Fundamental Concepts and Advances in Analytical Chemistry
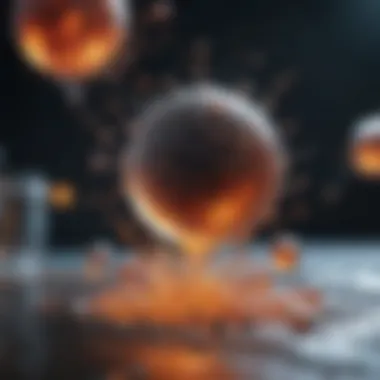
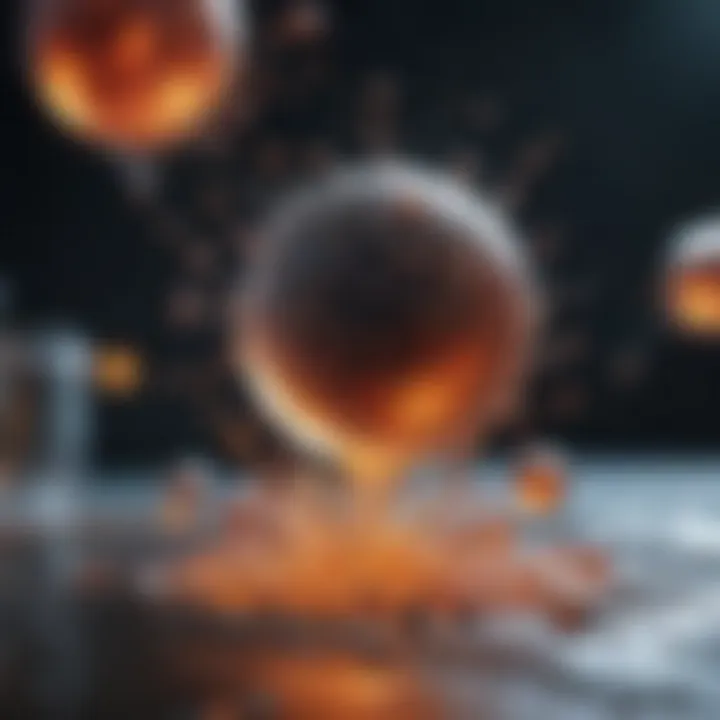
Intro
Analytical chemistry stands as a cornerstone in the broader landscape of scientific inquiry. It embodies a discipline that not only seeks to understand the composition of materials but also delves into how these materials interact within various contexts. The thrust of analytical chemistry is to provide robust methods that ensure accuracy, reproducibility, and reliability in measurements.
As we navigate through the core concepts and recent developments in this field, we unlock a trove of knowledge that can propel scientific advancement across numerous sectors—pharmaceuticals, environmental science, food safety, and more. Understanding analytical chemistry is akin to having a map in a complex maze; it guides researchers through the intricacies of substance analysis, offering insights that can spark innovation.
Key Concepts
Definition of the Main Idea
At its essence, analytical chemistry is the art and science of determining what substances are present in a sample and in what quantities. This involves a myriad of techniques ranging from traditional methods like titration to modern innovations such as mass spectrometry. The goal is not merely to identify substances but to characterize them in a manner that is insightful and meaningful.
Overview of Scientific Principles
A few fundamental principles underscore analytical chemistry:
- Separation: Before analysis can occur, components must often be isolated from a mixture. Techniques like chromatography facilitate this by exploiting variations in chemical properties.
- Identification: Once separated, it’s crucial to recognize which substances are present. Advanced technologies such as infrared spectroscopy use light interactions to yield spectral fingerprints that pinpoint molecular structures.
- Quantification: The final step involves quantifying the identified substances, ensuring that results reflect the true composition of the sample. Methods like gravimetry and titrimetry track changes meticulously to achieve accuracy.
"In a world increasingly driven by data, the techniques of analytical chemistry bridge the gap between raw information and meaningful insights."
"In a world increasingly driven by data, the techniques of analytical chemistry bridge the gap between raw information and meaningful insights."
Current Research Trends
Recent Studies and Findings
The landscape of analytical chemistry is ever-evolving, marked by innovations that redefine established conventions. Recent studies emphasize:
- The importance of miniaturization: Researchers are working on creating portable devices that can perform complex analyses in the field, cutting down on time and resources.
- Integration of computational methods: Advanced algorithms are being developed to analyze large datasets generated by modern instruments, helping to uncover patterns and correlations that might otherwise go unnoticed.
Significant Breakthroughs in the Field
Breaking new ground also means tackling challenges head-on. Notable recent breakthroughs include:
- Development of novel sensors: Innovations in sensor technology enhance detection limits and improve specificity, making it easier to spot trace amounts of contaminants in food or environmental samples.
- Advances in mass spectrometry: Enhancements in resolution and speed have made mass spectrometry a go-to method for complex mixtures, enabling more comprehensive analyses than ever before.
The unfolding narratives in analytical chemistry reveal a discipline that is not just about techniques but is interwoven with broader scientific progress. As one dives deeper into this compelling field, it becomes clear that the horizon is brimming with potential for discovery and innovation.
Prelims to Analytical Chemistry
Analytical chemistry stands as a pillar in the realm of science, bridging the gap between theory and practical applications. Understanding analytical chemistry is essential for anyone involved in research or any field that relies on the precise measurement of substances. It touches numerous sectors, including environmental science, pharmaceuticals, and clinical analysis, making it truly versatile.
Analyzing compounds and materials with exactness not only assists in quality control but also enables breakthroughs in various industries. Insights gained through analytical methods can lead to discoveries, guide regulatory compliance, and enhance product safety. In short, analytical chemistry is where the art of measurement meets the science of inquiry.
Historical Context
The historical development of analytical chemistry is rich and varied. Initially, analytical techniques began with simple methods like titration and gravimetry, which can trace their origins back to early alchemical practices. As time marched on, the industrial revolution prompted a need for more precise techniques. The introduction of instruments like the spectroscope in the 19th century marked a significant advancement.
During the 20th century, the drive for speed and accuracy led to the evolution of complex methodologies. For instance, the advent of chromatography revolutionized the way mixtures could be separated and analyzed. In essence, what started as rudimentary techniques has blossomed into an intricate field where modern technology intersects with analytical prowess.
Definition and Scope
Analytical chemistry can be broadly defined as the branch of chemistry that focuses on the qualitative and quantitative analysis of substances. This definition, while straightforward, encompasses a wide range of methodologies, which can be categorized into different approaches and techniques.
The scope of analytical chemistry is expansive, covering:
- Qualitative Analysis: Determining the presence of certain chemicals in a sample.
- Quantitative Analysis: Measuring the exact amount of a substance in a sample.
- Method Development: Creating new analytical methods to solve specific problems.
Modern analytical chemistry draws upon various techniques that can be tailored to meet the needs of different scientific questions, whether it’s elucidating the structure of an unknown compound or quantifying pollutants in the environment. Continuing advancements in technology, including automation and computer-assisted methods, are also reshaping this field, broadening its applicability and enhancing accuracy.
Key Techniques in Analytical Chemistry
The realm of analytical chemistry is ever-expanding, carving out pathways that not only facilitate exploration but also propel discoveries across various other scientific fields. At the heart of this discipline are key techniques crucial for the identification, quantification, and characterization of chemical substances. Each method offers unique advantages and is tailored to address particular analytical challenges. Understanding these methods paves the way for advancements in research and practical applications that stretch from pharmaceuticals to environmental monitoring, thus solidifying analytical chemistry's significance.
Spectroscopic Methods
Spectroscopic methods hold a prominent position among analytical techniques. They revolve around the interaction of light with matter to provide essential insights into the composition and structure of substances. Each method serves its own niche, whether it involves identifying molecular structures or determining concentration levels. Let's break down three notable spectroscopic approaches.
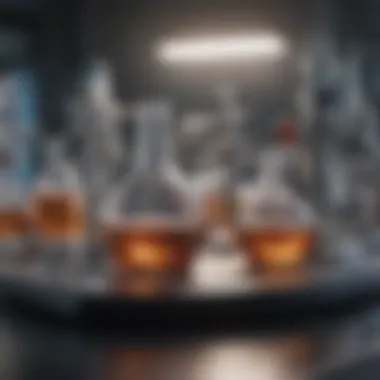
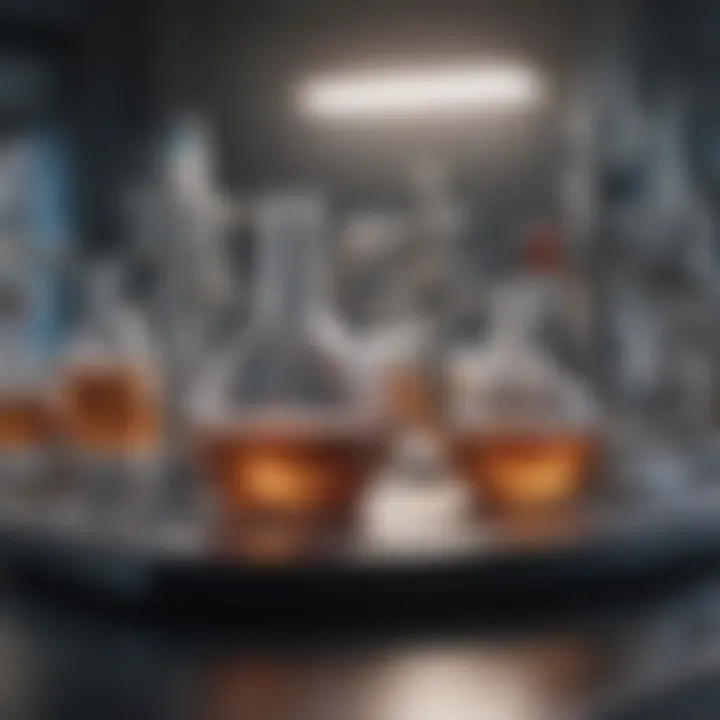
Ultraviolet-Visible Spectroscopy
Ultraviolet-Visible (UV-Vis) Spectroscopy is a powerful analytical technique characterized by its ability to assess the absorption of ultraviolet and visible light by a sample. One of its core contributions lies in its application for measuring the concentration of compounds in solution, utilizing Beer-Lambert Law principles. This method scores high on versatility and ease of use.
Key Characteristics:
- Speed and Simplicity: UV-Vis is relatively quick to administer and requires minimal sample preparation.
- Cost-Effective: Compared to other spectroscopic methods, it requires less expensive equipment.
A unique feature of UV-Vis is its ability to analyze color changes in chemical reactions. Its advantages are apparent in various fields, notably in pharmaceutical quality control. However, it also has limitations; it may struggle with low-concentration samples due to sensitivity issues and provides less structural information compared to other techniques.
Infrared Spectroscopy
Infrared (IR) Spectroscopy employs infrared light to scan a sample. It excels at identifying specific molecular bonds and functional groups within organic compounds, providing valuable information about molecular structure. This technique's mainstay is its ability to create spectra that serve as unique fingerprints for different substances.
Key Characteristic:
- Functional Group Identification: It effectively recognizes characteristic absorption bands corresponding to specific molecular vibrations, making it a go-to for organic chemists.
The unique capability of IR spectroscopy to unravel complex mixtures without extensive sample preparation enhances its application in areas like food chemistry and forensic science. Nonetheless, challenges exist, as it may require complementary techniques to obtain a full structural understanding, particularly for complex compounds.
Nuclear Magnetic Resonance (NMR)
Nuclear Magnetic Resonance (NMR) stands out as a sophisticated analytical technique that provides in-depth information about the structure of compounds through the behavior of nuclei in a magnetic field. Its profound contribution to analytical chemistry resides in elucidating molecular structure and dynamics, making it indispensable in organic and inorganic chemistry.
Key Characteristic:
- Structural Insight: NMR is unmatched in its ability to provide detailed information about molecular connectivity, including stereochemistry.
The unique advantage of NMR in revealing quantitative and qualitative details about a molecule is invaluable. However, the drawbacks include a high cost of equipment and the complexity of spectral interpretation, which can be a steep learning curve for newcomers.
Chromatography
Chromatography is another cornerstone in the toolkit of analytical chemistry, essential for separating mixtures into their individual components. Its significant role extends across various sectors, from chemical manufacturing to clinical diagnostics. By applying different stationary and mobile phases, it enables the purification and quantification of complex samples.
Gas Chromatography
Gas Chromatography (GC) is particularly suited for gas-phase samples and volatile compounds. It employs a carrier gas to transport the sample through a column packed with a stationary phase. The technique stands out for its separation efficiency and speed.
Key Characteristic:
- High Resolution: GC delivers sharp peaks, making it easier to identify and quantify components present in a sample.
A unique feature is its capacity to analyze trace impurities in substances, which is highly beneficial in pharmacokinetics and environmental monitoring. The primary disadvantage is its limited use for non-volatile compounds, restricting its applicability to a narrower range of samples.
Liquid Chromatography
Liquid Chromatography (LC) is utilized extensively, especially for compounds that are not easily vaporized. This technique separates components based on their interaction with the stationary phase while being carried by a liquid mobile phase.
Key Characteristic:
- Flexibility: LC can handle a wide array of chemical compounds across different industries.
A distinguishing feature of LC is its application in high-performance liquid chromatography (HPLC) to analyze pharmaceuticals and biochemical molecules efficiently. However, it often requires extensive training due to more intricate operational parameters and can be slower than GC.
Supercritical Fluid Chromatography
Supercritical Fluid Chromatography (SFC) combines features of both gas and liquid chromatography by using supercritical fluids as the mobile phase. This technique offers high efficiency and selectivity in separation processes.
Key Characteristic:
- Environmental Friendliness: SFC is seen as a greener alternative, often employing carbon dioxide as the solvent, which is less hazardous than traditional organic solvents.
Its unique feature is the ability to analyze compounds with greater solubility and lower viscosity than conventional methods. Despite its advantages, challenges in equipment cost and the need for specialized knowledge can limit its widespread adoption.
Electrochemical Analysis
Electrochemical analysis taps into the relationships between chemical substances and electrical energy, offering methods like potentiometry and voltammetry. These techniques provide critical insights, particularly in assessing the electrochemical properties of compounds. They are widely employed in environmental monitoring, food safety, and healthcare settings.
Mass Spectrometry
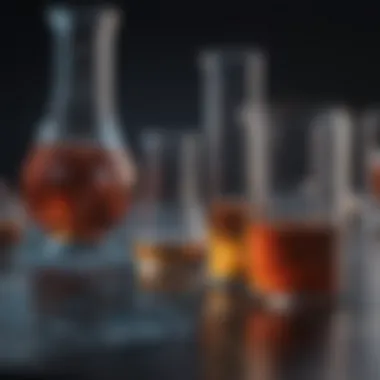
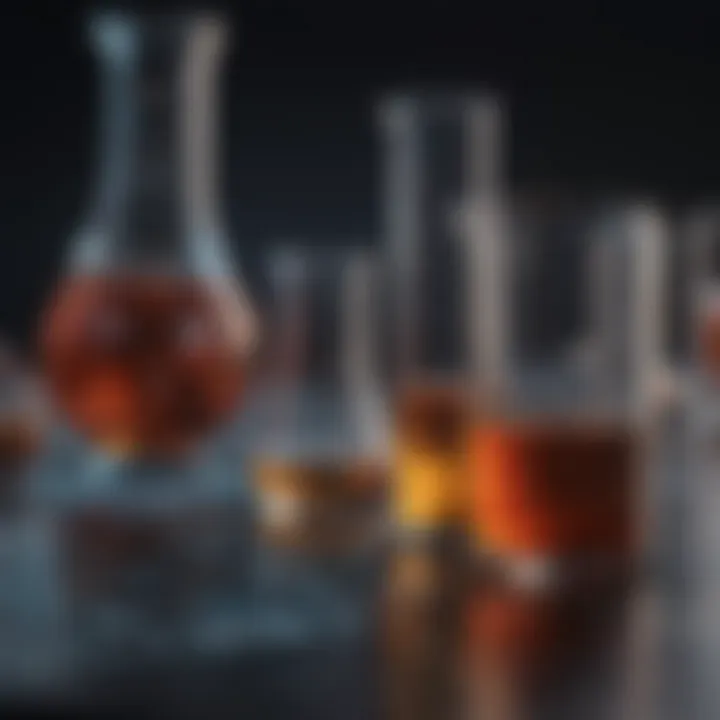
Finally, Mass Spectrometry (MS) operates on the principle of measuring the mass-to-charge ratio of ions, yielding information on molecular weight and structure. Its significance cannot be overstated—it's a fundamental tool in contemporary analytical chemistry, essential for the identification and quantification of biomolecules, environmental pollutants, and drugs, among others.
Ultimately, key techniques in analytical chemistry serve as critical instruments in the quest to unravel the complexities of matter. Each method brings something unique to the table, blending innovation with practicality to address the diverse range of challenges faced in the field. As the boundaries of analytical chemistry continue to expand, the importance of these techniques will only grow.
Data Analysis and Interpretation
Data analysis and interpretation form a cornerstone of analytical chemistry, blending experimental results with the theories and frameworks that govern those findings. This process is pivotal for transforming raw data into meaningful insights, guiding future research directions, and facilitating application in real-world scenarios. The ability to apply statistical methods and computational techniques enhances the accuracy of results and ensures that conclusions are both valid and relevant to the questions posed in any given study.
The importance of effective data analysis in analytical chemistry cannot be overstated. It not only validates experimental outcomes but also aids in the identification of patterns and anomalies. When a chemist analyzes data, they sift through vast quantities of information, ultimately drawing connections that may not be immediately obvious. By employing rigorous methods, researchers can ensure reproducibility and provide credible evidence to support their claims. This is fundamental, especially when findings are to be applied in critical fields such as clinical chemistry or environmental analysis.
In the ever-evolving landscape of analytical chemistry, the integration of sophisticated data analysis techniques is crucial. For instance, researchers now utilize machine learning algorithms to predict outcomes and enhance the efficiency of analyses. Additionally, big data technologies enable the handling of complex datasets, allowing scientists to glean insights that were once unimaginable.
"The future of analytical chemistry lies in smart data interpretation – where algorithms meet data-driven decision making."
"The future of analytical chemistry lies in smart data interpretation – where algorithms meet data-driven decision making."
Statistical Methods in Chemistry
Statistical methods play a central role in the analysis of experimental data in chemistry. These approaches are indispensable for ensuring that the conclusions drawn from experiments are statistically sound. In essence, they allow chemists to assess the reliability of their data, identify trends, and make predictions based on observed phenomena. Basic statistical concepts—such as mean, median, mode, variance, and standard deviation—provide foundational tools for evaluating data.
Beyond these fundamentals, more advanced statistical techniques are commonly employed. For instance:
- Regression Analysis: Used to model the relationship between dependent and independent variables, enabling the prediction of outcomes based on varying parameters.
- ANOVA (Analysis of Variance): Facilitates comparison of means across multiple groups, essential for determining the significance of experimental results in a multi-faceted dataset.
- Multivariate Statistics: Useful when multiple variables influence outcomes, allowing for a comprehensive analysis that considers interdependencies.
Employing these statistical methods ensures that data interpretation in analytical chemistry is not merely subjective but grounded in quantifiable observations. Their use also mitigates the risks associated with drawing erroneous conclusions, which can have serious ramifications, particularly in fields like pharmaceuticals or environmental evaluation.
Computational Chemistry
Computational chemistry emerges as a powerful ally in the realm of analytical chemistry, enabling chemists to answer complex questions through theoretical methodologies. With the advent of high-performance computing and sophisticated algorithms, researchers can simulate chemical processes, analyze molecular interactions, and predict reactivity without solely relying on experimental approaches.
One of the main advantages of computational chemistry is its ability to process a wealth of information much faster than traditional laboratory methods. This quick analysis can streamline the research process, allowing for more comprehensive studies at a fraction of the cost and time. Through modeling, chemists can visualize molecular structures, assess energetics, and evaluate potential outcomes before conducting physical experiments.
Furthermore, computational techniques like quantum chemistry and molecular dynamics simulations provide insights into reaction mechanisms and interactions at an atomic level. These capabilities not only illuminate the fundamental principles of chemical reactions but also highlight areas for innovative experimental designs, potentially paving the way for breakthroughs in areas like drug design or environmental remediation.
To sum up, the role of data analysis and interpretation in analytical chemistry is multi-faceted and crucial for the advancement of the field. By leveraging both statistical methods and computational approaches, chemists can enhance the accuracy and reliability of their results, ultimately driving significant contributions to a variety of scientific domains.
Applications of Analytical Chemistry
Analytical chemistry plays a pivotal role in various industrial, environmental, and clinical settings, shaping our understanding and manipulation of substances in a meticulous way. The significance of this discipline cannot be overstated, as it bridges scientific theory with practical applications. Let's explore specific applications and the impact they have across different fields.
Environmental Analysis
Environmental analysis concerns itself with evaluating pollutants and ensuring compliance with environmental regulations. The tools and techniques from analytical chemistry provide valuable insights into the quality of air, water, and soil. For instance, techniques like gas chromatography and mass spectrometry are paramount in detecting trace contaminants in environmental samples.
Without these methods, tracking harmful substances like persistent organic pollutants would be akin to finding a needle in a haystack. The precision offered by analytical chemistry aids in monitoring changes in climate and evaluating the effectiveness of remediation efforts.
"The modern imperative to protect our environment hinges on our ability to accurately measure its condition."
"The modern imperative to protect our environment hinges on our ability to accurately measure its condition."
Moreover, analytical chemistry underpins environmental policy by providing indispensable data. Authorities can rely on the analysis to make informed decisions regarding pollution control measures and public health initiatives. Continued advances in this area promise to yield more sensitive and robust techniques, enabling real-time monitoring that is critical in today’s accelerated pace of environmental change.
Clinical Chemistry
In clinical settings, analytical chemistry serves as the backbone of diagnostic processes. With the ability to assay blood, urine, and other biological fluids, techniques such as spectrophotometry and chromatography allow healthcare professionals to detect, diagnose, and monitor diseases.
For instance, a standard glucose test utilizes enzymatic reactions that are quantifiable through analytical methods. This has direct implications for managing diabetes, showcasing how quick and accurate lab results can save lives.
Collaboration between analytical chemists and medical technologists is growing, leading to impressive strides in personalized medicine. Patient-specific treatments are now becoming a reality, and the role of analytical chemistry is central to this trend. The integration of high-throughput techniques has also expedited drug testing, facilitating quicker diagnosis and treatment plans.
Pharmaceutical Applications
The pharmaceutical industry heavily relies on analytical chemistry to ensure the purity, potency, and safety of drugs. Methodologies like high-performance liquid chromatography (HPLC) are integral in both drug development and quality control processes. They help in characterizing compounds and verifying that they meet regulatory standards before reaching consumers.
Pharmaceutical applications extend beyond mere compliance; they influence formulation strategies as well. For example, understanding the stability of a drug under various conditions helps formulate effective dosage forms. Analytical techniques can pinpoint exact degradation pathways, allowing for better product stability.
In recent years, the trend toward biologics—medications derived from living organisms—has introduced new challenges. Here, analytical chemistry helps ensure consistency and reliability in complex biologics, which often exhibit variability due to their biological nature. Companies are continuously striving to innovate and refine their analytical approaches to keep pace with technological advancements in drug development.

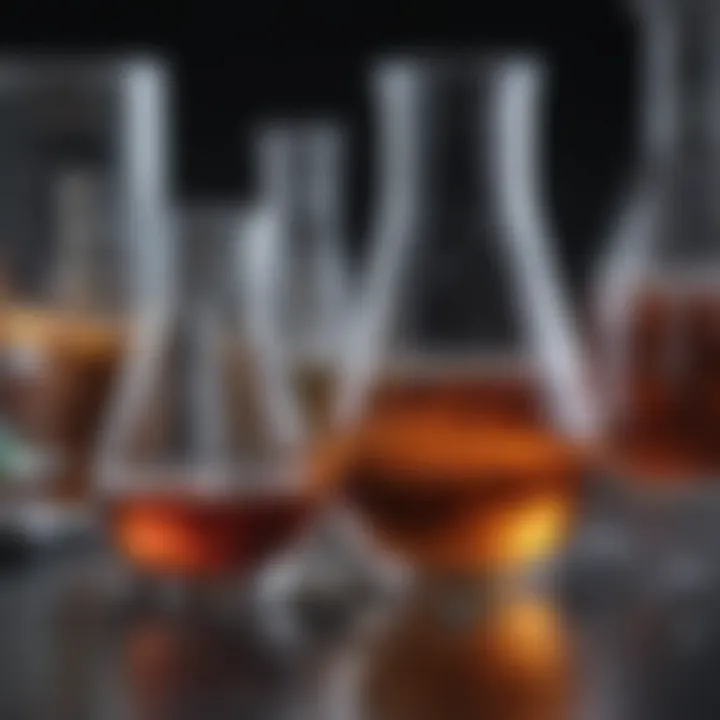
Challenges and Limitations in Analytical Chemistry
In the dynamic field of analytical chemistry, it is crucial to recognize the challenges and limitations that can impede progress. These hurdles not only affect the reliability of results but also influence the effectiveness of analytical techniques employed in various applications. Understanding these factors is that key to improving methodologies and enhancing the overall quality of analytical work. By assessing these challenges, researchers can explore solutions that lead to more accurate data, ultimately paving the way for advancements in science and technology.
Interference and Contamination
Interference and contamination are significant concerns in analytical chemistry. They can compromise the integrity of the data collected and can lead to misleading conclusions. Interference occurs when an analyte is affected by the presence of other substances, which can either enhance or suppress the signal that is measured.
Contamination, on the other hand, is the unintentional introduction of extraneous materials in a sample. It could stem from numerous sources—laboratory equipment, reagents, or even the environment. The consequences can be severe:
- False Readings: Contaminated samples may yield erroneous concentrations of the analyte.
- Compromised Results: Interference can result in the misidentification of substances, leading to incorrect interpretations.
To combat these issues, chemists must implement rigorous sampling protocols and utilize advanced techniques designed to minimize such occurrences. For instance, employing clean room standards and using high-purity reagents can greatly decrease the risk of contamination. Also, proper calibration of instruments helps in recognizing and correcting interferences.
"Avoiding contamination and interference is as vital as the analysis itself; one careless step can lead to a cascade of erroneous findings."
"Avoiding contamination and interference is as vital as the analysis itself; one careless step can lead to a cascade of erroneous findings."
Reproducibility and Accuracy
Reproducibility and accuracy are cornerstones in analytical chemistry that significantly affect the reliability of results. Reproducibility involves the consistency of results when experiments are repeated under the same conditions. In contrast, accuracy refers to how closely the measured values align with the true or accepted values of the analyte.
Achieving high levels of reproducibility and accuracy can be challenging due to a few reasons:
- Variability in Instrument Performance: Instruments can have slight variations over time that lead to differences in measurements.
- Operator Influence: The skills and techniques of the personnel conducting the experiments play a critical role. Even a seasoned chemist can introduce variability if methods are not rigorously followed.
- Environmental Factors: Changes in temperature, humidity, or even electromagnetic interference can skew results.
To ensure both reproducibility and accuracy, it's essential to adopt standardized protocols, regular equipment maintenance, and thorough training. Statistical methods can also be employed to analyze the data, identifying patterns or irregularities that may arise during the testing process. By recognizing these challenges, chemists can work on continually refining their methods, ultimately leading to more reliable analytical outcomes.
Emerging Trends and Future Perspectives
As analytical chemistry moves forward, the landscape is becoming increasingly dynamic and sophisticated. In this section, we delve into two primary trends that are reshaping the field: miniaturization and automation, alongside the integration of artificial intelligence. Both are not just driving innovations but also expanding the possibilities for research and application in various realms, from environmental monitoring to drug development.
Miniaturization and Automation
The shift toward miniaturization in analytical chemistry is akin to downsizing a cumbersome machine into a sleek, efficient device. This advancement ultimately leads to increased sensitivity and lower sample requirements, making it easier and cheaper to conduct analyses. For instance, microfluidics is one area that has gained traction in recent years. These systems allow chemists to manipulate tiny amounts of fluids in channels just a few hundred micrometers wide, making experiments not only faster but also more cost-effective.
Some benefits that have emerged from this trend include:
- Higher throughput: Smaller devices can run multiple analyses simultaneously, significantly reducing time spent on each sample.
- Reduced waste: With less sample required for testing, the amount of waste produced is minimized, addressing environmental concerns.
- Portability: Miniaturized instruments can be made portable, facilitating on-site testing in remote locations.
Automation, on the other hand, streamlines processes that were once time-consuming and prone to human error. Instruments can now autonomously acquire and analyze data, leading to more consistent results. Recent advancements in robotics and software algorithms enable sophisticated tasks that traditionally required skilled hands. An example is liquid handling robots capable of performing complex assay setups without oversight.
Integration with Artificial Intelligence
Artificial intelligence is making waves in analytical chemistry by transforming data processing and interpretation. It's like having a personal guide through a dense forest of data, helping chemists to navigate through vast amounts of information with ease. Integrating AI means that techniques can be developed that not only enhance the efficiency of data analysis but also provide deeper insights into the results.
With machine learning algorithms, chemists are now able to:
- Predict outcomes based on historical data. This means faster research cycles and more reliable predictions for experimental results.
- Identify patterns within complex datasets, which may go unnoticed by human analysts. It opens paths to discover novel relationships in chemical properties and behaviors.
- Optimize methods that can enhance accuracy, tailor conditions for specific analyses, or even suggest new experimental approaches.
"Incorporating artificial intelligence allows for more informed decision-making processes in analytical chemistry today, setting the stage for breakthroughs previously thought to be far from reach."
"Incorporating artificial intelligence allows for more informed decision-making processes in analytical chemistry today, setting the stage for breakthroughs previously thought to be far from reach."
As we stand on the brink of these trending methodologies, it becomes clear that emerging innovations are not just upgrades but transformative shifts. These advancements promise to make analytical chemistry more accessible and accurate, ultimately propelling the field into exciting new territories.
Ending
In the realm of analytical chemistry, conclusions serve as a pivotal anchor, tying together the various threads we have examined throughout this article. This section isn't merely a wrap-up; it's where significance crystallizes and insights solidify into a cohesive narrative. The importance of this conclusion lies primarily in its ability to distill vast amounts of information into digestible fragments that readers can reflect upon and apply in future contexts.
Summary of Key Insights
The exploration of analytical chemistry has brought to light several crucial takeaways:
- Diversity of Techniques: From spectroscopic methods to chromatography and mass spectrometry, each technique plays a unique role in unraveling complex chemical puzzles.
- Data Analysis Importance: The necessity of robust data analysis frameworks cannot be understated. It's an integral part of drawing reliable conclusions from empirical data, ensuring accuracy, and enhancing reproducibility.
- Applications Across Fields: The breadth of analytical chemistry's applications—from environmental monitoring to clinical diagnostics—demonstrates its indispensable role in advancing various scientific fields.
- Emerging Innovations: With the integration of automation and artificial intelligence, the landscape of analytical chemistry continues to evolve, promising increased efficiency and novel methodologies.
This synthesis underscores how foundational concepts serve as a springboard for ongoing advancements in the field, showing that analytical chemistry is not a stagnant discipline but rather a dynamic one that evolves in response to challenges and opportunities.
Call for Further Research
Looking ahead, the call for further research in analytical chemistry is both critical and pressing. There are a few specific areas that warrant deeper investigation:
- Interdisciplinary Approaches: Merging fields such as computer science with analytical chemistry could lead to groundbreaking advancements in data interpretation and instrument design.
- Enhancing Accuracy and Precision: Novel ways to mitigate interference and contamination issues during analysis need exploration to ensure that results reflect true sample characteristics.
- Sustainability in Analytical Practices: As society becomes increasingly aware of environmental issues, research into sustainable practices and green chemistry within analytical methodologies should be prioritized, aiming to reduce waste and energy consumption.
- Developments in Automation: The future hovers with potential; creating automated processes that require less human intervention could lead to enhanced efficiency and higher throughput in analysis.
In summary, the conclusion encapsulates not only the findings of this article but also indicates pathways for future inquiry. As the field continues to evolve, maintaining an inquisitive mindset and commitment to innovation will undoubtedly spur significant advancements in analytical chemistry.