Exploring Tubulin KDa: Structure and Function
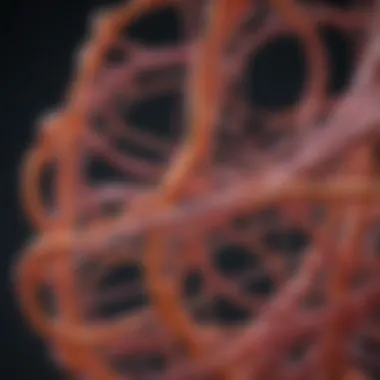
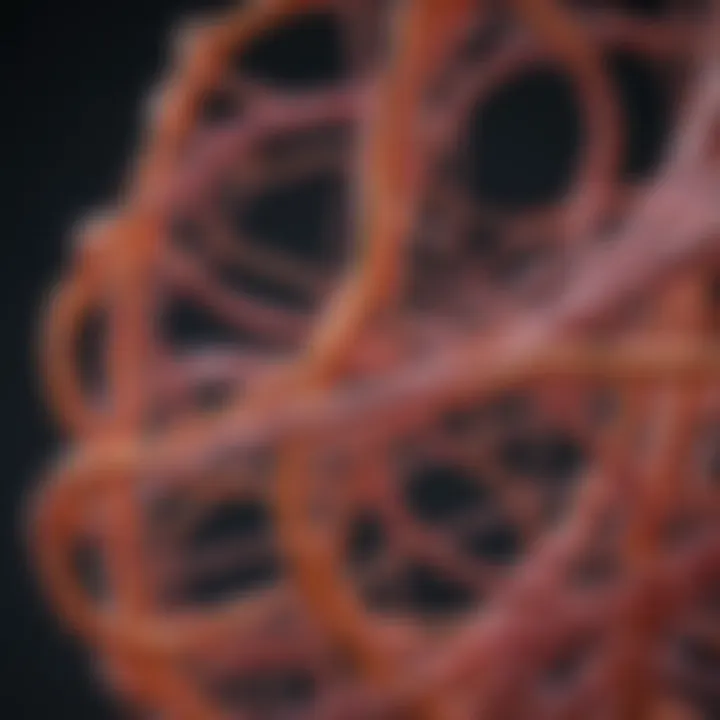
Intro
Tubulin, a highly versatile protein, plays a central role in the cellular architecture of eukaryotic organisms. It forms microtubules, which are crucial for numerous cellular functions, including maintaining cell shape, enabling intracellular transport, and facilitating cell division. The significance of tubulin extends beyond mere structural support; its dynamic nature allows it to interact with various cellular components, playing a vital role in signaling pathways and cell motility.
Understanding tubulin's molecular weight, specifically its representation in kilodaltons (KDa), can shed light on its structural characteristics and functional implications. By diving into the intricacies of tubulin, one can appreciate how this seemingly simple protein has profound effects on cellular health and disease.
In this article, we will explore the key concepts surrounding tubulin—its structure, function, and the latest research trends—providing a comprehensive view of its relevance in biological systems.
Preamble to Tubulin
Tubulin plays a central role in the biological machinery that drives a multitude of cellular functions. Understanding its structure and performance is not just an academic exercise but a critical endeavor that elucidates the fundamental workings of life at the cellular level. By examining tubulin, we grasp how microtubules form the framework of the cytoskeleton, influencing cellular shape, division, and intracellular transport.
In this article, we’ll navigate through the molecular intricacies and historical relevance of tubulin. Such knowledge is key for students, researchers, educators, and professionals alike, all of whom stand to benefit from a deeper insight into this essential protein. Tubulin is also pivotal in various health and disease contexts, thus making its study all the more relevant in today’s scientific landscape.
Definition and Basics
Tubulin is a globular protein that is instrumental in forming microtubules, which are part of the cytoskeleton within eukaryotic cells. It exists primarily in two forms: alpha and beta tubulin. These proteins can dimerize to form a heterodimer, the basic building block of microtubules. The precise molecular weight of tubulin is expressed in kilodaltons, with the alpha and beta tubulin each having a distinctive molecular weight around 55 kDa. This property contributes to its functionality in various cellular processes such as structural support, intracellular transport, and cell signaling.
Microtubules, formed from tubulin dimers, function actively in maintaining cellular organization. They assist with mitosis, intracellular transport, and even play a role in cell signaling pathways. Tubulin is, therefore, a cornerstone of cell biology, influencing processes that allow cells to perform their roles in organismal development and regulation.
Historical Context
The journey of tubulin discovery is interwoven with advances in cell biology and biochemistry. The term "tubulin" was first coined in the late 20th century when researchers began to map out its functions. It stemmed from early studies that described the filamentous structures in cells. Practitioners who employed electron microscopy in the 1970s were among the first to visualize microtubules, transforming our understanding of the cytoskeleton.
Scientists like Albert Kirschner and Timothy Mitchison in the 1980s made significant advancements by characterizing tubulin’s dynamics, leading to the concept of “dynamic instability,” where microtubules alternate between growth and shrinkage. This revolutionary idea not only painted a more vivid picture of cellular mechanics but also paved the way for further explorations into how tubulin contributes to processes such as cell division and intracellular transport.
Understanding this history reveals not merely how tubulin was discovered but the way it continues to influence ongoing research and therapeutic strategies today. In this context, the significance of tubulin transcends its basic definition, emerging as a focal point for therapeutic innovations.
Molecular Weight and Its Significance
Molecular weight serves as a fundamental concept in biochemistry and molecular biology, especially when discussing proteins like tubulin. For tubulin, which is integral to forming the cytoskeleton in eukaryotic cells, understanding its molecular weight in kilodaltons is more than an academic exercise; it is essential for comprehending its role in various cellular processes. The molecular weight not only affects its stability and interactions with other proteins but also impacts how it contributes to overall cellular mechanics.
The significance of molecular weight can be summarized as follows:
- Functional Roles: The molecular weight indicates the size and structure of tubulin, contributing to its diverse functionalities.
- Biological Interactions: It determines how tubulin interacts with other molecules within the cell, including microtubule-associated proteins and motor proteins such as kinesins and dyneins.
- Therapeutic Implications: Many drugs targeting cancer exploit tubulin’s response to its molecular weight. The difference in weight can shed light on resistance mechanisms against such drugs.
Understanding the molecular weight, therefore, provides insights not just into tubulin itself but also the broader implications for health and disease, making it a crucial topic within this discourse.
Understanding Kilodaltons
Kilodaltons (KDa) is a unit of measurement used to quantify the molecular weight of proteins. One kilodalton is equivalent to 1,000 daltons, where a dalton is a standard unit for expressing atomic and molecular weights. For tubulin, the molecular weight is generally around 50 KDa for each monomer, leading to a total of approximately 100 KDa in its dimer form.
This measurement tells us a great deal about the properties and behavior of tubulin within the cellular environment. It is not just a number; it informs us about:
- Complex Assembly: The dimerization of alpha and beta tubulin is essential in forming the long tubes called microtubules. Understanding its weight allows researchers to predict how these structures will behave under different conditions.
- Interactions and Binding: A protein's molecular weight can impact its binding affinities with other proteins. In the case of tubulin, different kilodaltons interact in a specific manner that facilitates its dynamic behavior during cellular processes.
Comparison with Other Proteins
When comparing tubulin's molecular weight with that of other proteins, its size is relatively moderate within the vast landscape of cellular proteins. This comparative context shines a light on tubulin’s unique role:
- Actin: For instance, actin, another vital cytoskeletal protein, also weighs around 42 KDa per monomer, which emphasizes the importance of size in cellular structure and function. The slight difference in weights can influence how these proteins assemble and interact within cells.
- Enzymes and Antibodies: Many enzymes like lysozyme weigh about 14 KDa, while larger proteins such as immunoglobulins can weigh between 150 KDa to 900 KDa. This variance in size shows how molecular weight can dictate the complexity and functionality of proteins in biological systems.
Structure of Tubulin
The structure of tubulin is paramount to understanding its myriad of functions within cells. It is not merely an assembly of atoms; it represents a well-orchestrated architecture that plays countless roles in cellular operations. Tubulin serves as the foundational building block for microtubules, which are essential for maintaining cell shape, enabling cellular movements, and facilitating intracellular transport.
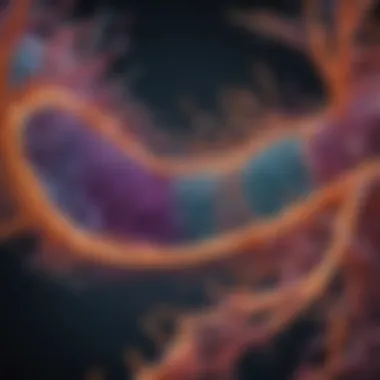
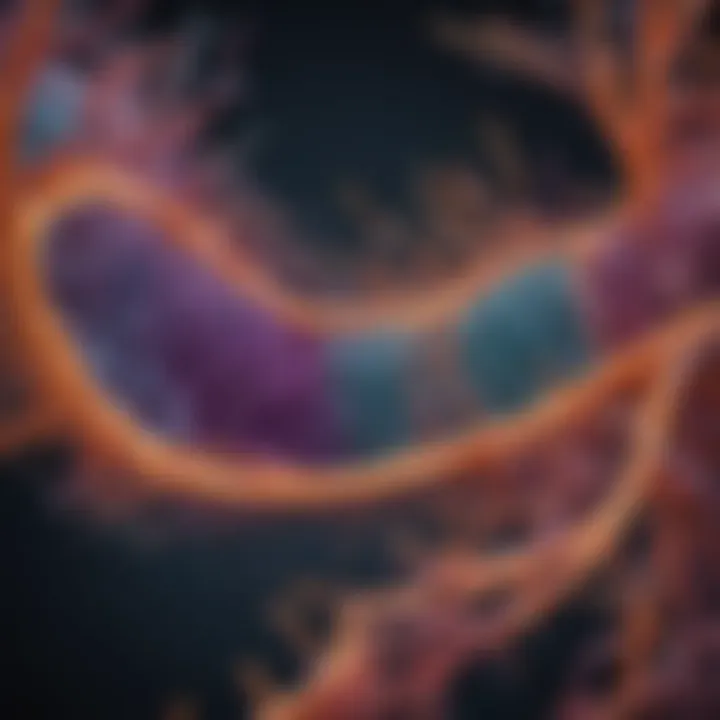
Alpha and Beta Tubulin
At the core of tubulin's structure are two tightly bound subunits named alpha and beta tubulin. These proteins exhibit a certain degree of symmetry as they come together to form a heterodimer, which is quite significant. Each subunit has a molecular weight, approximately 50 kDa for alpha and 55 kDa for beta, and they possess distinct yet complementary roles in microtubule dynamics.
- Alpha Tubulin: Characterized by its N-terminal domain, alpha tubulin binds to GTP but does not hydrolyze it. This stability is crucial, as it helps to anchor the microtubule at one end.
- Beta Tubulin: In contrast, beta tubulin binds GTP as well, but it is able to hydrolyze it to GDP. This hydrolysis plays a crucial part in the dynamic instability of microtubules, fueling their capacity for rapid growth and shrinkage.
These two types of tubulin not only dictate the physical properties of microtubules but also govern their functional dynamics. Their rapid assembly and disassembly, depending on cellular needs, reflect the adaptability required for cellular life.
Dimer Formation
The formation of tubulin dimers is a key point of interest. When an alpha-tubulin and a beta-tubulin come together, they exhibit a non-covalent docking behavior, resulting in a stable dimer. This interaction occurs rapidly, contributing to the dynamic properties that microtubules exhibit. Moreover, the ability of these dimers to polymerize into long filaments is central to maintaining microtubule integrity.
This dimerization is not just a trivial aspect; it's a dance of molecular forces at play. The exposed beta-tubulin offers a site for additional dimers to attach, allowing for the elongation of microtubules. The end result is a structure that can grow or contract, depending on cellular requirements.
Polarity and Treadmilling
Microtubules inherently possess polarity, which is crucial for their functions within the cell. Each microtubule has a designated plus end and a minus end, correlating to the orientation of the tubulin dimers within the filament. The plus end is generally where growth occurs, whereas the minus end tends to be more stable. This polarity is not arbitrary—it determines the directionality of motor proteins that travel along microtubules, facilitating critical processes such as transport, signaling, and even cell division.
The phenomenon of treadmilling characterizes the dynamic instability of microtubules. Under certain conditions, tubulin dimers add to the plus end while disassembling at the minus end. This process is vital for cellular activities like mitosis, where the microtubules must quickly reorganize to form the mitotic spindle.
"Treadmilling allows microtubules to maintain a constant length while still being in a state of flux, reflecting the cell's immediate needs."
"Treadmilling allows microtubules to maintain a constant length while still being in a state of flux, reflecting the cell's immediate needs."
In essence, the structure of tubulin, from the dimer formation to the concept of treadmilling, lays the groundwork for a multitude of cellular processes. Understanding these elements gives insight into finer details of how cells operate, adapt, and thrive.
Functional Roles of Tubulin
Tubulin plays a crucial role in numerous cellular processes, chiefly through its participation in microtubule formation. This section of the article discusses the essential functional roles of tubulin, shedding light on its significance for cellular structure and dynamics. By understanding these roles, we can appreciate how tubulin not only contributes to the basic framework of eukaryotic cells but also supports a diverse range of physiological processes that are pivotal for cell survival and function.
Microtubule Formation
Microtubules are dynamic structures composed predominantly of tubulin dimers, which consist of alpha and beta tubulin. The assembly of these dimers into microtubules involves a series of complex steps, starting from nucleation to elongation. This process is crucial because microtubules provide the scaffolding that helps maintain cell shape, facilitates intracellular transport, and establishes cell polarity.
- Nucleation: The initial formation of a microtubule occurs through nucleation, which is thought to require specialized proteins known as nucleating factors. These factors help in stabilizing the initial dimers to form small oligomers, laying the groundwork for further elongation.
- Elongation: Following nucleation, if conditions favor the addition of dimers, the microtubule begins to elongate. GTP-bound tubulin dimers add onto the growing end. As more dimers are added, the microtubule can take on various structural configurations, reflecting the dynamic nature of tubulin interactions.
- Dynamic Instability: This term captures the unique feature of microtubules where they can rapidly grow and shrink. It is a fundamental aspect of how cells adjust their structure and organization in response to shifting functional demands.
"It's this dynamic nature of tubulin that allows cells to remain adaptable in face of constant inner and outer changes."
"It's this dynamic nature of tubulin that allows cells to remain adaptable in face of constant inner and outer changes."
Understanding microtubule dynamics is not just academic. This knowledge has profound implications for fields like cancer research, where aberrations in microtubule formation can contribute to uncontrolled cell proliferation.
Cell Division and Mitosis
The role of tubulin in cell division is perhaps one of its most critical functional aspects. During mitosis, microtubules form the mitotic spindle, a structure essential for segregating chromosomes into the daughter cells. This process can be broken down into several stages:
- Prophase: The microtubules start to reorganize to form the spindle apparatus.
- Metaphase: Tubulin dynamics are paramount as the chromosomes align at the cell's equatorial plane, secured by the spindle fibers.
- Anaphase: Here, the microtubules shorten, pulling sister chromatids apart towards opposite poles of the cell.
- Telophase: The microtubules disassemble, allowing the nuclear envelope to reform.
Flaws in this tubulin-mediated process can lead to aneuploidy, which is commonly observed in various forms of cancer. Therefore, studying how tubulin orchestrates these mitotic events is vital in understanding not only normal cell function but also the mechanics behind tumorigenesis.
Intracellular Transport
Tubulin, through its reinforcing role in microtubule structures, is influential in enabling intracellular transport. Motor proteins, such as kinesins and dyneins, utilize microtubules as tracks to transport cellular components, including organelles and vesicles. This transport system can be elaborated as follows:
- Kinesins: Move cargo toward the plus end of the microtubule, which typically points towards the cell membrane. They are instrumental in exocytosis and in delivering neurotransmitters to synaptic terminals.
- Dyneins: Operate in the opposite direction, hauling materials toward the minus end of microtubules, usually toward the cell nucleus. They play a critical role in retrograde transport which is essential for recycling components within the cell.
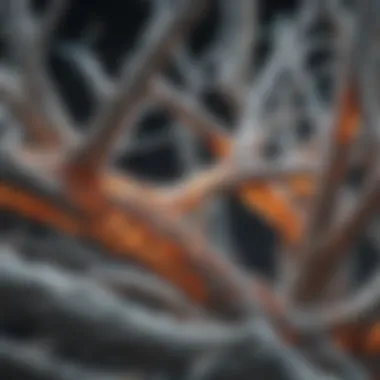
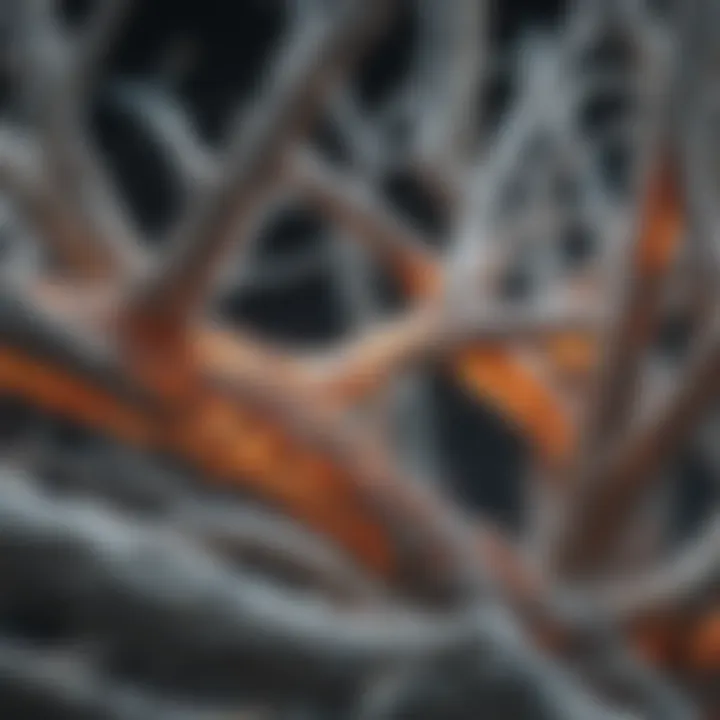
Thus, tubulin not only maintains the structural integrity of cells but is also a conveyor of vital materials that sustain cellular operations. An understanding of these transport mechanisms can have ramifications for research into neurodegenerative diseases, where such transport processes are often impaired.
Regulation of Tubulin Dynamics
The regulation of tubulin dynamics is one of the key themes in understanding the intricate roles that tubulin and microtubules play in cellular processes. The ability for tubulin to assemble and disassemble rapidly is crucial for maintaining cellular integrity and facilitating numerous biological functions. Without precise control over these dynamics, cells would struggle to perform essential tasks like division, movement, and structural support.
The delicate balance in tubulin dynamics reflects a fine-tuned system that allows cells to adapt to their environments, respond to signals, and coordinate activities with other cellular components. Factors that influence tubulin dynamics can be enzymes like GTPases, or even the concentration of tubulin itself, which can shift depending on cellular needs.
Post-Translational Modifications
Post-translational modifications (PTMs) of tubulin add yet another layer of complexity to its regulation. These modifications occur after the synthesis of the protein and can significantly alter its function and stability. For example, the addition of acetyl groups to tubulin can enhance the stability of microtubules, making them less prone to disassembly. This is particularly relevant in neurons, where stable microtubules are crucial for maintaining axonal structure.
Some common PTMs of tubulin include:
- Acetylation: Increases stability and affects interactions with other proteins.
- Tyrosination: A reversible modification that impacts microtubule dynamics.
- Glutamylation: Possibly involved in cellular signaling and protein interactions.
Each of these modifications can be dynamically regulated, influencing the behavior of tubulin in response to specific cellular cues. Keeping track of these changes provides valuable insights into how cells manage their internal landscapes.
Binding Proteins and Factors
Additionally, binding proteins and factors are critical for regulating tubulin dynamics. These proteins can stabilize or destabilize microtubules through interactions that affect their polymerization rates. For instance, proteins like tau stabilize microtubules in neurons, promoting longevity and stability against depolymerization. Conversely, proteins such as kinesin and dynein aid in the transport of cellular cargo along microtubules, which is essential for functions ranging from signaling to nutrient distribution.
The interplay between tubulin and its associated proteins can be likened to a well-choreographed dance, where each participant has a distinct role that contributes to maintaining order and efficiency within the cellular environment. Disruptions in this delicate balance, whether from genetic mutations or external factors, can lead to diseases, particularly neurodegenerative conditions.
"The regulation of tubulin dynamics showcases the complexity of cellular machinery and highlights how minor changes at the molecular level can have broad implications for cellular health."
"The regulation of tubulin dynamics showcases the complexity of cellular machinery and highlights how minor changes at the molecular level can have broad implications for cellular health."
Ultimately, understanding how these regulations function paves the way for innovative therapeutic strategies. By targeting specific PTMs or binding proteins, researchers may develop treatments to correct dysfunctional tubulin dynamics that contribute to various diseases.
Implications in Health and Disease
The relationship between tubulin's functionality and its implications in health and disease is vast. This is not just a matter of basic biology; understanding tubulin's roles can lead to breakthroughs in treatment and prevention strategies for a range of ailments. It becomes clear that tubulin is not merely a structural component; it has critical influences on numerous cellular processes.
Cancer and Tubulin
Cancer treatment has often embraced the notion of targeting rapidly dividing cells. Tubulin's importance in cell division positions it as a prime target in oncology. Drugs that inhibit tubulin polymerization—such as paclitaxel and vincristine—exploit the delicate balance of microtubule dynamics. This interference can effectively halt the growth of tumors by preventing the proper segregation of chromosomes during cell division. However, it's not merely about halting division; the complexity arises from the fact that cancer cells can develop resistance to these therapies. Some tumor cells bypass the drug's effects by modifying their tubulin structure or utilizing alternative pathways to maintain microtubule function. This has led to an ever-growing research landscape looking to innovate on existing therapies or discover novel compounds that could outsmart these adaptive mechanisms.
"To combat cancer’s relentless drive, researchers are delving deeper into the molecular undercurrents of tubulin dynamics."
"To combat cancer’s relentless drive, researchers are delving deeper into the molecular undercurrents of tubulin dynamics."
Notably, recent studies have indicated that certain mutations in tubulin can lead to an active role in promoting tumorigenesis. These mutations do not only affect the structure; they can alter microtubule dynamics, shifting the balance in favor of cancer cell proliferation. As such, advancements in understanding the genetic makeup of tubulin can potentially pave the way for personalized medicine approaches in cancer treatment.
Neurodegenerative Disorders
Neurodegenerative diseases such as Alzheimer’s, Parkinson’s, and Huntington’s represent a significant challenge, with tubulin’s role in cellular integrity and neuron function coming under the spotlight. Microtubules are vital for transporting materials within neurons, ensuring that essential proteins and organelles reach their destinations.
Deficiencies in tubulin stability can lead to catastrophic consequences for neurons. For instance, tau proteins, which are closely related to tubulin, can become hyperphosphorylated, leading to the formation of neurofibrillary tangles—a hallmark of Alzheimer’s disease. These disruptions impede regular transport functions, resulting in neuronal death over time.
Research into tubulin's role in neurodegeneration emphasizes
- Proactive measures for maintaining tubulin integrity
- Molecular therapies targeting tubulin alterations
- Understanding the connection between tubulin dynamics and amyloid-beta accumulation
Identifying therapeutic interventions that stabilize microtubules or correct their assembly has become a focus area. This research may ultimately thrive in providing new avenues to slow or rectify the detriment inflicted by neurodegenerative disorders.
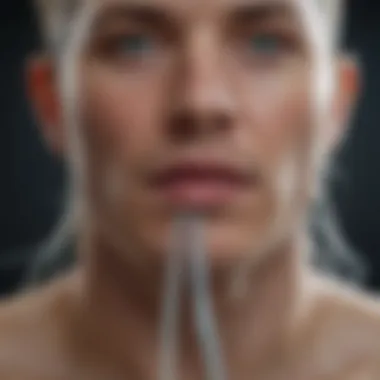
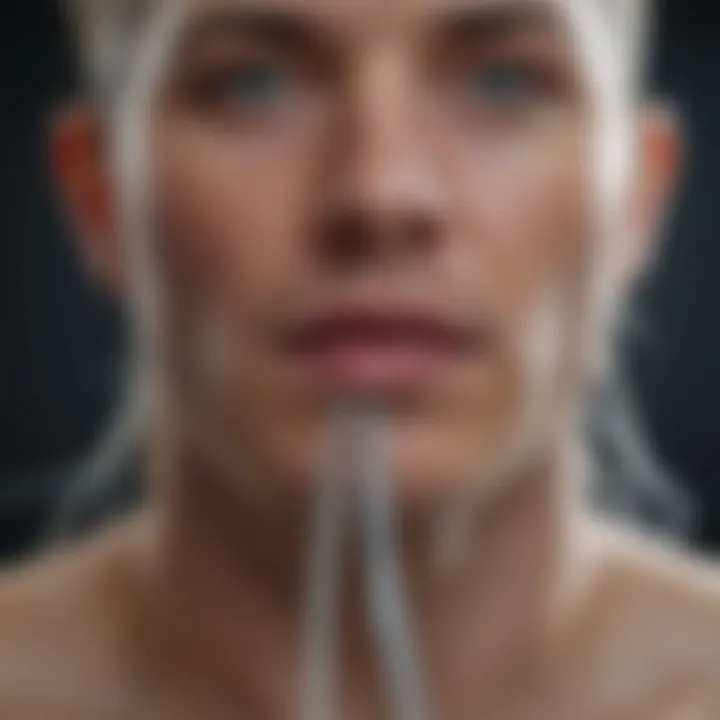
As we progress, it is imperative to recognize the intertwined nature of tubulin dynamics, health outcomes, and disease mechanisms. The future of research in this area looks promising, with potential to address significant health challenges.
Research Methods for Studying Tubulin
Researching tubulin, a cornerstone of cellular architecture, is vital for several scientific fields, particularly in biology and medicine. Understanding the mechanisms of tubulin dynamics and its interactions is not just academic; it has implications in diseases like cancer and neurodegenerative disorders. Different methodologies have been developed over the years to precisely study tubulin's structure and function.
Biochemical Techniques
Biochemical methods provide insights into tubulin's properties at a molecular level. These techniques can isolate tubulin from cells and study its behavior under various conditions. Some prominent biochemical approaches include:
- Protein Purification: Techniques such as affinity chromatography allow researchers to isolate tubulin from cell lysates. This purification is crucial for both functional studies and structural analysis.
- Binding Assays: Investigators can use these assays to understand how tubulin interacts with other proteins. By using labeled tubulin, they can measure binding affinities and kinetics, providing insights into the regulatory mechanisms that govern tubulin function.
- Enzymatic Assays: Certain enzymes alter the state of tubulin, affecting its polymerization behavior. Studying these enzymatic reactions helps clarify how post-translational modifications influence tubulin dynamics and stability.
These techniques collectively enhance our understanding of tubulin, uncovering its multifaceted roles in cellular processes. However, careful considerations must be taken into account, such as potential artifacts during purification processes and the biological relevance of the in vitro conditions selected for experimentation.
Imaging Techniques
Visualization is another critical area in tubulin research, employing sophisticated imaging methods to explore tubulin's structural organization within cells. Some techniques commonly used include:
- Fluorescence Microscopy: By tagging tubulin with fluorescent markers, scientists can observe microtubule behavior in live cells. This method enables real-time imaging of microtubule dynamics, providing pivotal insights into cellular processes like mitosis and motility.
- Electron Microscopy: This technique offers high-resolution images, allowing detailed studies of tubulin structures. It can reveal the arrangement of tubulin subunits in microtubules and how they contribute to the overall architecture of the cytoskeleton.
- Super-Resolution Microscopy: Advanced forms of microscopy, such as STORM (Stochastic Optical Reconstruction Microscopy), break the diffraction limit of light, enabling researchers to visualize tubulin structures at a nanometer scale. This is particularly useful for understanding the fine details of microtubule assembly and disassembly.
The integration of these imaging techniques forms a crucial part of tubulin research, supporting the investigation of how tubulin behaves in physiological conditions.
Understanding the methods used to study tubulin is fundamental, as they shape our approach to addressing broader biological questions and developing effective therapies.
Understanding the methods used to study tubulin is fundamental, as they shape our approach to addressing broader biological questions and developing effective therapies.
In sum, combining biochemical and imaging techniques allows researchers to map out the complex life of tubulin, revealing its importance in cellular processes and developing targeted interventions in disease contexts.
Future Directions in Tubulin Research
As we venture deeper into the realm of cellular biology, the exploration of tubulin continues to be paramount. This importance is not just academic; it has real implications for health, disease treatment, and understanding cellular functions. Researchers are currently eyeing tubulin as a crucial player in several biological processes, and identifying its underlying dynamics could open doors to groundbreaking applications.
Novel Therapeutic Targets
One of the most promising avenues in tubulin research is uncovering novel therapeutic targets. Recent studies have delved into how manipulating tubulin dynamics can lead to significant clinical advancements, particularly in cancer treatment.
- Targeting Microtubule Dynamics: The capability of microtubules to undergo rapid assembly and disassembly makes them attractive targets in cancer therapy. By developing drugs that stabilize or destabilize these structures, researchers can inhibit the proliferation of cancer cells effectively. For instance, compounds that bind specifically to beta-tubulin or disrupt the normal process of treadmilling could alter cancer cell behavior drastically.
- Enhancing Drug Specificity: By identifying unique structural features of tubulin that differ between normal and cancerous cells, scientists hope to devise more selective drugs. Such advancements could lead to treatments with reduced side effects, preserving healthy tissues while targeting malignant cells more effectively.
- Customizing Treatments: There’s also a burgeoning interest in personalized medicine, where treatments are tailored based on individual genetic profiles. By understanding how tubulin functions can vary among individuals, tailored therapeutic strategies might be created to optimize outcomes.
Understanding Resistance Mechanisms
While progress in tubulin targeting therapies is encouraging, understanding resistance mechanisms is equally vital. When cancer cells adapt, they often develop resistance to existing therapies, which can pose significant challenges.
- Identifying Resilience Pathways: Research is currently exploring the pathways that cancer cells utilize to develop resistance. For instance, alterations in the expression of tubulin isotypes might confer survival advantages, allowing cells to withstand treatment. Understanding these changes is crucial for designing second-line therapies that can effectively bypass or negate resistance mechanisms.
- Integrative Approaches: It is becoming increasingly clear that a multi-faceted approach is essential. Combining tubulin-targeting agents with other therapies may limit the possibility of developing resistance. Strategies might include integrating chemotherapy with immunotherapy and identifying the synergistic effects of such combinations on tumor response.
- Characterizing Tumor Microenvironments: Another dimension is how the tumor microenvironment influences tubulin’s behavior. Investigating the interplay between extracellular factors and tubulin dynamics can provide insights into how cells communicate and adapt.
Understanding and overcoming resistance mechanisms is as critical as the initial creation of therapeutic agents.
Understanding and overcoming resistance mechanisms is as critical as the initial creation of therapeutic agents.
Closure
In concluding this exploration of tubulin and its molecular weight denoted in kilodaltons, it becomes essential to accentuate the intricacies of this protein’s role in cellular dynamics. Tubulin is not merely a structural protein; its significance ripples through various biological processes that underpin life itself. The insight garnered through this article paves the way for a greater appreciation of tubulin's contributions beyond its structural role, especially in areas such as cell division, intracellular transport, and response to diseases.
Key points arise that underline the importance of understanding tubulin:
- Molecular Weight and Functionality: The molecular weight of tubulin, expressed in KDa, is more than a technical specification. It often correlates with the protein's functionality and interaction with other cellular components, enhancing our understanding of its operational mechanics.
- Health Implications: Recognizing how tubulin dynamics contribute to disease mechanisms is crucial. This understanding might lead to novel therapeutic targets, particularly in oncology and neurodegenerative disorders, where tubulin's functions are disrupted.
- Research Outlook: The methods employed to study tubulin are evolving, providing profound insights into its behavior under various conditions. New techniques promise to illuminate aspects of tubulin not fully grasped until now, potentially unlocking doors to innovative treatments.
The culmination of this article defines tubulin as a central player in cellular architecture. It emphasizes that the implications of tubulin research stretch far and wide, underpinning both basic scientific inquiry and applied medical science. As researchers strive to unravel the complexities of tubulin dynamics, the ongoing quest for knowledge stands to benefit numerous fields, from molecular biology to clinical applications.
"The study of tubulin is not just an exercise in understanding a protein. It is a gateway into the heart of cellular function and a field rich with potential to unlock the secrets of human health and disease."
"The study of tubulin is not just an exercise in understanding a protein. It is a gateway into the heart of cellular function and a field rich with potential to unlock the secrets of human health and disease."
In summary, the journey through the varied facets of tubulin highlights its importance. Its molecular characteristics are not just academic trivia; they are fundamental to the biological narrative that shapes our understanding of life at the cellular level.