Exploring the Interconnectedness of Gravity and Weight
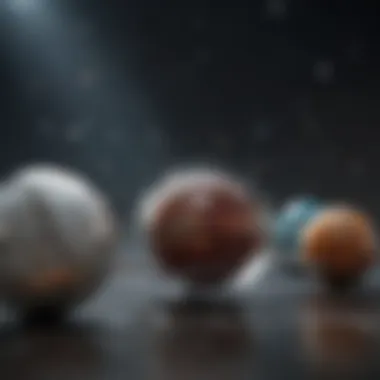
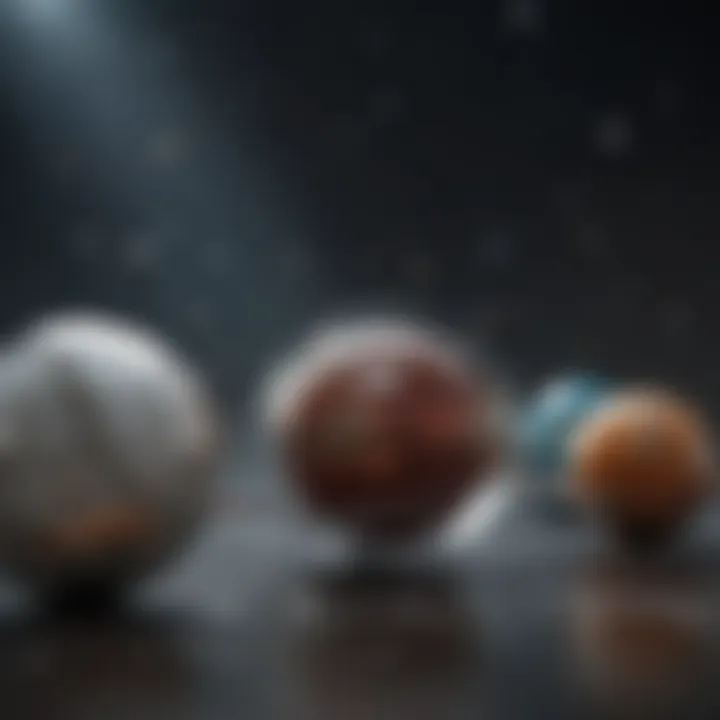
Intro
Understanding the nuances of gravity and weight is fundamental in comprehending the physical world. These two concepts are often mistakenly viewed as interchangeable, yet they hold distinct definitions and implications in the realm of physics. Gravity is the force that attracts a body toward the center of the earth or toward any other physical body having mass. Weight, on the other hand, is the measure of the gravitational force acting on an object. This article aims to clarify their interconnectedness while exploring their significance in both celestial mechanics and everyday phenomena.
In exploring this subject, we will delve into key concepts, examine current research trends, and uncover how gravity affects weight under varying conditions. By identifying the underlying scientific principles, we can gain a deeper appreciation of these forces in action. Let's begin by defining the main idea of gravity and weight.
Key Concepts
Definition of the Main Idea
Gravity can be described as a natural phenomenon by which all things with mass are brought towards one another. Sir Isaac Newton's law of universal gravitation states that every mass attracts every other mass in the universe, a concept crucial to understanding celestial movements. The strength of this attraction is dependent on two key factors: the masses of the objects and the distance between them.
Weight is the resultant force experienced by an object due to gravity. It is quantified by the equation:
Weight (W) = mass (m) × gravitational acceleration (g).
Here, gravitational acceleration is approximately 9.81 m/s² on Earth. This equation illustrates that weight varies according to gravitational conditions.
Overview of Scientific Principles
Various scientific principles govern the relationship between gravity and weight. The most notable among them is the inverse-square law, which states that the force of gravity decreases with the square of the distance between two objects. This law is essential in understanding why weight decreases as one moves further from a planet's surface and how this principle applies to celestial bodies.
Additionally, concepts in physics like mass, density, and buoyancy interact with gravity to influence the perception of weight. For example, an object in water may weigh less than it does in air due to the buoyant force that counteracts the pull of gravity, minimizing its effective weight in that environment.
"Gravity is not merely a force; it is an intrinsic property of mass that shapes the very fabric of the universe."
"Gravity is not merely a force; it is an intrinsic property of mass that shapes the very fabric of the universe."
Current Research Trends
Recent Studies and Findings
Recently, research has started to unfold new dimensions in understanding gravity's interactions with weight. Studies employing advanced technology, like gravitational wave detectors, have begun to study how gravity behaves in extreme environments, such as near black holes. These findings have implications not only for theoretical physics but also for how we understand weight on a cosmic scale.
Significant Breakthroughs in the Field
Significant breakthroughs have arisen from investigations surrounding the nature of dark matter and dark energy, which fundamentally influence gravitational forces. Understanding these components may reveal new insights into how weight acts in varying cosmic contexts. Research continuously progresses, shedding light on the complexities that govern gravitational interactions and their broader implications.
By examining these key concepts and trends, we can appreciate the profound implications of gravity and weight in scientific discourse, paving the way for advanced exploration in physics.
Understanding Gravity
Understanding gravity is essential for grasping the deeper aspects of physics and its implications in our everyday life. Gravity governs not only the motion of celestial bodies but also the behavior of objects here on Earth. By exploring the principles of gravity, one can gain insight into phenomena ranging from the fall of an apple to the orbit of planets. The connection between gravity and weight is crucial when analyzing physical interactions in the universe.
The study of gravity provides a foundation for disciplines like astrophysics, engineering, and even philosophy. It allows us to predict how objects will move and interact under varying forces. Furthermore, insights into gravity enhance our understanding of the fabric of space-time and the larger cosmos. This discussion aims to unravel the significance of gravity and set the stage for examining its relationship with weight, culminating in an exploration of real-life applications.
Definition of Gravity
Gravity is a natural force that attracts two bodies towards each other. This universal force operates at any distance, with its strength dependent on the mass of the objects involved and the distance between them. In essence, more massive objects exert a stronger gravitational pull. Gravity is vital for maintaining the structure of the universe, holding stars, planets, and galaxies in place.
Historical Perspectives on Gravity
Aristotle's Theory
Aristotle's view of gravity presented it as a force that pulls heavier objects downwards. He believed in a hierarchical universe, where lighter elements like fire and air moved upward, while heavier elements like earth moved downward. This theory, though simplistic, served as the foundation for many ancient thoughts regarding motion and led to a long-standing belief that weight directly correlated with speed of fall.
Newton's Law of Universal Gravitation
Isaac Newton revolutionized the understanding of gravity with his Law of Universal Gravitation. He posited that every object in the universe attracts every other object with a force that is directly proportional to the product of their masses and inversely proportional to the square of the distance between them. This understanding was significant as it quantified gravitational forces and allowed calculations of trajectories, thus paving the way for modern physics. The elegance of Newton's law lies in its predictability; however, it did not address the complexities of light or gravity in extreme scenarios.
Einstein's General Relativity
Albert Einstein expanded upon Newton’s ideas with his theory of General Relativity, introducing the notion of gravity as a curvature of space-time rather than just a force. It asserts that massive bodies like the Earth warp the space around them, causing objects to follow curved paths. This perspective offered a deeper understanding of gravity, especially in extreme conditions, such as near black holes. The theory has been substantiated through experimental validation, showcasing its relevance and adaptability.
The Nature of Gravitational Force
Mass and Distance
The relationship between mass and distance is central to understanding how gravitational forces work. According to Newton's law, the gravitational force increases with mass and decreases with distance. An important element of this principle is the gravitational force formula, formulated as F = G (\fracm_1 \times m_2r^2). Here, (F) represents the gravitational force, (G) is the gravitational constant, (m_1) and (m_2) are the masses of the two objects, and (r) is the distance between them. This equation highlights that the force diminishes significantly as distance increases.
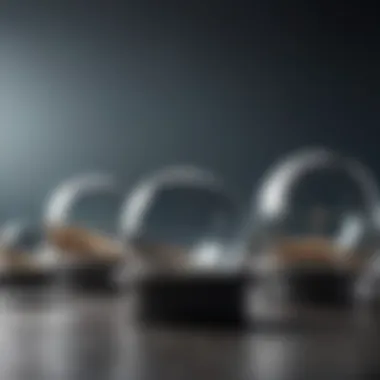
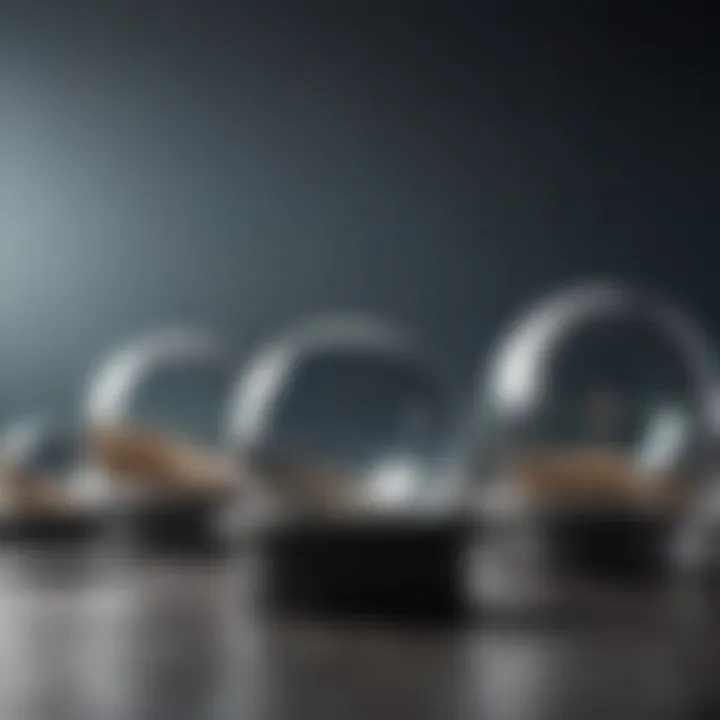
Gravitational Constant
The gravitational constant, denoted by G, plays a critical role in expressing gravitational interactions. The value of (G) is approximately 6.674 (× 10^-11 m^3 kg^-1 s^-2). This constant provides a baseline for calculating gravitational forces and varies with our understanding of gravity and mass. The universality of G signifies that it remains constant throughout the universe. Its implications can be profound when analyzing gravitational forces under varied contexts.
Defining Weight
Defining weight is essential in understanding its nuanced relationship with gravity. Weight is not merely a term used in everyday conversations; it has significant implications in scientific disciplines, especially in physics and engineering. This section aims to clarify the concept of weight and its underlying factors, providing a basis for exploring its connection to gravitational forces.
The significance of comprehending weight extends to various fields. In everyday life, the understanding of weight impacts practical activities such as cooking, transportation, and safety. Schools teach weight in basic physics for students to grasp foundational knowledge. For researchers and engineers, a precise understanding of weight is crucial for calculations involving forces, materials, and structural integrity.
What is Weight?
Weight is defined as the force exerted on an object due to gravity. This force depends on both the mass of the object and the gravitational acceleration at that location. The formula for weight is:
Weight (W) = Mass (m) × Gravitational Acceleration (g)
In the context of Earth, gravitational acceleration is approximately 9.81 meters per second squared. This means that an object with a mass of one kilogram experiences a weight of about 9.81 newtons.
Weight can vary based on location due to differences in gravitational pull. For example, on the Moon, where gravity is about one-sixth that of Earth's, an object will weigh significantly less than it does on Earth.
Weight Versus Mass
Mass and weight are often used interchangeably in casual conversation, but they refer to distinct physical properties.
- Mass is a measure of the amount of matter in an object, typically measured in kilograms or grams. It does not change regardless of where the object is located in the universe.
- Weight, on the other hand, is the force acting on that mass due to gravity. It can change depending on the gravitational field strength in that location.
For instance, a person weighing 70 kilograms on Earth would have a different weight on Mars or the Moon due to the variations in gravitational acceleration.
Understanding these differences is crucial in fields such as physics and engineering, where the distinction can have significant implications for design, safety, and functionality.
"The accurate distinction between weight and mass is foundational for all scientific work involving force and motion."
"The accurate distinction between weight and mass is foundational for all scientific work involving force and motion."
The Relationship Between Gravity and Weight
Understanding the relationship between gravity and weight is crucial in grasping fundamental principles in physics. Gravity is a force that attracts two bodies towards each other, while weight is a measure of the force exerted on an object due to gravity. The interplay between these concepts is not only foundational in scientific studies but also has practical implications in everyday life. Recognizing how weight varies based on gravity helps in various fields such as engineering, aviation, and space exploration. This section discusses how weight is influenced by gravity and the role of gravitational fields in determining weight.
How Weight is Affected by Gravity
Weight is the result of gravitational force acting on an object's mass. This relationship can be summarized with the equation:
[ W = m imes g ]
Where W is weight, m is mass, and g represents the gravitational acceleration. Since gravity varies depending on location, weight is not a constant for a given mass. For instance, an object on Earth weighs more than it would on the Moon due to the difference in gravitational pull. The acceleration due to gravity on Earth is approximately 9.81 m/s², while on the Moon, it is roughly 1.62 m/s². Thus,
- An object weighing 50 kg on Earth has a weight of about 490 Newtons.
- That same object would weigh about 80 Newtons on the Moon.
This illustrates that the value of weight changes with the local gravitational field, hence it is critical to understand how gravity impacts weight in different environments.
Gravitational Fields and Their Effects on Weight
A gravitational field represents the influence that a massive body exerts on the surrounding space. This field can be visualized as a region in which other masses experience weight due to the gravitational pull. The strength of the gravitational field is determined by two factors: the mass of the attracting body and the distance from that body.
- Mass: Greater mass results in a stronger gravitational field.
- Distance: The further away you are from the mass, the weaker the gravitational pull. This follows the inverse-square law, which states that gravitational force decreases with the square of the distance.
Consider the different gravitational fields:
- Earth: Strong gravitational field due to its large mass, resulting in higher weight for objects.
- Moon: Weaker field leads to significantly reduced weight for the same mass.
- Mars: Its gravity is about 0.38 times that of Earth's, meaning weight is still lower than on Earth but higher than on the Moon.
Understanding these gravitational fields aids in calculating weight accurately in various locations.
"An awareness of how gravitational fields work is essential for comprehending weight variations across different celestial bodies."
"An awareness of how gravitational fields work is essential for comprehending weight variations across different celestial bodies."
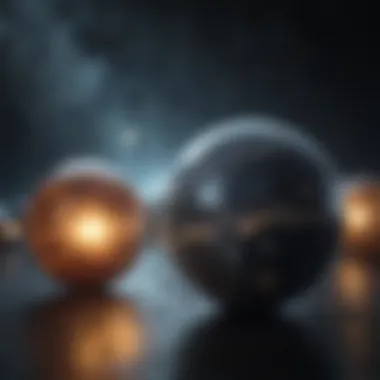
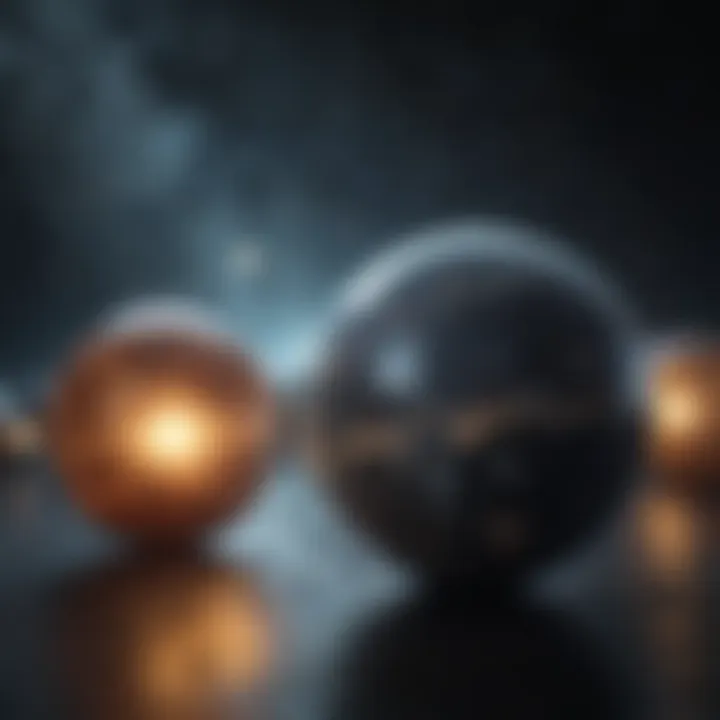
In summary, gravity plays a vital role in defining weight, and variations in gravitational fields lead to different weight measurements. These factors are important not only for theoretical physics but also for applied sciences. They determine how objects behave in various gravitational environments, impacting areas such as astronaut training, spacecraft design, and our general understanding of the universe.
Mathematics of Gravity and Weight
The mathematics of gravity and weight is pivotal in understanding how these forces interact in both theoretical and practical contexts. This section will address how equations and calculations underpin the concepts of weight and gravity, laying the foundation for deeper exploration of their implications.
Mathematical principles help us quantify the forces acting upon objects, enabling precise predictions and measurements. This is especially crucial in scientific research and engineering applications. Furthermore, understanding the mathematics involved also reinforces the interdependence of weight and gravitational forces.
The Equation for Weight
Weight Calculation Formula
The weight calculation formula is expressed as ( W = mg ), where ( W ) signifies weight, ( m ) represents mass, and ( g ) is the acceleration due to gravity. This straightforward formula allows for quick calculations of weight based on an object's mass and the gravitational field strength.
One of the key characteristics of this formula is its simplicity. It effectively demonstrates how mass and gravity work together to define weight. This characteristic makes it a popular choice for educational purposes, helping students grasp foundational physics concepts.
The unique feature of the weight calculation formula is its universal application, despite varying gravitational conditions. It means that while the value of ( g ) changes across different celestial bodies, as long as mass is known, weight can be calculated. However, one disadvantage is that it doesn’t account for factors such as air resistance or rotational influences, which can affect precise weight measurements in practical scenarios.
Units of Measurement
Units of measurement used for weight include Newtons (N) and pounds (lb). In the International System of Units (SI), weight is measured in Newtons, aligning with the physics of motion and forces. This characteristic supports a coherent framework for scientific research.
The unique feature of using Newtons is that it directly connects weight with the fundamental units of mass and acceleration. This integration benefits scientific communication, ensuring clarity when discussing experimental results. However, the use of imperial units like pounds may be more familiar in some countries, which can lead to confusion.
Variations in Weight Across Celestial Bodies
Earth
Weight on Earth serves as the primary reference point for understanding weight in various gravitational fields. Earth's standard gravitational acceleration is approximately ( 9.81 , \mathrmm/s^2 ). This characteristic makes Earth a beneficial point of comparison for weight calculations across different celestial bodies.
One unique feature is the relatively stable gravitational field on Earth, which influences everyday experiences of weight. It allows for predictable outcomes in engineering and design, but the disadvantage is that this stability can limit understanding of how weight functions under varying gravitational conditions encountered in space.
Moon
The Moon presents a fascinating case for weight variation due to its lower gravitational acceleration of around ( 1.62 , \mathrmm/s^2 ). This reduced gravity significantly decreases the weight of objects. This encourages exploration of weightlessness in a way that challenges intuitive understanding of mass and gravity.
The key characteristic of the Moon's gravitational field is its ability to demonstrate how weight can drastically change even with a similar mass. This feature is particularly beneficial for space exploration, aiding in design and research missions by allowing simulations of lunar conditions.
However, a disadvantage is that such stark differences can mislead new learners into over-simplifying gravity's role in physics. Understanding these variations is crucial to clear interpretation.
Mars
On Mars, the gravitational pull is about ( 3.71 , \mathrmm/s^2 ), which is approximately 38% that of Earth's. This weight reduction has significant implications, particularly for potential colonization and production of resources on the Martian surface. The comparative analysis of weight on Mars versus Earth helps scientists understand how human activity could be effectively engineered in extraterrestrial environments.
A key characteristic of Mars is that its gravity allows for a unique exploration of how various factors affect weight. This aspect makes the planet an important subject for astronauts preparing for future missions. However, understanding weight on Mars also presents a challenge: the need to adapt existing technologies and methodologies originally designed for Earth.
Understanding weight on various celestial bodies illuminates the core principles of gravity, and provides significant insights for both scientific inquiry and practical applications across diverse fields.
Practical Implications of Gravity and Weight
Understanding gravity and weight holds significant practical implications. These concepts dictate how objects behave on Earth and in space. Recognizing their importance helps us in academic fields, engineering, and everyday life. Practical applications range from designing sturdy buildings to calculating the weight of objects on different planets.
Everyday Examples of Gravity's Influence
Gravity plays a central role in daily life. For instance, when you drop an object, gravity is the force that pulls it toward the ground. This action is not just a simple phenomenon; it showcases the predictable interaction between mass and gravitational pull. Consider the case of skydiving—parachutists experience increasing speed due to gravitational acceleration until they deploy their parachutes. The understanding of gravity here allows for safer jump techniques and equipment design.
Other instances include how we walk and run. Our bodies adapt to gravitational forces to maintain balance and posture. The importance of gravity in sports cannot be overstated; it affects everything from jumping in basketball to throwing a javelin. Understanding the precise influence of gravity helps athletes improve techniques and maximize performance.
Engineering and Design Considerations
In engineering, gravity's effects must be carefully considered during design and construction. Buildings, bridges, and other structures must withstand gravitational forces. Engineers apply principles of physics to ensure safety and stability. For example, skyscrapers must counteract bending and twisting caused by wind, while ensuring that the gravitational load is appropriately distributed.
Moreover, advancements in materials science have led to lighter, stronger materials that can resist gravitational forces effectively. Engineers utilize knowledge of gravity when planning weight distributions in vehicles, ensuring stability and performance. This knowledge is vital for both land vehicles and aerospace engineering, especially during launches or landings.
Applications in Space Exploration
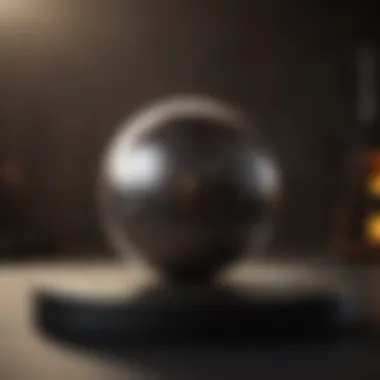
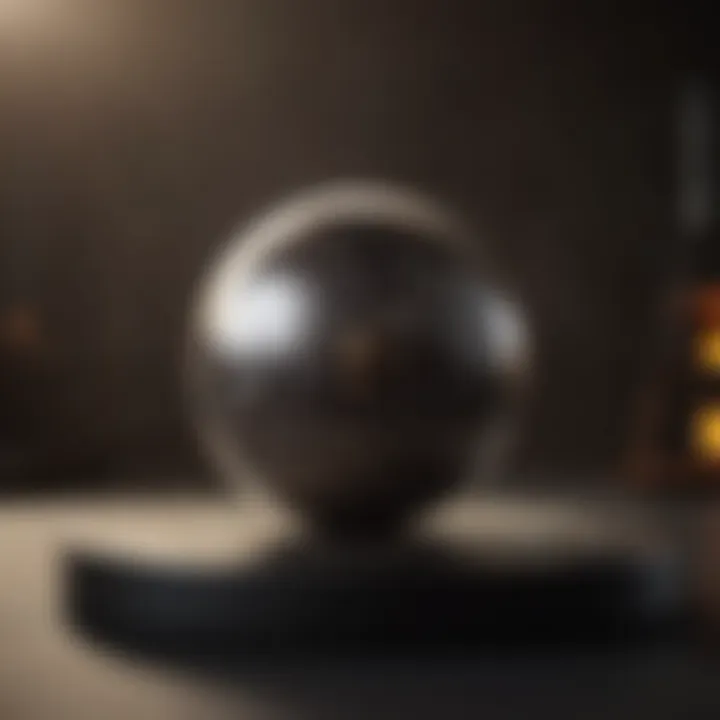
In the realm of space exploration, understanding gravity and weight is crucial. The different gravitational forces on celestial bodies affect how spacecraft are designed and operated. Launch trajectories, fuel requirements, and landing strategies all depend on this knowledge. For instance, landing on the Moon, where gravity is only about one-sixth that of Earth's, requires different calculations and approaches.
The impact of microgravity in the International Space Station (ISS) provides insights into human physiology and material science away from Earth's gravitational pull. Experiments conducted in space yield data that benefits multiple sectors on Earth, including medicine, agriculture, and technology.
Misconceptions and Clarifications
Understanding gravity and weight is fundamental in the field of physics. However, misconceptions are widespread, leading to confusion among students and even educators. Recognizing these misconceptions is important as it ensures accurate scientific communication. Clarity in these concepts promotes better understanding and application in real-world scenarios. Addressing misunderstandings can enhance educational resources and curriculum design, catering to diverse learning needs.
Common Myths About Gravity and Weight
One prevalent myth is that weight and mass are interchangeable terms. This misunderstanding stems from everyday language, where people often equate heaviness with weight. However, according to physics, mass is a measure of matter present in an object, while weight is the gravitational force acting on that mass.
Another common belief is that gravity ceases in space. While it is true that the force of gravity decreases with distance, it never completely disappears. For example, the gravitational influence of Earth extends far into space, affecting satellites and astronauts. Therefore, weight still exists in space, albeit at lower values than on Earth.
Additionally, some think that gravity is a linear force, meaning it affects all objects equally regardless of their properties. In reality, gravity acts on objects based on their mass and the distance between them. Heavier objects exert a greater gravitational pull than lighter ones within the same field. This results in variations in how weight impacts different celestial bodies.
Clarifying the Differences Between Weight and Gravity
To clarify, gravity refers to the natural phenomenon by which all things with mass are attracted to one another. It is a force that operates at a distance, influenced by the mass of objects and their separation. Weight, on the other hand, is a specific manifestation of gravity. It is the force experienced by an object due to gravity.
The formula for weight is:
[ W = mg ]
where ( W ) represents weight, ( m ) stands for mass, and ( g ) is the acceleration due to gravity. This formula demonstrates that weight depends directly on both the mass of an object and the strength of the gravitational pull at a particular location.
In summary, understanding these differences improves both academic and practical knowledge of physics. By resolving myths and clarifying distinctions, individuals can grasp the intricate relationship between gravity and weight, aiding in their studies or scientific endeavors.
Understanding the core principles of gravity and weight is essential for advancing both educational practices and scientific inquiry.
Understanding the core principles of gravity and weight is essential for advancing both educational practices and scientific inquiry.
For deeper exploration into these concepts, resources like Wikipedia and Britannica provide extensive insights into gravitational theories and their implications.
Future Research Directions
The study of gravity and weight is evolving. Research into these topics is significant for both theoretical physics and practical applications. New insights into gravitational interactions can lead to breakthroughs in technology, space exploration, and our understanding of the universe. Exploring future research directions helps identify gaps in current knowledge and suggests where inquiry should focus.
Emerging Theories in Gravitational Physics
Emerging theories in gravitational physics aim to refine our understanding of gravity beyond traditional Newtonian and Einsteinian models. One of the leading candidates is string theory, which posits that fundamental particles are not point-like but rather tiny, vibrating strings. This shift could unify gravity with the other fundamental forces of nature.
Loop quantum gravity is another theory that attempts to quantize gravity. It seeks to reconcile general relativity with quantum mechanics by proposing that spacetime itself has a discrete structure at the smallest scales. Research in this area could lead to profound insights into black holes and the early universe.
Advancements in gravitational wave astronomy have also opened new frontiers. The detection of gravitational waves from merging black holes and neutron stars offers opportunities to test various predictions of general relativity and explore the dynamics of extreme astrophysical events. As observation techniques improve, new phenomena may be discovered that challenge existing theories.
Technological Advances and Their Impact
Technological advances play a critical role in the exploration of gravity and weight. The development of precise measurement devices, such as atomic gravimeters, has enhanced our ability to measure gravitational forces with exceptional accuracy. These devices can lead to applications in geophysics, such as oil and mineral exploration, where understanding gravitational variations in the Earth's crust is essential.
In space exploration, advancements in propulsion technologies continue to reshape our approach to gravitational challenges. For instance, the concept of the ion drive provides a more efficient means of navigation in the gravitational fields of various celestial bodies. This could facilitate longer missions and enable a deeper exploration of the solar system.
Furthermore, artificial intelligence is increasingly being used to process and analyze vast amounts of data generated by gravitational research. Machine learning techniques enable researchers to identify patterns and anomalies in astronomical data, potentially leading to new discoveries regarding gravity's role in the universe.
"The intersection of theoretical research and technological innovation holds the key to unlocking mysteries about gravity's fundamental nature."
"The intersection of theoretical research and technological innovation holds the key to unlocking mysteries about gravity's fundamental nature."
In summary, the future of research in gravity and weight is promising. Emerging theories challenge conventional understanding, while technological advances offer tools for deeper exploration. Keeping abreast of these developments will be crucial for scientists and engineers alike, as they forge paths to new paradigms in physics.
Culmination
The conclusion of this article encompasses several important aspects regarding the connection between gravity and weight. One central affirmation is that understanding this relationship significantly enhances our grasp of fundamental physics. It allows us to visualize how gravity acts on various objects in distinct environments. The insights gained from the analysis can be applied in scientific research, engineering, and education.
Summarizing the Relationship
Gravity and weight are intrinsically linked concepts within the realm of physics. They influence each other in consistent ways, and understanding them is crucial for various fields. Gravity is a force that causes masses to attract, while weight is the force experienced by an object due to gravity's pull on its mass. The equation governing weight, W = m * g, illustrates this connection clearly. Here, W represents weight, m is mass, and g is the acceleration due to gravity. As gravity changes, weight changes proportionately. This relationship is observable not only on Earth but across different celestial bodies. For example, an object weighing 100 kg on Earth will weigh significantly less on the Moon due to its reduced gravitational pull.
Implications for Science and Everyday Life
In everyday life, these concepts quietly shape our experiences. When determining how much weight we can lift or how vehicles operate under different conditions, an implicit understanding of gravity and weight is at play. Moreover, the health sector considers these aspects when developing fitness equipment and rehabilitation plans.
In summary, the nexus between gravity and weight is not merely an abstract academic concept. It underpins much of our understanding of the physical world and informs practical applications that benefit society as a whole.