The Importance of Mass Spectral Databases in Science
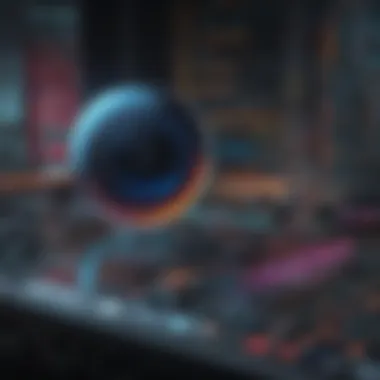
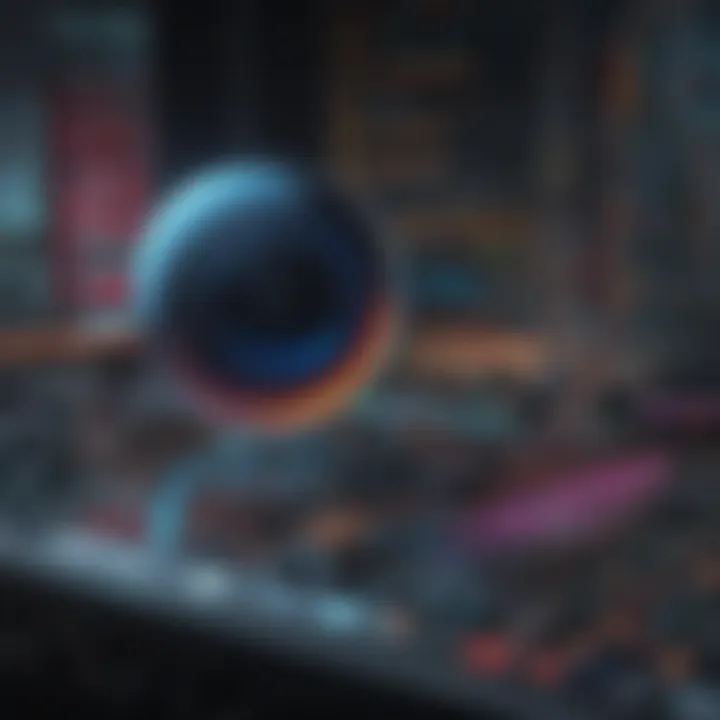
Intro
In the realm of scientific exploration, the intricate web of data plays a pivotal role in shaping our understanding of compounds. At the heart of this endeavor are mass spectral databases, repositories of essential information that serve as a compass for researchers navigating the complex landscape of chemistry and biology. These databases provide a treasure trove of mass spectral data, empowering scientists to identify, quantify, and explore various substances with unparalleled precision.
Mass spectral databases are not just random collections of data; they represent years of meticulous work by professionals dedicated to advancing our scientific knowledge. From the selection of target compounds to the rigorous validation of spectral information, every step is critical. The importance of these databases in contemporary research cannot be overstated, as they facilitate groundbreaking discoveries and innovations across multiple disciplines.
This article will embark on a journey through the essential facets of mass spectral databases, shedding light on their significance, mechanisms of operation, and the evolving role they play in scientific research. We will dive into the methodologies employed in constructing these databases, examine current trends and breakthroughs, and contemplate their future in an ever-changing scientific landscape.
Foreword to Mass Spectrometry
The world of mass spectrometry has become a cornerstone in both analytical chemistry and biological research. Its ability to dissect complex mixtures into identifiable components allows scientists to analyze substances with remarkable precision. Understanding this technique and its applications lays the groundwork for the broader discussions regarding mass spectral databases. These databases serve as an essential repository of mass spectra, providing researchers with the tools needed to discern molecular identities and quantities easily.
What makes mass spectrometry particularly significant in scientific inquiry is not just its stellar performance in identifying compounds, but also how it aids in advancing knowledge across various fields ranging from drug discovery to environmental monitoring. With a robust foundation in mass spectrometry, one can appreciate how mass spectral databases enhance inquiry and contribute to the rigor of modern scientific investigations.
Basics of Mass Spectrometry
To grasp mass spectrometry, one must first understand its fundamental principles. At its core, this technique involves the ionization of chemical species and their subsequent separation based on mass-to-charge ratio. This complex process can be broken down into three primary steps: ionization, acceleration, and deflection.
- Ionization: The sample is vaporized and ionized, often through methods such as Electron Impact (EI) or Electrospray Ionization (ESI). Molecules gain or lose charge, creating ions.
- Acceleration: The ions are then accelerated through an electric field, gaining kinetic energy that plays a vital role in the final analysis.
- Deflection: Finally, ions are deflected based on their mass-to-charge ratio in a magnetic field, allowing them to be detected and analyzed.
The result is a mass spectrum, which is essentially a plot of the abundance of detected ions against their mass-to-charge ratios. This information can provide insights into the molecular weight and structure of the analyzed compounds.
Applications in Scientific Research
The applications of mass spectrometry in scientific research are both varied and profound. In fields such as medicine, pharmaceuticals, and environmental studies, mass spectrometry provides insights that can lead to breakthrough discoveries and innovations. Here are a few notable applications:
- Clinical Diagnostics: In healthcare, mass spectrometry is widely used for biomarker discovery and the identification of metabolic disorders. For example, it can detect small changes in metabolites that are indicative of diseases.
- Proteomics: Researchers utilize mass spectrometry for protein profiling, allowing for the identification of different proteins in a cell or tissue sample. This has implications in understanding diseases at a molecular level.
- Metabolomics: It plays a crucial role in studying metabolic processes, linking metabolites to the physiological state of an organism. This is vital in areas such as drug metabolism and toxicity.
In short, mass spectrometry acts as a powerful tool for researchers, enabling the precise and accurate analysis of complex chemical mixtures, unlocking new possibilities for understanding our world.
Defining Mass Spectral Databases
Mass spectral databases play a pivotal role in the analysis of compounds across various scientific domains. When we speak about defining such databases, it’s essential to acknowledge their multifaceted nature and the significant benefits they offer. First off, these databases are essentially collections of data derived from the masses and relative abundances of ions produced during mass spectrometry experiments. This definition barely scratches the surface; understanding the nuances of these databases is crucial for anyone delving into scientific research.
The importance of defining mass spectral databases lies in the clarity it provides to researchers. By comprehensively outlining what constitutes these databases, one can better appreciate their functionalities and limitations. For instance, while some may assume that a mass spectral database is simply a repository of mass spectra, it encompasses much more, including the metadata that comes with spectral data, such as compound names, chemical structures, and experimental conditions. This information adds layers of context that are invaluable for effective analysis.
What Constitutes a Mass Spectral Database?
A mass spectral database is not just a lump of spectral data thrown together. It is a well-structured system designed for easy access and analysis. To put it simply, a mass spectral database consists of:
- Mass Spectra: The core component is, of course, the mass spectra of various compounds. Each spectrum should be precise and reproducible, playing a crucial role in identifying compounds.
- Metadata: This includes crucial details like the identity of the compound, its chemical formula, and the conditions under which the data was collected. For example, if a researcher looks up caffeine in a database, they should find not just its mass spectrum but also information regarding the ionization method used to create that spectrum.
- Data Formats: The information must be stored in formats that allow for standardization and ease of use. This includes structured formats like XML or CSV that facilitate sharing and analysis across different software platforms.
Ultimately, mass spectral databases serve as a treasure trove of information for scientists seeking to identify and quantify substances with precision.
Key Components and Structures
Delving deeper into the architecture of mass spectral databases, several key components are fundamental to their functionality. Understanding these can provide insights into how to best utilize them in research.
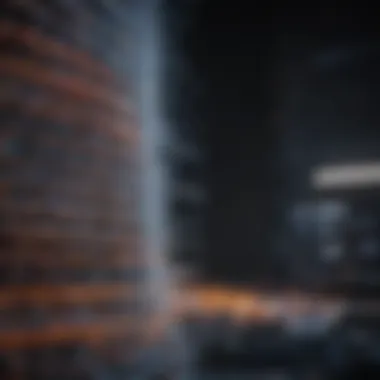
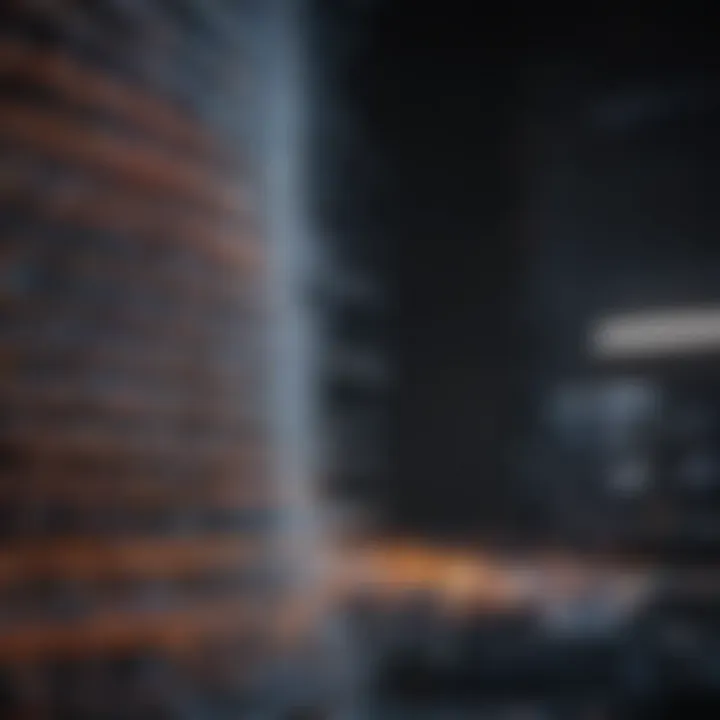
- Database Management Systems (DBMS): These are software applications that store and manage databases. A robust DBMS enables secure and efficient retrieval of mass spectral data.
- Search Algorithms: Effective search algorithms, such as those employing a similarity index, allow researchers to quickly find relevant spectra when queried. This feature is crucial for identifying unknown compounds efficiently.
- Integration Tools: Many mass spectral databases are designed to integrate seamlessly with other analytical tools. For example, they may offer plugins to software like ChemSpider or NIST, thus facilitating comparative studies.
- User Interface (UI): A clear and user-friendly interface is important for researchers who may not be tech-savvy. A good UI breaks down complex queries into simpler tasks, allowing users to navigate the database effortlessly.
- Archiving System: Proper archiving ensures that older data remains accessible without cluttering the main database. This is particularly useful for longitudinal studies or comparative historical analyses.
In summary, mass spectral databases are intricate systems, combining various components that work cohesively to facilitate scientific research. By understanding their definitions and structures, researchers can better exploit these databases, leading to more effective experiments and analyses.
Construction of Mass Spectral Databases
The construction of mass spectral databases is a foundational element in making mass spectrometry a formidable tool in scientific research. Without these databases, scientists would be left to swim without a paddle, trying to decipher and identify substances purely from raw data. The intricate process involved in building these databases not only enhances the reliability of data but also ensures that it is readily available for various research applications.
Data Collection Techniques
Collecting data is the first stepping stone in building any mass spectral database. Various techniques can be employed to gather mass spectral data effectively. Some common methods include:
- Direct Analysis in Real Time (DART): This technique allows for rapid data collection from samples without extensive preparation, offering crucial insights into complex mixtures.
- Matrix-Assisted Laser Desorption/Ionization (MALDI): This method is particularly effective for large biomolecules, capturing the nuances of their mass spectra.
- Gas Chromatography-Mass Spectrometry (GC-MS): Used extensively in organic chemistry, this combined technique helps in separating and identifying compounds by their mass spectra.
Each of these techniques contributes varying levels of specificity and resolution in the data collected, ultimately impacting the quality of the database. It is important for researchers to carefully select a technique that aligns with their research goals while ensuring that it captures the data accurately.
Data Curation Process
Once the data is collected, it does not simply sit there awaiting analysis. Instead, a rigorous curation process comes into play. Curation involves several steps to ensure that the data is:
- Accurate: Duplicates or erroneous entries must be caught and eliminated.
- Comprehensive: All relevant data points need to be included, so that users see a full picture.
- Standardized: Formatting and terminologies should be consistent to facilitate ease of use and analysis across various studies.
Ultimately, a thoughtfully curated dataset acts as a lifeline for researchers, providing them with reliable data that can be leveraged for compound identification and quantification.
Quality Control Measures
Quality control is of paramount importance in maintaining the integrity of a mass spectral database. Several measures can be taken to ensure that the data contained within it stays relevant and trustworthy:
- Regular Audits: Conducting periodic reviews of database entries allows for correction of any inaccuracies that may surface over time.
- User Feedback Mechanisms: Implementing channels for users to report errors can contribute immensely to ongoing quality improvement.
- Updates Based on New Standards: As technology and methodologies evolve, databases need to adapt. Staying informed of best practices and updating the database accordingly is essential.
Reliable high-quality data is non-negotiable in scientific research; it builds trust in the findings and, more importantly, drives future discoveries.
Reliable high-quality data is non-negotiable in scientific research; it builds trust in the findings and, more importantly, drives future discoveries.
Constructing a mass spectral database is far from a simple task. It encompasses thoughtful data collection methods, meticulous curation processes, and unwavering commitment to quality control measures. Without these essential elements, the potential for mass spectral databases to contribute valuably to scientific research would be significantly hampered.
Types of Mass Spectral Databases
Understanding the different types of mass spectral databases is crucial, as each serves distinct functions and purposes in scientific research. These databases can be likened to the many aisles in a library, each carefully curated to cater to the diverse needs of researchers, educators, and professionals across various fields.
Public vs. Private Databases
Public databases, such as the National Institute of Standards and Technology (NIST) Mass Spectral Database, provide free access to a plethora of mass spectral data. This openness fosters collaboration and innovation, allowing scientists across the globe to share and utilize critical information without budget constraints. Because of their wide accessibility, public databases often become the go-to starting point for researchers seeking to identify unknown compounds or confirm findings.
On the flip side, private databases, maintained by commercial organizations, typically require a subscription or one-time payment for access. These databases can offer enhanced features, more refined data, or specialized information that might not be found in public repositories. For instance, certain industries may need proprietary data for specific compounds, driving them toward these tailored resources. The choice between public and private often hinges on a researcher's budget, the specificity of the data required, and the desired level of support and updates.
Consider a scenario where a chemist is studying a new synthetic compound. If they turn to a public database, they may get the basic mass spectrum needed for identification. However, should they opt for a private database, they might find detailed, curated information on similar compounds or even insights derived from proprietary algorithms, enhancing their research depth significantly.
Maintaining a balance between public and private resources is essential. Researchers must assess the trade-offs and potential benefits. While public databases can serve as a foundation, private databases might offer that extra edge in competitive research domains.

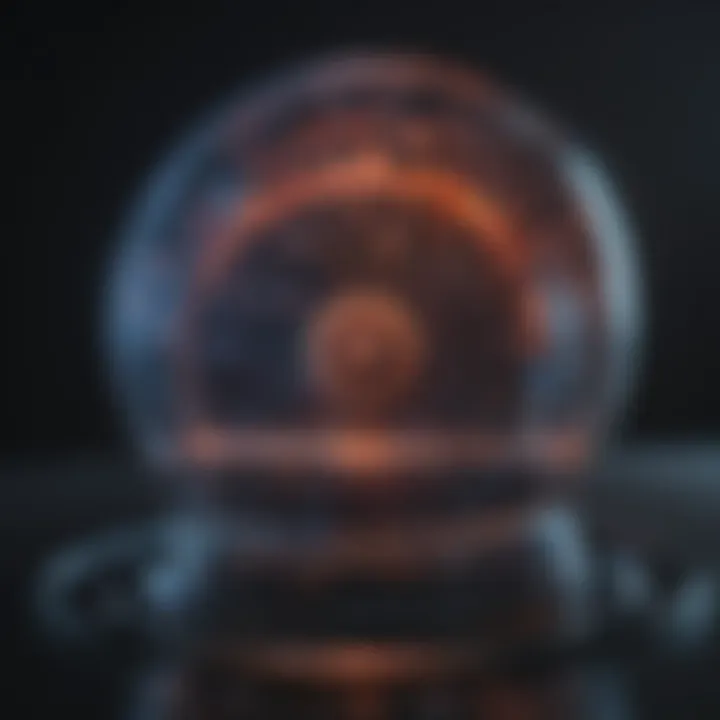
Specialized Databases for Niche Applications
The landscape of mass spectral databases also includes specialized repositories. These databases focus on niche applications or fields, such as metabolomics, proteomics, and environmental sciences. They are valuable resources, providing tailored mass spectra for specific molecules or types of analyses that general databases may overlook.
For example, consider the METLIN database dedicated to metabolomics, which houses a vast array of metabolites. Researchers working in this area can find detailed mass spectra and related information for compounds relevant to metabolic pathways, which may not be available in general databases. This specificity saves time and helps in the effective identification of closely related compounds that might otherwise be easily confused.
Using specialized databases can significantly enhance research outcomes, resulting in better identification accuracy and more nuanced understanding of complex biological processes. Given the rapid evolution of various scientific fields, staying informed about newly launched or updated specialized databases also becomes crucial. Integration of mass spectral data from these databases into existing workflows can improve the overall quality of research, making it a central pillar in today's research environment.
"Specialized databases are like treasure maps in the world of scientific exploration; they lead researchers to the unique insights that shape our understanding of complex phenomena."
"Specialized databases are like treasure maps in the world of scientific exploration; they lead researchers to the unique insights that shape our understanding of complex phenomena."
In summary, knowing the types of mass spectral databases—public, private, and specialized—empowers researchers to make informed choices. Understanding these variations can ultimately dictate the success of scientific endeavors across different fields.
Utilization of Mass Spectral Databases
The utilization of mass spectral databases plays a pivotal role in scientific research, especially in fields like chemistry, toxicology, and pharmacology. These databases serve not just as repositories of data but as powerful tools that facilitate deeper understanding and discovery in various disciplines. By leveraging the wealth of information stored in these databases, researchers can effectively identify unknown compounds and delve into advanced quantitative analysis, providing crucial insights that can lead to groundbreaking discoveries.
Identification of Unknown Compounds
One of the standout features of mass spectral databases is their ability to identify unknown compounds through the analysis of their mass spectra. When researchers encounter a new substance, they can input its spectral data into a relevant database, enabling rapid comparison against a vast array of existing spectra. This process is like looking for a familiar face in a room full of strangers; if the database has it cataloged, finding it is relatively straightforward.
- Speed and Efficiency: The manual analysis of mass spectra can be time-consuming and intricate. With databases, researchers can narrow down potential candidates quickly, cutting down the time from hours to mere minutes. This is particularly beneficial in industries where time-to-market is critical, such as pharmaceuticals.
- Improved Accuracy: By cross-referencing multiple spectral entries, researchers can increase the probability of accurate identification. Mass spectral databases continually update their libraries as new compounds are discovered, making them more reliable over time.
"A well-constructed database not only enhances accuracy but also broadens the scope of research possibilities."
"A well-constructed database not only enhances accuracy but also broadens the scope of research possibilities."
Quantitative Analysis Capabilities
Beyond merely identifying substances, mass spectral databases excel in quantitative analysis. This involves measuring the concentration of compounds within a sample, which is vital in various applications, from monitoring environmental pollutants to ensuring drug dosage accuracy.
- Measurement Consistency: By employing established quantitative methods and comparing them against reference spectra in databases, scientists can achieve consistent and reproducible results. This is crucial for regulatory compliance in industries such as food safety and pharmaceuticals.
- Data Integration: Many mass spectral databases allow integration with other analytical tools, providing a more comprehensive view of a sample's composition. This can include chromatographic data, which when paired with mass spectral data, enhances the robustness of the analysis.
In an age where the complexity of compounds is ever-increasing, the integration of mass spectral databases into research methodologies not only streamlines processes but also fosters innovation. The capacity to identify unknown substances and perform quantitative analyses paves the way for advancements in various scientific fields, underscoring the necessity of these databases in contemporary research.
Challenges in Mass Spectral Databases
The role of mass spectral databases is crucial in shaping scientific endeavors, but they are not without their hurdles. Understanding these challenges is essential for harnessing the full potential of these databases. As scientific inquiry grows more complex, the intricacies of maintaining and improving mass spectral databases cannot be overlooked. The importance of this topic lies in the implications for data integrity, the continual evolution of standards, and the adoption of new technologies, which are all interlinked in providing reliable and actionable scientific data.
Data Integrity Issues
Data integrity serves as the cornerstone of any reliable mass spectral database. Without it, the credibility of the results one obtains from these databases is compromised. Various factors can undermine data integrity, including:
- Errors in Data Entry: It’s not unusual for human errors to creep in during the data input phase. These mistakes can distort the data and lead to incorrect interpretations.
- Instrument Drift: Mass spectrometers need regular calibration. If an instrument drifts over time without being recalibrated, the resulting data can be inconsistent.
- Data Loss or Corruption: Digital data can be lost or corrupted due to technical failures or improper storage practices, which is detrimental to the overall reliability of the database.
Ensuring the integrity of data involves stringent checks and balances. Regular audits, comprehensive data validation techniques, and collaborative efforts among researchers foster an environment where quality can be maintained. It becomes vital for database custodians to establish transparent processes that allow real-time updates while safeguarding the original data integrity.
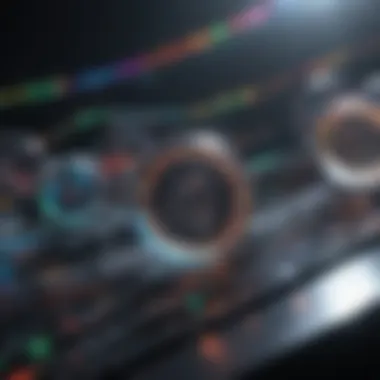
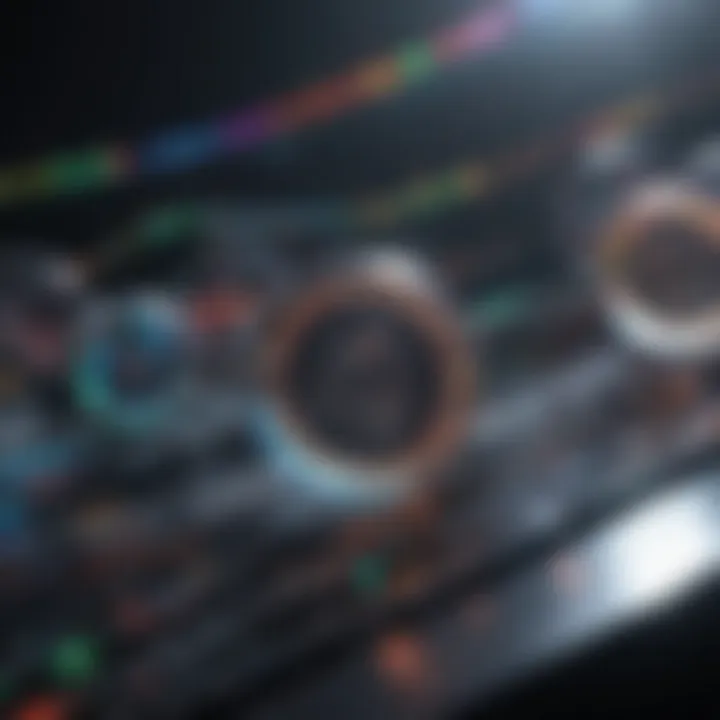
"Consistency and precision in mass spectral data is non-negotiable for successful scientific outcomes."
"Consistency and precision in mass spectral data is non-negotiable for successful scientific outcomes."
Evolving Standards and Technologies
Another significant challenge pertains to the ever-evolving landscape of standards and technologies that govern the use of mass spectral databases. The realm of mass spectrometry is dynamic, characterized by:
- Emerging Analytical Techniques: As new technologies arise, they can render existing standards outdated. For example, advancements in ionization methods can change the spectrum of data available and, inversely, the interpretations of existing data.
- Interoperability: As scientific research often involves collaboration across disciplines, the need for databases to work together seamlessly is critical. However, varying standards can pose challenges, resulting in data silos.
- Regulatory Compliance: Organizations and institutions must navigate the convoluted maze of compliance with local and international regulations. Keeping databases up-to-date with these requirements demands proactive management.
Adaptation to rapid technological innovations is non-negotiable for the continuation of science. Therefore, ongoing dialogue among scientists, database developers, and regulatory bodies is paramount. It's not only about keeping pace but also about setting standards that can be flexible and scalable to accommodate the pace of innovation.
Future Directions in Mass Spectrometry and Databases
The landscape of mass spectrometry is continuously reshaping itself, and with it comes the potential for mass spectral databases to evolve into more powerful tools for scientific research. Understanding these future directions is essential for students and professionals keen on harnessing the full capability of this technology. As the push for greater precision and efficiency grows within the scientific community, mass spectral databases will undoubtedly play a central role in advancing our knowledge.
Integration with Other Analytical Techniques
The integration of mass spectrometry with other analytical techniques presents a remarkable prospect for enhancing the capabilities of scientific research. Techniques like chromatography, nuclear magnetic resonance (NMR), and infrared spectroscopy, when paired with mass spectral data, allow researchers to cross-validate findings and achieve a more holistic view of chemical compounds.
By melting together various methods, we can tackle much more complex samples. This will inevitably lead to richer, more nuanced data that can support more profound insights into molecular behavior. For instance, coupling mass spectrometry with chromatographic methods can result in clearer separation and identification of compounds in mixtures, enhancing both the accuracy and repeatability of results.
Additionally, utilizing multiple techniques can aid in detecting elusive substances. The combination of results can act like pieces of a puzzle coming together to give a complete picture, making it easier to identify unknown compounds or characterize complex biological samples.
The Impact of Artificial Intelligence and Machine Learning
Artificial intelligence and machine learning are the buzzwords in modern science, and mass spectral databases are positioned to be significantly impacted by these technologies. The sheer amount of data generated by mass spectrometry can be overwhelming, but with AI tools designed to sift through vast datasets, researchers can uncover trends and insights that a human alone might miss.
For example, machine learning algorithms can improve the accuracy of compound identification by learning from previous mass spectral data and predicting outcomes based on similarities in molecular weights and fragments. In cases where time is of the essence, such as in clinical diagnostics or environmental monitoring, AI can offer timely insights that facilitate rapid decision-making.
Furthermore, AI systems can help automate the data curation process, making it less prone to human error and more efficient. This means that databases can be updated swiftly and maintained with consistent accuracy, ensuring that researchers have access to the latest and most reliable data.
"The future of mass spectrometry is not just in identifying compounds but in understanding the magnitude of data we can analyze and interpret through advanced technologies."
"The future of mass spectrometry is not just in identifying compounds but in understanding the magnitude of data we can analyze and interpret through advanced technologies."
In summary, the future directions in mass spectrometry and its databases point towards an era where integration with other analytical techniques and the influx of AI will refine the processes of identification and analysis. This evolution in technology not only holds promise for greater accuracy and efficiency, but also for fostering innovative research across diverse fields, from drug discovery to environmental studies.
Finale
The closing segment of this article presents a pivotal moment to reflect on the vital role that mass spectral databases play in scientific research. By tracing back through the previous sections, we observe a landscape that emphasizes accuracy, consistency, and continual evolution in understandings of chemical compounds. The intricate interplay between technology, data integrity, and evolving methodologies demonstrates that mass spectral databases are more than mere repositories; they serve as foundations upon which much scientific discovery rests.
Recap of Significance
In this discourse, we emphasized the significance of mass spectral databases in various fields. These databases not only bolster the identification of unknown substances but also enhance quantitative analysis capabilities. It’s paramount to recognize how these resources provide researchers with reliable and comprehensive data, essential for advancing scientific inquiries. Furthermore, the vast scope encompassing both public and specialized databases showcases a commitment to fostering innovation. A few critical insights from our exploration include:
- Data Integrity: Ensuring that the information remains credible and updated is crucial for reliable outcomes.
- Diversity of Applications: From pharmaceuticals to environmental studies, the influence of these databases spans a multitude of disciplines.
- Collaboration Possibilities: The integration of mass spectrometry with other analytical techniques can amplify research potential exponentially.
Through these elements, it's clear that mass spectral databases are indispensable tools that contribute directly to the rigor of scientific exploration.
The Continued Relevance in Research
As we look to the future, the relevance of mass spectral databases cannot be overstated. Scientific research continually evolves, and so too must the databases that support the discoveries within it. With advancements in artificial intelligence and machine learning, these databases are poised to become even more dynamic and user-friendly. They will not only act as data banks but also serve as intelligent systems that can predict outcomes, suggest analytical pathways, and assist researchers in real-time.
Moreover, the ongoing challenges around data integrity and standardization underscore the need for dedicated efforts toward maintaining the quality of these resources. As new compounds are discovered and existing data is refined, mass spectral databases will adapt, ensuring that researchers have access to the most relevant and accurate information available.