science, increased resolution is key to understanding complex ecosystems and environmental changes. Enhanced imaging techniques assist researchers in monitoring environmental parameters with greater accuracy, facilitating the analysis of intricate relationships within ecosystems.
Examples of applications include:
- Ecosystem Monitoring: High-resolution satellite imaging can track changes in land use, deforestation, and urbanization with precision, aiding effective policy-making.
- Pollutant Tracking: Enhanced resolution allows for the detection and study of pollutants, providing crucial data for environmental protection strategies.
- Biodiversity Studies: Detailed imaging aids in species identification and monitoring, critical for conservation efforts.
The integration of enhanced resolution in environmental science underscores the importance of precise data in addressing ecological issues and contributing to sustainability efforts.
Limitations and Challenges in Increasing Resolution
Understanding the limitations and challenges in increasing resolution is crucial for researchers and educators alike. Enhancements in scientific imaging have allowed us to observe and analyze phenomena at unprecedented scales. However, with these advancements come inherent hurdles that can impede potential breakthroughs. This section will cover several physical limitations, data processing challenges, and issues related to interpreting high-resolution data.
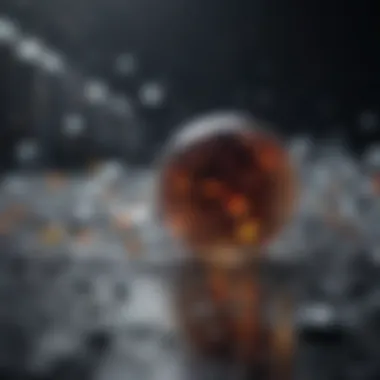
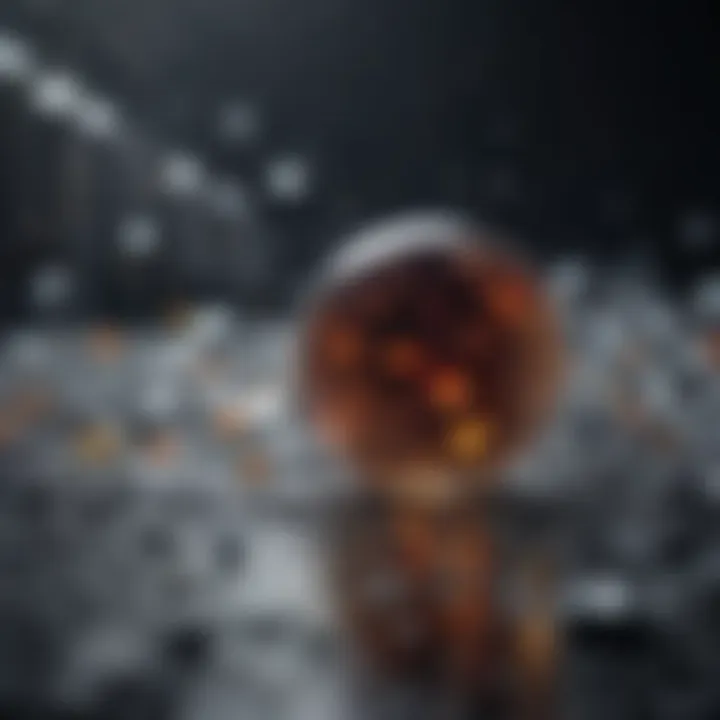
Physical Limitations
Physical limitations play a significant role in determining the maximum achievable resolution. These constraints are often dictated by the fundamental principles of physics, and they differ across various imaging techniques. For instance, in optical microscopy, diffraction limits become a crucial consideration. The classical theory outlines that light waves can only resolve details that are a fraction of the wavelength of light. This is particularly pertinent in biological sciences, where cellular structures are often below this limit.
"Resolution is not merely a technical specification; it is bound by principles that can confound even the best methods."
"Resolution is not merely a technical specification; it is bound by principles that can confound even the best methods."
Additionally, imaging modalities like electron microscopy face their own set of physical challenges. The electron beam's interaction with the sample can lead to various issues, including sample damage and noise, which can obscure fine details. As researchers seek higher resolution techniques, innovative solutions must address these physical constraints while preserving sample integrity.
Data Processing Challenges
As imaging resolution increases, so does the volume of data generated. Higher resolution images contain significantly more pixels, resulting in larger file sizes and more complex datasets. This explosion of data presents formidable challenges for processing and analysis. Researchers frequently find themselves limited by the available computational power and processing algorithms that can handle such extensive datasets without losing vital information.
Data storage also becomes a significant concern. High-resolution images require sophisticated storage solutions to ensure quick access and retrieval. The technology must evolve to manage vast amounts of information while maintaining data integrity, often necessitating collaboration between imaging scientists and data engineers.
Interpretation of High-Resolution Data
Interpreting high-resolution data is not a straightforward task. Enhanced detail does not always translate to a clearer understanding. Researchers must be particularly cautious about noise, artifacts, and potential misinterpretation of data. High resolution might lead to over-interpretation or the identification of features that may not have biological significance.
Furthermore, experts must develop appropriate frameworks for analysis to leverage the information provided by high-resolution images. This requires a combination of domain knowledge and technical expertise, as missteps in interpretation can lead to erroneous conclusions. As a result, continuous training and education become necessary components for professionals who aim to work with advanced imaging technologies effectively.
Future Prospects in Resolution Improvement
The future of resolution improvement in scientific imaging is critical as advancements in resolution can lead to significant breakthroughs in various scientific domains. Enhanced resolution allows for more detailed observations, which can drive discoveries, refine methodologies, and develop innovative technologies. As fields like biology, chemistry, and materials science evolve, the quest for better resolution methods remains paramount.
Emerging Technologies
A range of emerging technologies promises to enhance resolution in scientific imaging. One notable area is the enhancement of optical and electron microscopes. Techniques such as fourier ptychographic microscopy increase resolution by computationally merging multiple low-resolution images into one high-resolution image. Another exciting technology is quantum dot imaging, which utilizes semiconductor nanocrystals for improved imaging contrast and resolution.
These technologies are not just theoretical; they are already being integrated into various fields, including biomedical research and nanotechnology. Researchers are enthusiastic about how these cutting-edge methods can refine imaging techniques further. Improved resolution can deliver insights at cellular and molecular levels that were previously beyond accessible limits.
Cross-disciplinary Approaches
The advancement of resolution improvement is increasingly relying on a cross-disciplinary approach. Collaboration among diverse fields, such as computer science, biology, and engineering, is essential. Techniques developed in one area can often translate to another; for instance, algorithms from artificial intelligence applications are proving useful in improving imaging resolution. For example, the combination of machine learning with imaging has shown great promise in enhancing resolution and reducing noise in signals.
Moreover, researchers from different domains share insights that lead to innovative solutions. For example, materials scientists can inform biologists on how to manipulate imaging techniques for specific applications. This exchange is crucial for developing holistic applications that improve resolution across varied scientific contexts.
The Role of Community in Advancements
Community plays a fundamental role in pushing the boundaries of resolution enhancement in scientific imaging. Collaborative efforts among researchers, institutions, and educational bodies facilitate knowledge sharing and resource exchange. Online platforms like Reddit or social media can serve as forums for discussions, allowing scientists to connect, collaborate, and seek advice on specific imaging challenges.
Additionally, open-source communities contribute significantly by making advanced imaging software accessible to smaller labs that might lack resources. This democratization of technology helps drive research innovation at many levels. The cumulative knowledge and effort from the community result in faster advancements and problem-solving capabilities, thus ensuring that improvements in resolution are widely understood and applied.
The End
In the context of scientific imaging, identifying the significance of resolution is paramount. The advancements discussed throughout this article illustrate how enhanced resolution can unlock new realms of discovery across various scientific disciplines. This conclusion synthesizes key insights drawn from previous sections, emphasizing the multifaceted nature of resolution in imaging.
Summary of Key Points
The key elements discussed in this article include:
- Defining Resolution: Understanding what resolution entails provides a foundation for appreciating its value.
- Types of Resolution: The distinction among spatial, temporal, and spectral resolution showcases the many dimensions of imaging.
- Limitations of Current Techniques: Awareness of the challenges faced by traditional imaging methods is crucial for appreciating innovations.
- Advancements in Techniques: The introduction of super-resolution microscopy and data-driven approaches represents significant progress in enhancing images.
- Applications across Disciplines: The implications for biological research, materials science, and environmental analysis highlight the practical benefits of resolution improvement.
- Future Prospects: Emerging technologies and cross-disciplinary efforts indicate ongoing developments that could further enhance imaging capabilities.
Each of these points stresses the importance of resolution, encapsulating its impact on scientific progress.
Implications for Future Research
Looking ahead, the implications of improved resolution in scientific imaging are profound. Future research may focus on:
- Developing Next-Gen Technologies: Continued innovation in imaging technologies will likely yield breakthroughs that further refine our ability to see and analyze finer details in samples.
- Interdisciplinary Collaboration: As imaging becomes an integral part of various scientific fields, fostering collaboration among researchers can enhance the integration of diverse techniques and methodologies.
- Standardization of Protocols: Establishing common standards for reporting resolution can facilitate comparisons across studies, promoting reproducibility in research.
- Ethics of High-Resolution Data: As resolution improves, the ethical implications regarding data collection and interpretation must be considered to ensure responsible use of advanced imaging technologies.
In summary, the pursuit of higher resolution in scientific imaging is not merely about achieving clearer pictures, but about unlocking the potential for unprecedented discovery and understanding in the scientific community.