Exergy Explained: Importance and Applications
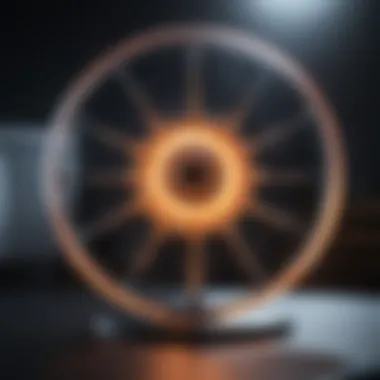
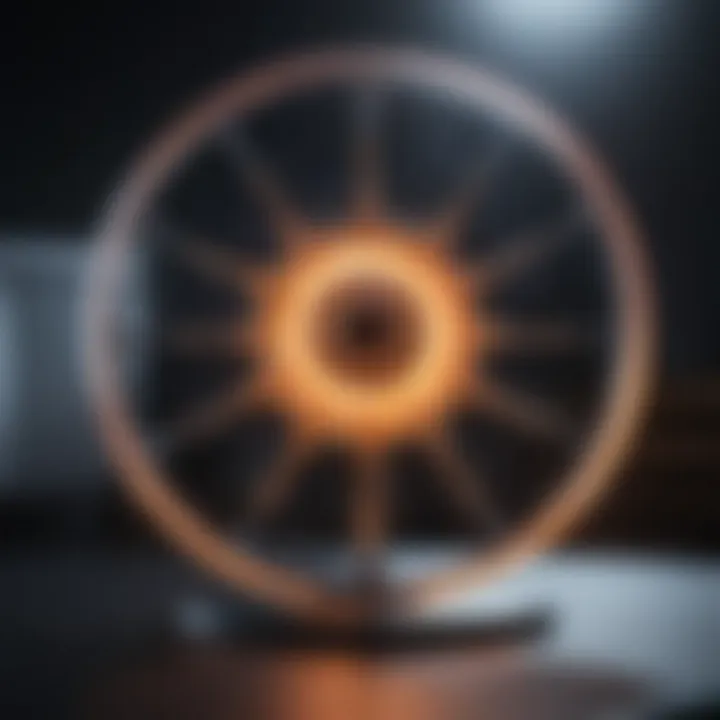
Intro
Understanding exergy is essential for anyone involved in the study or application of thermodynamics. This concept, rooted in the laws of thermodynamics, serves as a foundation for evaluating the efficiency of energy systems. From mechanical engineering to environmental science, exergy plays a vital role in determining how energy is used, conserved, or wasted in various processes. In this article, we will dissect the essence of exergy, explore its significance, and delve into its multifaceted applications across numerous fields.
Key Concepts
Definition of the Main Idea
Exergy, in simple terms, is the measure of useful energy available from a system to perform work. Unlike energy, which is conserved, exergy provides valuable insights into the useful work potential of energy resources. In essence, exergy quantifies the part of energy that can be transformed into work, taking into account the environment's state. This makes it a crucial concept that helps scientists and engineers understand energy conversion processes more comprehensively.
Overview of Scientific Principles
To ground our understanding of exergy, it's important to touch on some fundamentals of thermodynamics. Exergy is linked to the first and second laws of thermodynamics:
- First Law of Thermodynamics: This law posits that energy can neither be created nor destroyed; it merely changes forms.
- Second Law of Thermodynamics: This principle introduces the concept of entropy, indicating that energy transitions are accompanied by a loss of usable energy, or exergy, which can lead to disorder.
Essentially, exergy assesses how much of the energy input can effectively do work by comparing it against a standard, typically the surrounding environment. As such, it holds significant relevance in optimizing processes.
"Exergy can be seen as the energy that can actually generate power. Without it, we are merely sitting on a pile of unused capacity."
"Exergy can be seen as the energy that can actually generate power. Without it, we are merely sitting on a pile of unused capacity."
Current Research Trends
Recent Studies and Findings
Recent studies have shown a heightened interest in how exergy can influence energy sustainability and efficiency. Research emphasizes the importance of exergy in evaluating renewable energy sources, like solar and wind, and their integration into existing systems. It sheds light on the inefficiencies often overlooked in traditional energy systems, enabling improvements in resource utilization. For instance, recent studies stratifying exergy flow in thermal systems have highlighted how minor adjustments can yield substantial efficiency gains.
Significant Breakthroughs in the Field
Novel approaches in modeling exergy flows are proving groundbreaking. They've enabled researchers to better understand and quantify energy transformations in complex systems. Furthermore, investigations into exergy in industrial processes are revealing potential pathways for optimizing performance while reducing environmental footprints. With the increasing focus on climate change and sustainability, the application of exergy is likely to expand even further.
By engaging deeply with the principles of exergy, we can unlock greater efficiencies in energy use, ultimately advancing sustainable practices across various sectors. Incorporating this perspective not only contributes to academic research but also enhances practical applications, bridging the gap between theory and modernization.
Intro to Exergy
Exergy is a term that, while perhaps not commonly found in everyday conversation, is essential in understanding how energy systems operate efficiently. In many ways, it's the backbone of thermodynamic analysis, shedding light on how energy can be transformed and utilized in meaningful ways. This introduction serves to establish why exergy matters, specifically in a world increasingly focused on sustainability and efficient resource management.
When we discuss exergy, we are essentially talking about the potential of energy to perform useful work. This notion is crucial in various fields—from engineering to environmental science—because it helps identify real-world efficiencies and inefficiencies in energy systems. For students and professionals alike, grasping the concept of exergy unlocks a deeper comprehension of energy consumption, production, and the fundamental principles that govern these processes.
Benefits and Considerations
- Enhanced Efficiency: Understanding exergy leads to improved resource allocation and reduced waste. As we optimize systems based on exergy values, we can create more efficient machinery, power plants, and renewable energy solutions.
- Broader Applications: Exergy isn't limited only to engineering applications. It permeates fields like economics, environmental studies, and systems analysis, providing a universal framework for evaluating energy systems.
- Grounding in Theory: The fundamental definitions and historical evolution of exergy allow practitioners to base their practices on solid theoretical underpinnings, creating a bridge between academic theory and industrial practice.
In the following sections, we will delve deeper into the definition of exergy and its historical development, which form the foundation of our understanding of this vital concept.
Definition of Exergy
Exergy is often defined as the maximum useful work obtainable from a system as it comes into equilibrium with its surroundings. In simpler terms, while energy is conserved, exergy quantifies the energy available to do work. To illustrate this:
- If you have a cup of hot coffee, the energy stored in it is its thermal energy. However, the ability to convert that thermal energy into work (say, by heating a room) is where exergy comes into play. As the coffee cools, its exergy diminishes until it reaches ambient temperature, at which point it can no longer perform useful work.
This example highlights that not all energy is created equal; the context and conditions of that energy dictate its quality and potential for work. Exergy acts as a measure for this quality, offering a more nuanced view than traditional energy metrics, which can sometimes be misleading.
History and Evolution of the Concept
The concept of exergy was brought into the world of thermodynamics by the physicist Rudolf Clausius in the mid-19th century. Clausius's work laid the groundwork for thermodynamic laws, but the explicit term 'exergy' was coined much later by George A. E. O. K. V. Szargut in the 1950s, who sought to establish a clearer framework for evaluating energy efficiency.
From its inception, the idea of exergy has evolved significantly:
- Early Understandings: Initially rooted in thermodynamic theory, exergy analysis was primarily a theoretical exploration.
- Practical Applications: Over time, engineers and scientists recognized its practical implications, applying exergy in the design and optimization of thermal systems. This transition marks a significant shift in how energy systems are conceived.
- Modern Developments: Today, exergy analysis plays a vital role in sustainable design and renewable energy. It informs research initiatives and guides policy-making aimed at reducing energy consumption and enhancing efficiency.
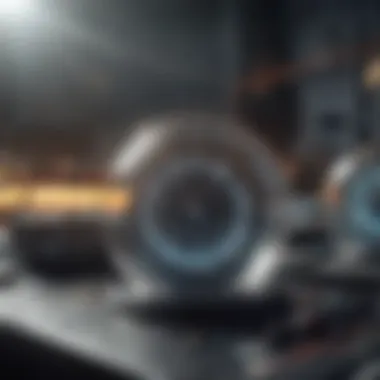
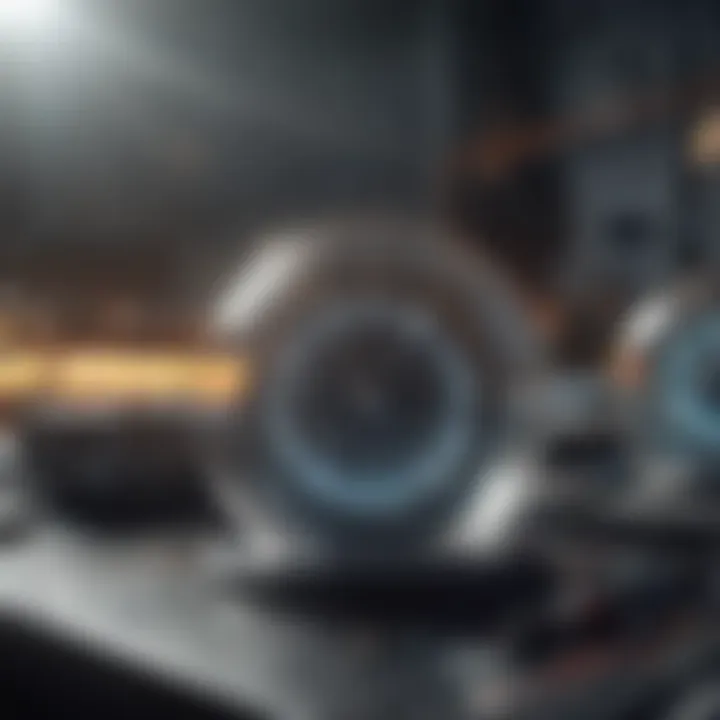
In summary, understanding exergy is relevant not just for the sake of theory, but also in practical terms to motivate and shape our approaches toward energy use today.
Theoretical Framework
Delving into the theoretical framework of exergy is akin to mapping the landscape of energy transformations and efficiencies. It sets the stage for a comprehensive understanding, highlighting why exergy is not merely an abstract concept but a critical tool for interpreting the world of energy systems. This section lays the groundwork, explaining its relevance for students, researchers, educators, and professionals alike. When considering energy conversions, the theoretical aspects elucidate how the principles of thermodynamics inform the allocation and utilization of energy resources. Grasping this framework is vital for developing effective strategies in energy management.
First and Second Laws of Thermodynamics
To fully appreciate exergy, one must first understand the bedrock of thermodynamic principles—the First and Second Laws. The First Law, often known as the Law of Energy Conservation, posits that energy cannot be created or destroyed; it only transforms from one form to another. For example, when burning a fuel, its chemical energy converts to thermal energy, which can subsequently turn into kinetic energy if used to propel a vehicle.
The Second Law introduces a different flavor to the energy conversation. It states that energy transformations are inherently efficient, leading to an increase in entropy—or disorder—within a closed system. In essence, while energy may be conserved, its quality deteriorates over time. This concept is crucial to understanding exergy because exergy quantifies the maximum useful work obtainable from a system as it moves toward equilibrium. It determines not only how much energy is available but also how well that energy can be utilized.
"Exergy is the bridge that converts theoretical energy principles into practical applications."
"Exergy is the bridge that converts theoretical energy principles into practical applications."
This relationship between the laws shapes energy systems, highlighting that in any given process, the focus should be on not just the energy involved but also the quality of that energy. Recognizing these laws allows engineers and scientists to design more efficient systems, accommodating the reality that not all forms of energy are created equal.
Energy vs. Exergy
When it comes to distinguishing between energy and exergy, clarity is key. Energy is the capacity to perform work, while exergy refers to the quality of that energy—its potential to do useful work in a given context. For instance, a large amount of thermal energy might sound impressive, but if it is at a low temperature, its ability to perform work diminishes significantly.
In several practical applications, this distinction matters more than it may seem at a glance:
- Thermal Power Plants: These plants might consume large amounts of fuel, but if their design doesn’t capture exergy efficiently, they waste a good deal of potential work.
- Refrigeration Systems: High-quality exergy allows these systems to maintain temperature differentials effectively, preserving energy while users benefit from cool environments.
- Renewable Energies: Solar panels harness energy, yet without considering exergy, the potential efficiency of solar energy remains underestimated.
Understanding these nuances informs decisions across various fields – from renewable energy implementation to industrial engineering. By providing crucial insights into how systems can be optimized, this differentiation ultimately promotes sustainability and efficiency.
Calculation of Exergy
Understanding how to calculate exergy is crucial in grasping its importance in thermal systems and overall energy efficiency. This section delves into various formulations of exergy for different systems, emphasizing that precise calculations can lead to noteworthy improvements in optimization and sustainability efforts. Given that exergy references the quality of energy and its ability to perform work, knowing how to measure it accurately is fundamental for engineers and scientists. By identifying exergy losses, one can highlight inefficiencies and reduce waste in both industrial processes and energy systems.
Exergy Formulations for Different Systems
When exploring exergy, one must consider that it takes different forms depending on the system at hand. For instance:
- Closed Systems: Exergy is typically derived from the difference in energy states between the system and its environment. In this scenario, formulas like the Gibbs free energy are often involved.
- Open Systems: In systems such as those involved in combustion or chemical reactions, exergy calculations must account for matter and energy flows. Formulations would include the contributions from both thermal and chemical potentials.
- Thermal Systems: Here, exergy calculations tend to emphasize temperature differences. The Carnot efficiency equation can shed light on the maximum potential work output available from a temperature differential.
The formulas may seem daunting, but here’s a streamlined representation for a simple thermal system:
[
E_x = E_total - T_0(S_sys - S_sur)
]
where
- (E_x) is the exergy,
- (E_total) represents the total energy,
- (T_0) is the environment temperature,
- (S_sys) and (S_sur) are the total entropy of the system and surroundings, respectively.
This formulation serves as a foundational piece for understanding exergy across several applications.
Factors Affecting Exergy Values
The calculation of exergy does not exist in a vacuum; instead, it is influenced by various factors that can significantly alter the exercise of energy systems. Here are some key elements to consider:
- Temperature Difference: The greater the temperature difference between a system and its surroundings, the higher the exergy value. This means effective use of heat sources can maximize work potential.
- Pressure Variations: In many processes, especially gas compression or expansion, pressure changes can also contribute to changes in exergy. Precise control over pressure can become a tool in optimizing performance.
- Phase Changes: For systems involving phase transitions, such as evaporation or condensation, the exergy values can shift dramatically. The specific heat capacities of materials involved also come into play.
- Material Properties: Different materials have intrinsic properties that affect their ability to store and transfer energy. This naturally influences exergy calculations.
"Understanding the influencing factors of exergy makes it possible to optimize performance and reduce waste in energy systems."
"Understanding the influencing factors of exergy makes it possible to optimize performance and reduce waste in energy systems."
Exergy in Energy Systems
The examination of exergy within energy systems holds immense significance as it offers a nuanced understanding of the efficiency and sustainability of various processes. By focusing on how energy is utilized, we can better appreciate the role of exergy in transforming resources into functional energy. Unlike mere energy, which tells how much can be harnessed, exergy reveals the quality and usability of that energy. This understanding is crucial for developing strategies that lead to improved efficiency, lower waste, and ultimately, a more sustainable future.
Applications in Power Generation
Power generation stands as a cornerstone of modern society, fueling everything from households to massive industrial operations. In this landscape, exergy serves as a vital tool for dissecting and enhancing the performance of different power generation methods.
- Thermal Power Plants: Traditional thermal plants often deal with energy conversion losses. By employing exergy analyses, engineers can pinpoint where energy is dissipated and implement solutions that bolster efficiency. This might include optimizing the heat exchange processes or looking into cogeneration systems, where both electrical and thermal energy is produced simultaneously.
- Nuclear Power: In the realm of nuclear energy, exergy helps in evaluating the effectiveness of the reactor design. As these systems operate under strict constraints, understanding which aspects of the process are most exergically inefficient can lead to design improvements that enhance operational output while ensuring safety.
- Fossil Fuels: Even with fossil fuel-based power plants, exergy can guide better fuel utilization and cleaner combustion technologies. Analyzing exergy balances allows the calculation of losses in various components like boilers and turbines, promoting innovations that reduce environmental impact.
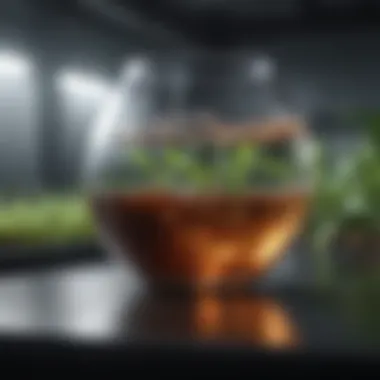
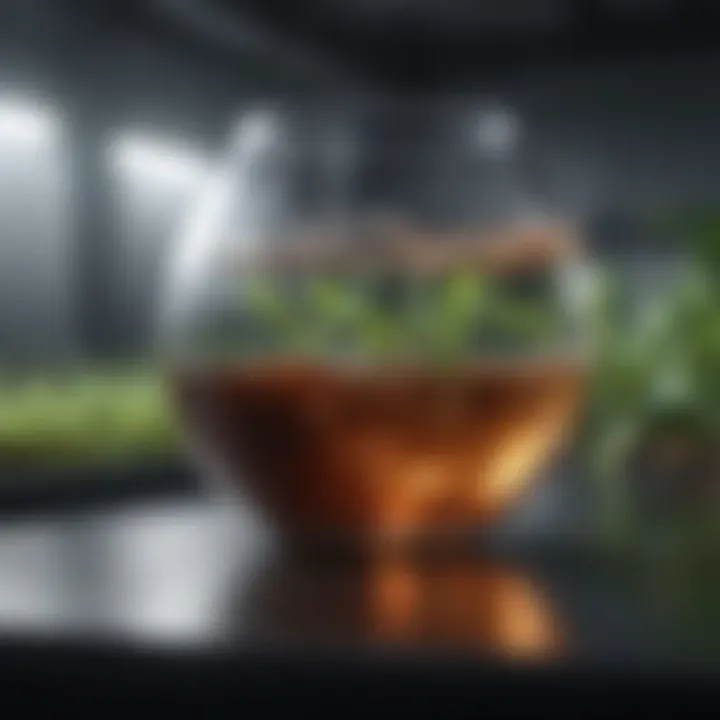
The bottom line is that exergy analysis provides a clear, structured method for evaluating how effectively energy resources are employed and how potential losses can be curtailed, encouraging a deeper dive into efficiency improvements.
Exergy Analysis of Renewable Resources
Shifting focus to renewable resources, such as solar, wind, and hydroelectric systems, exergy analysis emerges as a critical evaluator of performance, guiding adaptations that sustain environmental integrity while maximizing yield.
- Solar Power: In solar energy systems, exergy assessments help in optimizing photovoltaic (PV) panels and concentrating solar power (CSP) systems. For instance, understanding the exergy losses due to thermal emissions allows designers to enhance thermal storage and improve overall efficiency.
- Wind Energy: In wind farms, exergy can be instrumental in analyzing turbine performance in different wind speeds. For example, determining the exergy output versus the kinetic energy available in the wind can direct efforts toward site selection and turbine design for optimized power generation.
- Hydropower: Similar to other forms, hydropower also benefits from exergy evaluations. By assessing the exergy output in relation to the height of water falls and flow rates, operators can fine-tune operations for peak efficiency, particularly in variable water conditions.
Above all, integrating exergy considerations into renewable energy systems not only bolsters production but also aligns closely with sustainability goals, proving that effective resource management can yield both environmental and economic benefits. This approach resonates widely across all forms of sustainable practices, lending credibility and depth to exergy as a concept in energy discourse.
Exergy allows for a comprehension of not only how much energy is available but also how viable that energy is for our needs, making it a central theme in sustainable energy practices.
Exergy allows for a comprehension of not only how much energy is available but also how viable that energy is for our needs, making it a central theme in sustainable energy practices.
Industrial Applications of Exergy
Exergy is not just an abstract concept in thermodynamics; it has real-world applications in various industrial sectors. Understanding exergy can lead to significant improvements in efficiency, resource utilization, and sustainability. Firms that incorporate exergy analysis into their operations often discover substantial reductions in waste and energy consumption, leading to both economic and environmental benefits. This section delves deeply into how exergy can be applied in industrial settings, particularly focusing on process optimization and performance metrics that enhance productivity.
Process Optimization Using Exergy
In many industrial operations, various processes consume energy, but they do so inefficiently. By applying exergy concepts, industries can optimize these processes, leading to enhanced overall performance. Exergy analysis allows an organization to assess where energy losses occur during various stages of production. For example, during heating or cooling processes, significant amounts of energy might be wasted.
In practical terms, consider a typical chemical production facility. By evaluating each stage using exergy metrics, engineers can pinpoint inefficiencies—areas where the actual output does not match the input energy in a meaningful way. Using this analysis, modifications can be made, such as adjusting temperatures, improving insulation, or even redesigning equipment to salvage lost energy. A key takeaway here is that understanding how much useful work you’re getting from your energy input directly correlates with the sustainability of the operation.
Key Steps in Process Optimization:
- Identify Optimal Operating Conditions: Find conditions that maximize the useful output of energy.
- Evaluate Energy Losses: Use exergy to determine where inefficiencies occur.
- Implement Design Changes: Revamp systems based on exergy analysis outcomes.
- Monitor and Adjust: Regularly check performance metrics to ensure continued efficiency.
In many cases, integrating exergy-based optimizations leads to a reduction in operational costs and an increase in the reliability of processes.
Exergy-Based Performance Metrics
When you think about improving industrial processes, having the right metrics is crucial. Traditional metrics often focus on energy consumption, but they may not convey the full picture. That's where exergy-based performance metrics come in. These metrics can provide more insightful evaluations of energy efficiency by taking into account the quality of energy, not just the quantity.
For instance, let's compare two power plants: one using coal and another using solar. Simply looking at energy output may indicate that the coal plant is more efficient because it generates more energy per unit of coal consumed. However, a deeper dive using exergy calculations can reveal that the solar plant, despite its lower energy output, makes better use of energy and contributes less to ecological damage.
Some valuable exergy performance metrics include:
- Exergy Efficiency: The ratio of useful exergy output to the exergy input.
- Specific Exergy: Exergy per unit mass of the product or service.
- Exergy Destruction Rate: Measures the loss of potential useful work due to irreversibilities in processes.
“By applying exergy-based metrics, industries can transform the way they evaluate their energy systems, leading to better decision-making and strategic improvements.”
“By applying exergy-based metrics, industries can transform the way they evaluate their energy systems, leading to better decision-making and strategic improvements.”
Understanding and utilizing these metrics allows companies to structure their operations around maximizing exergy efficiency, thereby minimizing waste and boosting profitability. They can serve as benchmarks for continuous improvement initiatives within organizations.
In summary, the integration of exergy into industrial processes not only aids in optimizing operations but also fosters a culture of sustainability and accountability. Manufacturers that embrace this methodology may well find themselves ahead of the curve, leading to a more sustainable and economically viable future.
Exergy and Sustainability
Understanding exergy's influence on sustainability introduces a valuable lens through which we can examine resource efficiencies in various systems. Exergy provides not just a measure of potential work within a system, but also a framework that closely aligns with sustainability principles. In a world increasingly aware of environmental limits and resource allocation, exergy emerges as a fundamental driver towards more sustainable practices.
For instance, energy systems often pivot around the idea of power output, often neglecting how effectively resources are utilized. By focusing on exergy, we illuminate the pathways that maximize useful work while minimizing entropy generation. This focus on efficiency translates into less waste and promotes renewable practices.
Key considerations for integrating exergy into sustainability efforts include:
- Understanding that not all energy is equal; exergy highlights the quality and usability of energy, rather than just its quantity.
- Incorporating exergy analysis in system design ensures that sustainable technologies are not just effective but also resource-efficient.
- Developing metrics that reflect actual sustainability through exergy can drive innovation and policy-making in industries from manufacturing to waste management.
"Exergy is a bridge that connects the theoretical tenets of thermodynamics with the tangible realities of sustainability."
"Exergy is a bridge that connects the theoretical tenets of thermodynamics with the tangible realities of sustainability."
Thus, exergy analysis is pivotal in crafting systems that align not just with modern capabilities but also with future eco-friendly practices. The realization that each joule of energy can have variable worth leads us to more measured approaches in resource consumption, thus paving the way for long-term sustainable solutions.
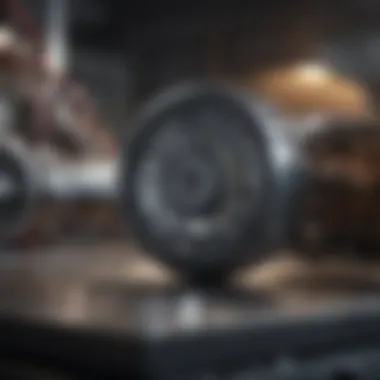
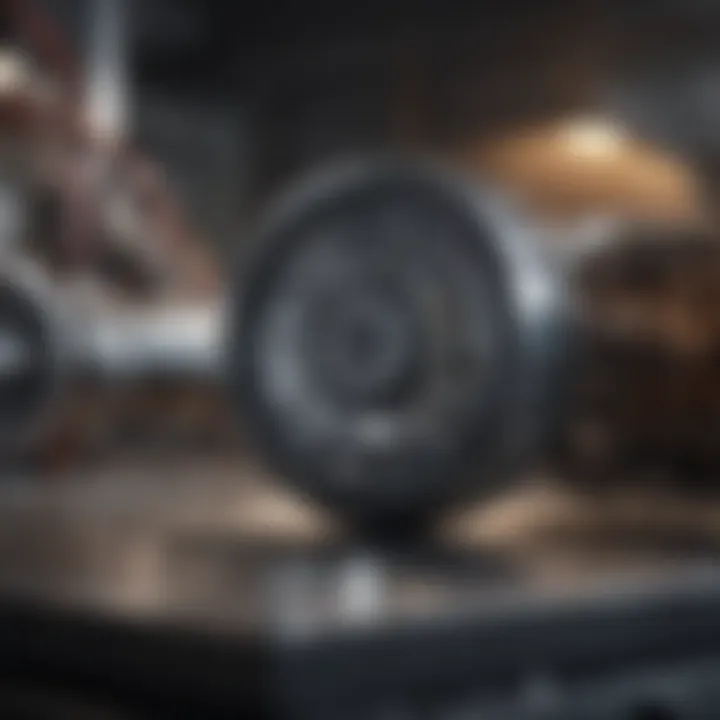
Measuring Sustainability through Exergy
The actual measurement of sustainability through exergy is intricate, yet essential. Evaluating how much useful work can potentially be extracted from energy resources immediately shifts the discussion from quantity to quality. This perspective becomes vital in dynamic fields like renewable energy, where resources vary not just in type (solar, wind) but also in capability and availability.
A few key metrics to consider when measuring sustainability through exergy include:
- Exergy Efficiency Ratio: This ratio compares the useful energy produced to the total exergy input, providing direct insight into operational efficiency.
- Exergy Destruction Analysis: This looks at where energy is wasted in processes and identifies opportunities for improvements.
- Life Cycle Assessment Using Exergy: Applying exergy evaluations throughout a product's lifecycle enhances understanding of sustainability impacts from production to disposal.
With these measurements, organizations can track progress and recalibrate strategies towards energy systems that contribute positively to ecological and societal goals.
Role in Waste Management
The role of exergy in waste management cannot be understated. Exergy analysis enables a clearer perspective on how much potential energy is being lost in waste streams and reveals avenues for recovery and reuse. In essence, it promotes a shift from viewing waste as merely refuse to recognizing it as a resource waiting for an opportunity to be converted back into useful energy or material.
Consider the following in waste management:
- Exergy Approach to Recycling: Using exergy to assess the recycling processes can help identify inefficiencies and enhance the quality of recycled products.
- Waste-to-Energy Technologies: In these systems, exergy helps determine the optimal methods for converting waste into heat, electricity, or fuel, ensuring maximum retrieval of energy during processing.
- Circular Economy Transition: Understanding the exergy potential of discarded materials aligns perfectly with circular economy principles, where waste becomes a valuable asset rather than a liability.
Overall, integrating exergy principles into waste management practices promotes smarter resource use and aligns with broader sustainability goals. This innovative approach ensures that systems not only mitigate environmental impacts but also create economic value from waste.
By embracing the concept of exergy, both in sustainability assessments and waste management strategies, industries can progress towards a more sustainable future, one where resource utilization is optimized and ecological footprints are minimized.
Limitations and Challenges
Understanding the limitations and challenges surrounding exergy is crucial for grasping its full implications in various practical contexts. As much as exergy presents a powerful framework for assessing energy systems' efficiency, it's important to acknowledge that misconceptions and measurement difficulties can hinder its effective application. Addressing these concerns can lead to more precise utilization of exergy concepts in both research and industry.
Common Misconceptions about Exergy
Many people hold misconceptions about exergy that can lead to confusion. A prevalent myth is that exergy and energy are one and the same, which they are not. While energy represents the total capacity to perform work, exergy refers specifically to the portion of energy that can actually be converted into work, given the constraints of the environment. It's like comparing apples to oranges – both are essential but serve different purposes.
Another misconception involves the assumption that exergy can only be applied in high-tech scenarios. In reality, the principles of exergy can be utilized in many everyday processes, from heating systems to food preservation methods. This broad applicability is often overlooked, leading many to disregard its practical value in optimizing even simple systems.
Furthermore, some may argue that exergy assessments are only relevant for large-scale engineering projects. However, small systems, even household appliances, can benefit significantly from exergy analyses. It’s essential to debunk the myth that only complex systems require this level of examination.
"The real measure of progress is not whether we add more to the abundance of those who have much; it is whether we provide enough for those who have too little." - Franklin D. Roosevelt
This quote reflects the spirit of utilizing exergy to enhance efficiency for everyone, not just the advanced technologies.
"The real measure of progress is not whether we add more to the abundance of those who have much; it is whether we provide enough for those who have too little." - Franklin D. Roosevelt
This quote reflects the spirit of utilizing exergy to enhance efficiency for everyone, not just the advanced technologies.
Technical and Practical Challenges in Measurement
When it comes to measuring exergy, the path can be quite bumpy. The first hurdle often involves the complexity of obtaining accurate data for the systems being evaluated. The exergy content of a substance depends on its temperature, pressure, and chemical state, requiring precise conditions to measure effectively.
Moreover, the mathematical formulations needed to calculate exergy can intimidate even seasoned professionals. Given that methods can vary depending on the system type, it gets tricky for practitioners who might not have the theoretical background. This obstacle can result in inaccurate predictions or analyses, thereby limiting the technique's potential benefits.
Another issue that cannot be ignored consists of varying definitions and interpretations of exergy across disciplines. For instance, environmental scientists may define exergy differently than mechanical engineers. This variability can lead to misunderstandings or conflicts, complicating collaborative efforts in interdisciplinary projects.
In summary, tackling these limitations and challenges is fundamental for harnessing the full power of exergy. By improving our understanding and addressing misconceptions, we can enhance the effectiveness of exergy analyses. As we navigate through various complexities in measurement, the goal should always be to refine our approaches and broaden the application of exergy in the hope of increased efficiency and sustainability in energy systems.
Future Directions
The exploration of exergy transcends the traditional boundaries of thermodynamics, earmarking its significance in various upcoming domains. Recognizing exergy’s potential opens avenues for innovation and enhances our understanding of energy efficiency in diverse contexts. The dialogue surrounding future directions in exergy not only highlights critical research areas but also emphasizes the necessity for technological advancements that can redefine energy usage. As we look forward, the intersection of exergy with contemporary challenges in sustainability and efficiency will warrant attention from researchers and industry professionals alike.
Emerging Research Areas
Research into exergy is no longer a niche topic; it’s gaining traction among energy economists, environmentalists, and engineers. Here are some pivotal areas where emerging research is keenly focused:
- Integration with Renewable Energy: How can exergy analysis refine the processes involved in solar and wind energy? Society is more inclined toward renewables, allowing exergy principles to optimize resource utilization.
- Life Cycle Assessment: The link between exergy and life cycle assessment of products may offer valuable insights. This necessitates evaluating not just the energy consumed but also the quality of energy within the lifecycle.
- Exergy in Industrial Processes: Industries are increasingly apt to use exergy as a performance metric. Research focusing on how exergy can streamline and enhance manufacturing operations is becoming pivotal in sectors like chemical engineering.
- Climate Change Mitigation: With climate issues on the rise, employing exergy to develop climate-responsive strategies presents a genuinely promising research path. The balance between GDP growth and ecological impacts can heavily benefit from such insights.
Potential for Technological Innovations
The landscape of technology is ripe for transformation, particularly through the lens of exergy. Recognizing and leveraging exergy could catalyze breakthroughs in various technological sectors:
- Smart Grid Technology: Implementing exergy analysis could lead to more efficient electricity distribution systems. This aspect holds significant promise in optimizing supply chains and grid management.
- Energy Storage Solutions: As technology is developed to store energy more efficiently, exergy assessments can contribute to better design and function of batteries and other storage mediums.
- Carbon Capture Technologies: Innovations in mitigating carbon emissions through exergy principles might drive improvements in operations that seek to enhance energy recovery while reducing the carbon footprint of industries.
- Desalination Processes: As water scarcity intensifies, harnessing exergy in desalination technology can optimize the energy needed for transforming saline water into fresh water, critically benefiting regions suffering from droughts.
"Understanding the future of exergy leads us not only to technological innovations but also prepares us for challenges we have yet to face."
"Understanding the future of exergy leads us not only to technological innovations but also prepares us for challenges we have yet to face."
In summation, the future directions of exergy research and application are geared toward making significant impacts on energy efficiency and sustainability. By diving into emerging fields and nurturing technological innovations, researchers and practitioners can not only adapt but also thrive in an ever-evolving landscape.