Exploring the Intricacies of Shock Machines: Design and Application
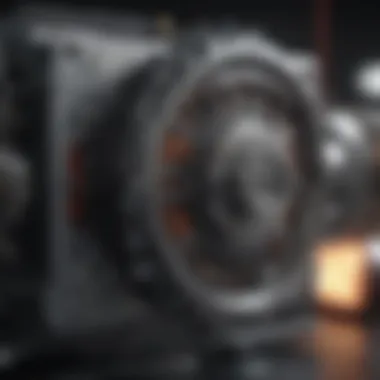
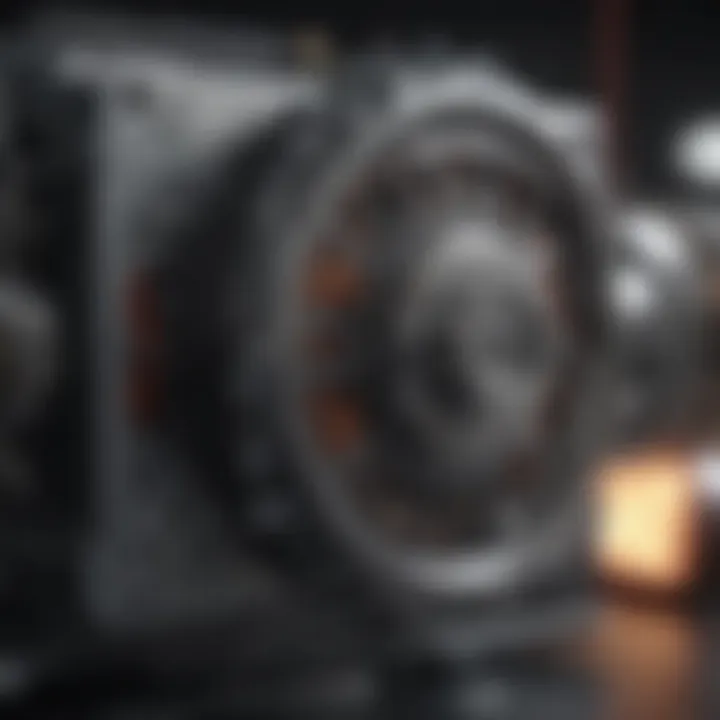
Intro
This article discusses shock machines, devices that produce a rapid and controlled change in pressure or force. These machines have a myriad of applications, particularly in scientific experiments. Using shock machines effectively requires an understanding of both their design principles and their functionalities. Key to this understanding is knowledge of scientific theory and practical application.
Key Concepts
Definition of the Main Idea
Shock machines generate shock waves, which are abrupt changes in pressure within a medium. This phenomenon can be utilized in various fields including materials science, engineering, and physics. They help in simulating conditions that materials will face during events like explosions or high-speed impacts. Understanding shock machines can provide insights into material behavior under extreme conditions.
Overview of Scientific Principles
The operation of shock machines is grounded in physics principles. Key concepts include:
- Wave propagation: Light and sound waves behave differently when traveling through various materials.
- Energy transfer: Shock machines deliver energy instantly, creating a necessary force for experimentation.
- Mechanical properties: Different materials respond uniquely to shock waves, which aids in material testing.
The effects of shock waves can be related to the speed and intensity of the wave, and how they interact with different substances.
Current Research Trends
Recent Studies and Findings
Research in shock machines continues to evolve. Recent studies have focused on improving efficiency, accuracy, and expanding their applications. For instance, studies from institutions like the Massachusetts Institute of Technology (MIT) have provided new insights into the dynamics of shock waves in non-linear materials.
Significant Breakthroughs in the Field
Recent advancements include:
- Innovative designs: New prototypes enhance precision in wave generation.
- Computational models: Advanced simulations offer deeper understanding of wave behavior, leading to better predictive models.
- Interdisciplinary applications: Researchers now utilize shock machines in fields like biology and medicine, expanding their usability.
This multidisciplinary approach not only fosters collaboration but also accelerates discovery in diverse areas of research.
This multidisciplinary approach not only fosters collaboration but also accelerates discovery in diverse areas of research.
Epilogue
Understanding the dynamics of shock machines is essential for their effective application in various scientific and engineering fields. The ongoing research and developments indicate a promising future for these machines, enhancing their capabilities and applications.
Preamble to Shock Machines
Shock machines play a significant role in various scientific fields by facilitating the creation and analysis of shock waves. These machines are not merely tools; they represent a convergence of physics, engineering, and applied science aimed at solving complex problems. Their relevance extends beyond academic curiosity; they have practical applications in designing safer structures, enhancing materials, and advancing medical technology. Understanding the core functionality and design of shock machines brings numerous benefits to researchers and professionals alike.
Firstly, shock machines enable scientists to conduct controlled experiments that illuminate the behavior of materials under extreme conditions. This leads to invaluable data applicable in various industries, including aerospace, automotive, and biomedical fields. Furthermore, gaining insights into the dynamics of shock waves allows for improved safety protocols and innovative solutions in engineering design.
In addition, considering the evolution of these machines contributes to a broader comprehension of technological advancements. As shock machines become more refined, they embrace new materials and integration with digital technology, enhancing their effectiveness and efficiency. This evolution underscores the necessity for ongoing research and development in this domain.
Finally, understanding the historical context of shock machines informs current practices and inspires future innovations. Acknowledging past advancements enables present-day professionals to build on the legacy of scientific inquiry and technological evolution.
The Physics Behind Shock Machines
Understanding the physics behind shock machines is crucial for comprehending their functionality and effectiveness in various applications. Shock machines utilize the physics of shock waves and energy transfer to achieve their objectives. This segment of the article will elaborate on the fundamental principles governing shock waves and the mechanisms through which energy is transferred in these systems.
Basic Principles of Shock Waves
Shock waves are high-pressure waves that travel through mediums, often resulting from sudden changes in pressure or velocity. The formation of a shock wave occurs when an object moves through a medium at a speed greater than the speed of sound in that medium. This leads to compressed air and a rapid increase in pressure, creating a wave front that travels away from the source.
A shock wave is characterized by several key properties:
- Wave Front: This is the leading edge of the shock wave, where properties such as pressure, temperature, and density increase sharply.
- Strength of the Wave: The intensity of a shock wave depends on the speed of the moving object and the characteristics of the medium it is traveling through.
- Supersonic Flow: In many shock machines, the goal is to create or utilize supersonic flows, where the speed exceeds the local speed of sound.
Understanding these principles is essential not only for engineers and physicists but also for those involved in industries utilizing shock machines. The ability to control shock waves can enhance performance, leading to advancements in fields ranging from aerospace to materials science.
Energy Transfer Mechanisms
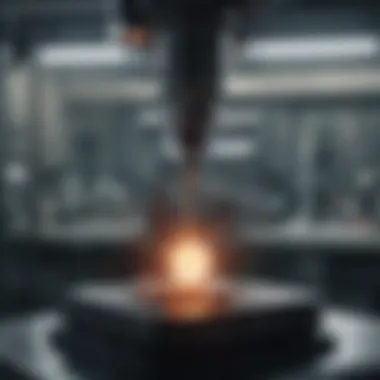
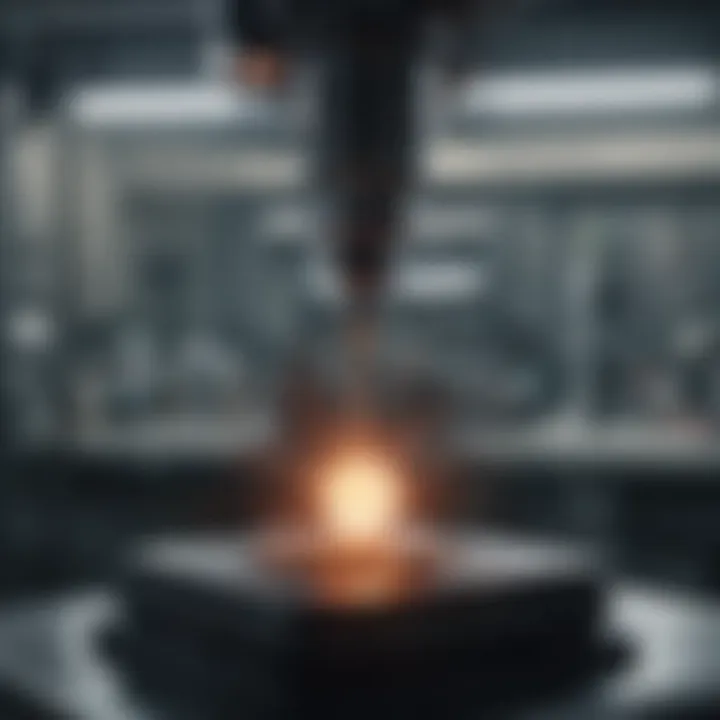
Energy transfer in shock machines occurs through various mechanisms that depend on the nature of the shock wave and the materials involved. The transfer of energy can occur in both elastic and inelastic forms, depending on how the medium interacts with the wave.
Some significant mechanisms include:
- Kinetic Energy Transmission: As a shock wave travels, it carries kinetic energy, affecting the motion of particles in the medium. This can lead to various practical applications, including propulsion technologies.
- Thermal Energy Changes: The rapid increase in pressure from a shock wave can result in temperature rises, which facilitates processes such as welding and cutting.
- Phase Transitions: In some materials, shock waves can induce phase transitions, altering physical states from solid to liquid or gas. This is particularly relevant in material testing to evaluate properties under extreme conditions.
The understanding of these energy transfer mechanisms is critical. It informs the design of shock machines and enhances their operational effectiveness, allowing for more precise applications in experimental physics and engineering.
Design Elements of Shock Machines
The design of shock machines plays a crucial role in determining their effectiveness and functionality. Diligent consideration of various design elements is essential for optimizing performance while ensuring safety and reliability. Understanding the design components can greatly impact the desired output of shock machines, and thus their applications in both experimental and practical scenarios.
Components and Materials
The components of shock machines are diverse and directly influence their operational efficacy. Several key parts must be present and carefully constructed:
- Casing: The outer structure must withstand the forces generated during operation. Common materials include steel and specialized polymers; these materials offer both strength and some flexibility.
- Energy Source: Shock machines often rely on mechanical, hydraulic, or electrical energy sources. Precision in the selection of the energy source is vital, as it affects the energy transfer efficiency.
- Shock Generation Mechanism: This is central to the function of the machine. Mechanical systems might include springs or hydraulic rams. Meanwhile, electrical devices often utilize capacitors to produce rapid bursts of energy.
- Safety Shields: These protect operators from any potential hazards. Often, transparent polycarbonate is used to allow visibility while ensuring safety.
The materials chosen for these components must exhibit notable qualities such as durability, shock resistance, and heat dissipation. Recent explorations into advanced composites are promising, as they may enhance the performance and longevity of these machines.
Engineering Considerations
Engineering considerations are paramount when designing shock machines. These factors ensure that equipment is both functional and safe. Here are several important aspects:
- Load Calculation: Understanding how much load the machine will handle is fundamental. Incorrect calculations can lead to catastrophic failures.
- Dynamic Analysis: Analyzing how shock waves propagate through materials is vital in optimizing design and material selection. Engineers use various simulation tools to predict the behavior during operation.
- Regular Maintenance Protocols: Machines must be designed for easy access to components for repair or replacement. This consideration saves time and resources in the long run.
- Environmental Impact Assessments: Assessment of how the machine will perform in various environments ensures better longevity and efficiency. Strain caused by temperature fluctuations, humidity, or other conditions must be accounted for.
Effective design practices in shock machine engineering enhance operational efficiency while reducing risks associated with their use.
Effective design practices in shock machine engineering enhance operational efficiency while reducing risks associated with their use.
In summary, the intricate design elements of shock machines encompass various components and substantial engineering insights. This attention to detail ensures robust functionality, paving the way for more efficient applications in diverse fields like experimental physics, material testing, and even medical devices.
Types of Shock Machines
Understanding the types of shock machines is crucial for appreciating their applications and functionalities. These machines have varied designs, each suited for specific operational contexts and outcomes. By categorizing shock machines into mechanical and electrical types, we can better analyze their unique features, advantages, and challenges.
Mechanical Shock Machines
Mechanical shock machines utilize physical components to generate shock waves. The mechanism typically involves kinetic energy transfer through a system of levers, weights, or springs. This design allows for the creation of high-intensity shock waves, essential in fields like material testing and protective engineering.
The benefits of mechanical shock machines include:
- Simplicity in design, often leading to lower production costs.
- High reproducibility of results, essential in experimental setups.
- Ability to create controlled environments for the testing of materials under shock loading.
However, there are notable considerations:
- Maintenance can be complex, especially if the components wear out.
- The energy output can be less precise compared to electrical systems.
Electrical Shock Machines
Electrical shock machines, on the other hand, rely on electrical energy to produce shock waves. These machines usually feature sophisticated circuits and control systems. By using capacitors and rapid discharge mechanisms, electrical machines can achieve precise control over shock intensity and duration.
Key advantages of electrical shock machines include:
- Enhanced versatility, as they can be easily adjusted for different shock profiles.
- Automated operation, allowing for increased efficiency in experiments.
- Reduced manual intervention, which minimizes operator error.
Yet, these machines also pose some challenges:
- Higher initial costs associated with advanced technology.
- Require a stable power supply to function effectively.
"The distinction between mechanical and electrical shock machines highlights the diverse approaches within the field, each contributing to advances in experimental physics and engineering."
"The distinction between mechanical and electrical shock machines highlights the diverse approaches within the field, each contributing to advances in experimental physics and engineering."
Applications of Shock Machines
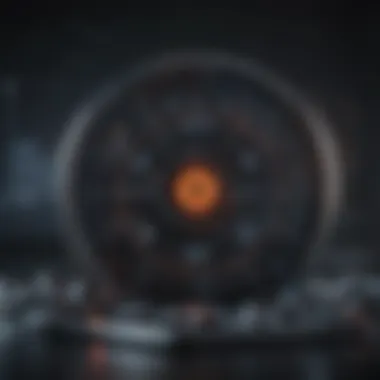
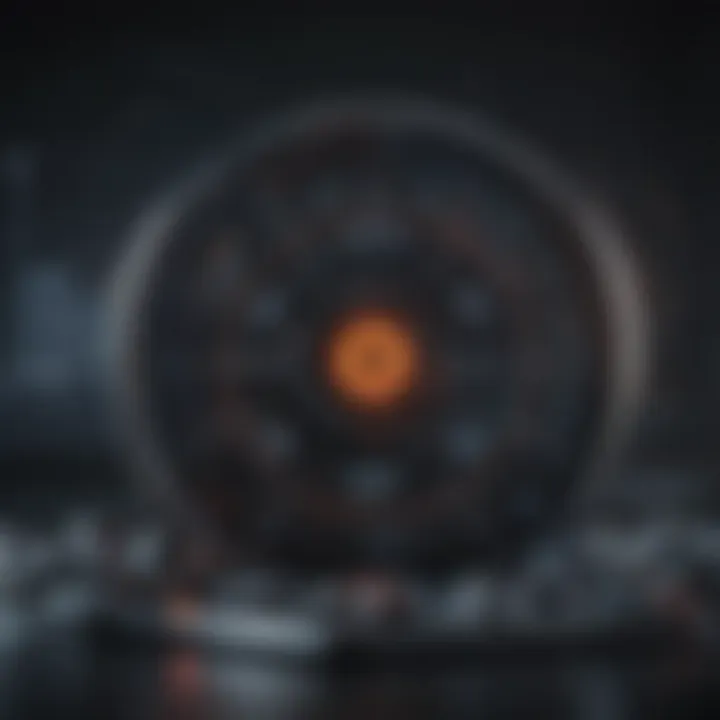
The exploration of shock machines extends beyond basic theoretical principles. Understanding their applications helps in comprehending their significance across various fields. Shock machines are crucial in several domains including experimental physics, material testing, and medical devices. This section discusses these applications in detail to underline both their importance and implications.
Experimental Physics
In experimental physics, shock machines serve as essential tools for studying high-energy phenomena. They create controlled shock waves that allow researchers to investigate material behavior under extreme conditions. This includes exploring the properties of materials at high pressure and temperature fundamentally influencing various scientific fields.
Specifically, shock machines enable fast experiments that reveal how materials react almost instantaneously. These insights can lead to advancements in a range of applications, from aerospace engineering to defense technologies. Moreover, the capability to generate repeatable results fosters confidence in experimental outcomes, thus enhancing scientific reliability.
Material Testing
The purpose of material testing using shock machines cannot be overstated. Industries require rigorous testing of materials to determine their durability and resilience. Shock machines facilitate simulating real-world conditions that materials might face during their lifecycle. This allows scientists and engineers to evaluate properties such as toughness, strength, and elongation.
Among the key benefits are:
- Durability Assessment: Identifies how materials survive under stress.
- Predictive Analysis: Provides data to forecast material behavior.
- Quality Control: Ensures materials meet particular industry standards.
Understanding the reliability of materials leads to better designs and safer products. This is especially significant for sectors like automotive and construction, where failure can result in catastrophic consequences.
Medical Devices
Shock machines are also increasingly used in the medical field. They are integral in developing devices such as extracorporeal shock wave lithotripsy. This method uses shock waves to break down kidney stones non-invasively, demonstrating how shock machines can significantly enhance patient care.
Additionally, research in trauma treatment has highlighted the potential of shock waves in healing tissues. The applications extend to regenerative medicine, where controlled shock applications may stimulate cellular repair and regeneration. This emerging avenue shows promise not only in improving recovery times but also in potentially minimizing the need for invasive procedures.
Operational Considerations
Operational considerations are critical when discussing shock machines. These machines, which harness the principles of shock waves, require careful management to ensure they function effectively and safely. This section will explore the elements under this category, including safety protocols and regular upkeep, which are essential for optimal operation.
Safety Protocols
Understanding safety protocols is vital for anyone operating shock machines. These machines generate intense pressure and can create hazardous conditions if not handled properly. Several protocols should be established and adhered to, including:
- Personal Protective Equipment (PPE): Operators must wear appropriate gear such as gloves, goggles, and lab coats to minimize risk.
- Training: Comprehensive training programs are essential, ensuring all staff are familiar with machine operation and emergency procedures.
- Emergency Shut-off Systems: Installing reliable emergency shutdown mechanisms ensures quick response in case of malfunction.
- Workspace Safety: Designated areas for shock machine operations should be secured and marked clearly to restrict access to authorized personnel only.
Implementing these safety protocols not only protects personnel but also enhances the reliability of the experiments being conducted. Furthermore, maintaining a culture of safety improves overall operational efficiency.
Calibration and Maintenance
Calibration and maintenance are vital for the accurate performance of shock machines. Inaccurate calibration can lead to erroneous results and potentially hazardous situations. Regular maintenance ensures that the machines remain in optimal working condition. Essential practices include:
- Routine Calibration: Schedule regular checks to ensure that the machine settings match predefined standards. This correction process can significantly reduce errors in experimental data.
- Component Inspection: Regularly inspect all machine components for wear and tear. Parts such as sensors and pressure gauges should be thoroughly checked and replaced when necessary.
- Documentation: Keep detailed records of all maintenance activities. This practice aids in observing patterns in equipment performance and identifying areas that may need improvement.
- Software Updates: With advances in technology, ensure that any operating software is kept up to date to enhance functionality and safety features.
Recent Advancements in Shock Machine Technology
Recent advancements in shock machine technology signify a crucial development in the field. These innovations bring forth enhancements that not only improve the efficiency and effectiveness of the machines but also broaden their applications. The importance of these advancements cannot be overstated, as they play a pivotal role in refining experimental methods, optimizing performance, and developing new materials.
Understanding these advancements involves examining two main elements: innovative materials and integration with digital technology. Both aspects are essential in elevating the capabilities of shock machines and ensuring their relevance in modern scientific research and various industries.
Innovative Materials
The introduction of innovative materials marks a significant leap in shock machine technology. Materials such as carbon fiber composites and advanced polymers provide superior strength-to-weight ratios. This not only reduces the overall mass of the machines but also increases their durability.
- Lightweight Design: By utilizing lightweight materials, manufacturers can create shock machines that are easier to transport and operate without compromising structural integrity.
- Increased Resilience: Advanced materials often exhibit better resistance to impact and fatigue. This is especially important in scenarios involving repeated use, where conventional materials may fail under stress.
- Temperature Resistance: Some modern materials are designed to withstand extreme temperatures. This capability expands the operational range of shock machines in various research environments.
These improvements ensure that shock machines can perform reliably in challenging conditions, which is paramount for precise experimental outcomes.
Integration with Digital Technology
The integration of digital technology into shock machines presents a transformative approach to their operation and analysis. With the advent of smart sensors and data analytics, researchers can now gather real-time information during tests.
- Precision Control: Digital interfaces allow for finer control over the shocks produced. This level of precision leads to more accurate experiments with repeatable outcomes.
- Data Collection: Advanced sensors monitor variables such as pressure, temperature, and timing. This data can be analyzed to improve designs and understand material behaviors under shock conditions.
- Compatibility with Simulation Software: Digital technology often works hand-in-hand with simulation programs that model shock behavior. This synergy enables better pre-test predictions, enhancing the design process before actual experimentation takes place.
These advancements ensure that shock machines remain at the forefront of technological development, providing researchers with the tools necessary to push scientific boundaries.
These advancements ensure that shock machines remain at the forefront of technological development, providing researchers with the tools necessary to push scientific boundaries.
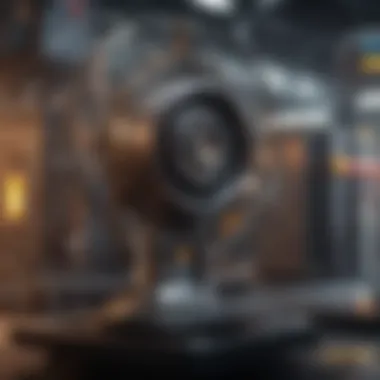
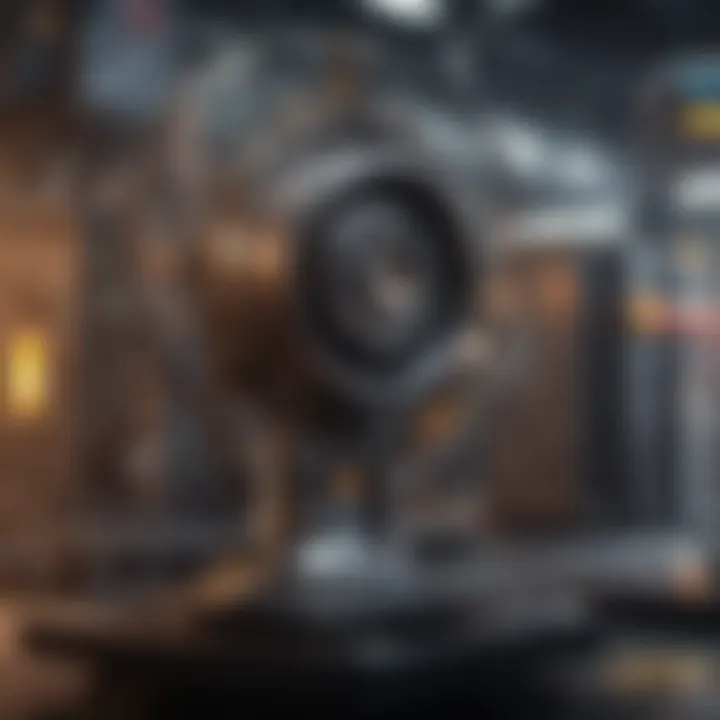
The ongoing evolution in shock machine technology, driven by innovative materials and the integration of digital technology, emphasizes a promising future. Not only are these machines becoming more efficient, but they are also paving the way for breakthroughs in various fields, driving research further into unknown territories.
Challenges and Limitations
Understanding the challenges and limitations of shock machines is crucial in appreciating their capabilities and implementation. It informs users about the boundaries of their current technology and identifies areas for potential improvement. Addressing these barriers not only aids in refining existing shock machine models but also guides future research endeavors. In academic and industrial settings, recognition of these limits ensures better risk management and operational efficiency.
Technical Barriers
Technical barriers often stand as obstacles in advancing shock machine technology. These barriers can stem from several sources, including material inadequacies, precision limitations, and the complexity of necessary calibrations. For instance, materials used must withstand extreme conditions without degrading over time. This aspect is often challenging because finding balanced performance between durability and efficiency is not straightforward. Additionally, the intricacies involved in calibrating shock machines to conform to specific experimental parameters can hinder their operation.
Another issue lies in the simulation and modeling of shock waves. Accurately predicting shock wave behavior in real-time conditions necessitates sophisticated computational techniques and software solutions. Regular errors in computation may cause discrepancies that can severely affect experimental results, leading to wasted resources and time.
Cost Implications
Cost implications present another significant challenge when dealing with shock machines. The initial investment required for high-quality shock machines can be extensive. This expense is often accompanied by maintenance and operational costs, which can deter institutions from adopting these technologies, particularly in budget-constrained environments.
Furthermore, developing innovative materials and advanced technologies frequently require additional funding, which many organizations may not prioritize. This financial constraint can limit the pace of progress and restrict new entries into the field, as only those with sufficient backing can afford to invest in cutting-edge shock machine technology.
As a result, many potential users may opt for existing models that may not meet their specific needs, thereby stifling innovation and limiting the capabilities of shock machines in various applications.
"Recognizing both technical barriers and cost implications enables researchers and engineers to make informed decisions on the utilization of shock machines in their work."
"Recognizing both technical barriers and cost implications enables researchers and engineers to make informed decisions on the utilization of shock machines in their work."
By tackling these challenges head-on, the scientific community can push forward, ensuring that shock machines fulfill their potential in experimentation.
Overall, the interaction between technical barriers and cost implications highlights a need for collaborative efforts, research, and innovation aimed at overcoming these hurdles. Such collaboration may lead to breakthroughs that would benefit various fields from material science to medical applications.
Future Directions in Shock Machine Research
The field of shock machine research is evolving, influenced by technological advancements and increased understanding of underlying physics. It is critical for researchers, engineers, and educators to stay informed about these future directions. This section will explore emerging trends and significant collaborative efforts aimed at enhancing the capabilities and applications of shock machines.
Emerging Trends
Recent developments in shock machine technology underscore the importance of innovation. Among these trends, we can observe:
- Integration of Advanced Materials: The use of high-performance materials like carbon composites and alloys can improve the durability and efficiency of shock machines.
- Automation and Control Systems: Enhanced automation enables operators to manipulate shock wave parameters with precision. It increases reproducibility in experiments and reduces human error.
- Data Analytics and Machine Learning: With the rise of big data, the incorporation of analytic tools allows for better insights into performance metrics and operational efficiency.
These emerging trends facilitate the design of shock machines that are not only more effective but also more accessible for diverse scientific applications.
Potential Collaborative Efforts
Collaboration among academic institutions, industries, and research facilities is vital for pushing the boundaries of shock machine technology. Key aspects of potential collaborations include:
- Interdisciplinary Research Initiatives: Engaging experts from physics, engineering, materials science, and computer science can yield significant breakthroughs. Each discipline contributes unique insights that can enhance overall machine functionality.
- Partnerships with Industry: Collaborating with manufacturers allows for the prototyping of cutting-edge designs, incorporating practical feedback into research findings. This connection bridges the gap between theory and application.
- Shared Platforms for Experimentation: Establishing platforms where researchers can share data and findings fosters a collaborative environment. Such platforms enhance innovation by combining resources and expertise.
"Collaborative efforts will drive the next generation of shock machines, transforming theoretical principles into real-world applications, ready for various scientific fields."
"Collaborative efforts will drive the next generation of shock machines, transforming theoretical principles into real-world applications, ready for various scientific fields."
Epilogue
The conclusion serves as a critical synthesis of the ideas presented in this article, rounding up the crucial findings and insights on shock machines. This section does not merely restate points made earlier, but it assesses their broader implications in scientific inquiry and engineering practices.
Summarizing Key Findings
Throughout the article, several key findings emerge that underline the significance of shock machines in various fields. Firstly, the foundational principles of shock machines, rooted in physics, enable a versatile application spectrum—from experimental physics to medical device development. Their ability to generate shock waves has proven essential in material testing and applications that necessitate precision and reliability. Moreover, recent advancements in materials and technology, particularly the integration of smart systems, transform the operational efficacy of these machines. This clearly indicates their growing relevance in contemporary research and industrial settings.
"The ability of shock machines to adapt and evolve alongside advancements make them an indispensable tool for researchers and engineers."
"The ability of shock machines to adapt and evolve alongside advancements make them an indispensable tool for researchers and engineers."
Implications for Future Research
Looking forward, the implications for future research in the field of shock machines are promising. As technology continues to progress, the potential for collaboration across disciplines widens. Future research can explore areas such as:
- Development of new materials that enhance shock wave generation efficiency.
- Enhancements in predictive algorithms for better calibration and maintenance, ensuring optimal performance.
- Investigation into sustainable practices and energy sources in driving shock machine operations.
Such avenues not only point towards improving the existing models but may potentially unlock new applications previously deemed unattainable. Therefore, ongoing exploration in this dynamic field remains vital for fueling innovation and addressing challenges.
In summary, the conclusion encapsulates the critical developments in shock machine technology and outlines pertinent future research directions. This not only underscores the machines' current impact but also propels interest towards their evolving role in science and engineering.