Membrane Staining Immunofluorescence: Cellular Insights
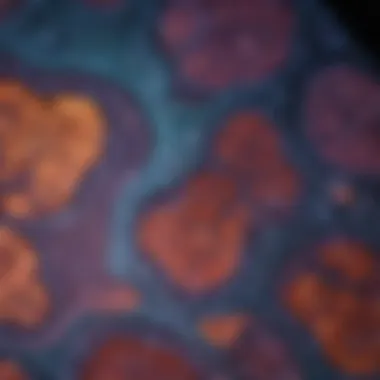
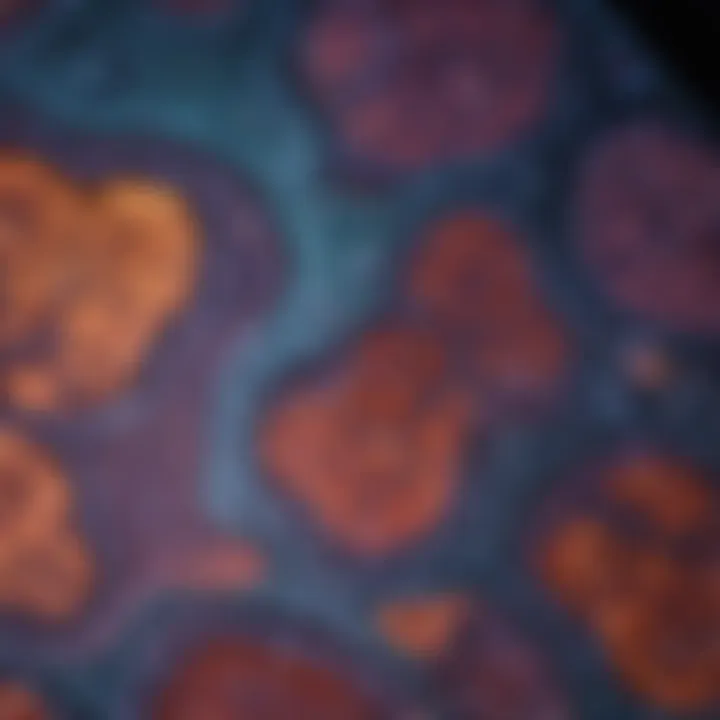
Intro
The realm of cellular biology is intricate, where countless processes at the molecular level govern life itself. One technique that shines a light on this complex world is membrane staining immunofluorescence. Rather than simply observing cells under a microscope, this method allows researchers to visualize specific components within the cell membranes, unraveling their roles and interactions. By labeling specific proteins or structures with fluorophores, scientists can elucidate cellular dynamics and behaviors that are often hidden from view.
Membrane staining immunofluorescence is not just a nifty trick; it’s a powerful tool that unveils crucial information about cellular structures and functions. Both unfathomable mysteries and minute details of biology become accessible through this technique. It bridges gaps in our understanding, making it essential for students, researchers, and professionals alike.
This discussion takes you through the essentials of this technique, including its underpinnings, various methodologies, common applications, and the exciting advancements shaping its future in research.
Key Concepts
Definition of the Main Idea
Membrane staining immunofluorescence is a specialized method for visualizing protein distributions and dynamics in living or fixed cells. It fundamentally relies on the principle of using specific antibodies labeled with fluorophores. When exposed to light of a certain wavelength, these fluorophores emit light, revealing the location of the target molecule. This makes detecting and affirming the presence of certain cellular components feasible, thus enabling a deeper understanding of cellular operations.
Overview of Scientific Principles
Several key principles underpin this technique:
- Fluorophores: These are fluorescent chemical compounds that absorb light at one wavelength and emit it at another. The choice of fluorophore can influence the success of staining, determining brightness and stability.
- Specific Binding: Antibodies used in immunofluorescence specifically bind to target antigens in cell membranes. This specificity is crucial for accurate visualization, ensuring that the signal detected relates only to the proteins of interest.
- Microscopy: After staining, specialized fluorescence microscopy is utilized to capture images. This equipment can distinguish signals from the background, offering clear imagery of cellular structures.
- Image Analysis: Post-capting images often require complex analysis to quantify the fluorescent signals and derive insights about cellular behavior.
Understanding these foundational concepts is key for both novice and seasoned players in the biological field, laying the groundwork for a myriad of applications.
Current Research Trends
Recent Studies and Findings
Various studies have indicated how membrane staining immunofluorescence plays its part in understanding disease mechanisms, particularly in cancer and neurodegenerative disorders. Recent research has increasingly focused on custom-developed antibodies, allowing for more targeted approaches in identifying proteins related to specific diseases. Some notable findings highlight novel roles for proteins previously thought to be unrelated to membrane dynamics, shifting paradigms in cellular biology.
Significant Breakthroughs in the Field
In recent years, advancements in imaging technology and fluorescence tagging have revolutionized the field. Noteworthy developments include:
- Super-resolution microscopy: This technique surpasses the diffraction limit of light, permitting visualization of structures at the molecular level with unprecedented clarity.
- Multicolor staining: Researchers are now able to track multiple targets within the same sample simultaneously. This has facilitated deeper insights into complex cellular interactions.
- Live-cell imaging: Techniques allowing for live analysis provide dynamic insights into cellular behavior and adaptability in real-time.
Given the rapid pace of progress, staying abreast of the latest advancements in membrane staining immunofluorescence is vital for anyone engaged in cellular biology.
Harnessing the power of membrane staining immunofluorescence not only enhances scientific understanding but also paves the way for groundbreaking therapeutic interventions.
Harnessing the power of membrane staining immunofluorescence not only enhances scientific understanding but also paves the way for groundbreaking therapeutic interventions.
Foreword to Membrane Staining
In the realm of cell biology, membrane staining immunofluorescence serves as a critical methodology for visualizing and understanding the intricate dynamics of cellular structures. This approach combines the specificity of antibodies with the striking visual efficacy of fluorescent labeling to reveal normally hidden cellular components. Knowing how these techniques work is an essential endeavor for students, researchers, and professionals alike, as it bridges theory and practice in the biological sciences.
Membrane staining is not merely about coloring cells; it’s about unlocking the secrets of cellular interactions and functions. With it, researchers can observe processes such as cell communication, membrane integrity, and signaling pathways, all of which are pivotal to comprehending health and disease states.
Defining Membrane Staining
Membrane staining refers to techniques aimed at highlighting cellular membranes using fluorescent markers. At its core, this process involves the binding of specific antibodies to target proteins located within or on the cell membrane. These antibodies are conjugated to fluorophores, allowing them to emit light under suitable excitation wavelengths. Essentially, it's like putting a glow stick on a hidden door to see the passage behind it.
In practical terms, membrane staining techniques can be categorized into various methods: direct and indirect staining. Each method has its own advantages regarding sensitivity and specificity. With direct staining, the antibodies bind straight to the antigens, providing straightforward results. Indirect staining amplifies the signal by using a secondary antibody, which can be useful when studying low-abundance proteins.
Importance in Cellular Biology
The significance of membrane staining in cellular biology cannot be overstated. This technique enables the visualization of structure-function relationships within cells, which is critical for understanding various biological processes. It assists in:
- Identifying Cellular Components: By labeling distinct proteins, scientists can observe where key molecules are located within the membrane context.
- Understanding Disease Mechanisms: For example, in cancer research, aberrations in membrane protein expression can be monitored, possibly leading to insights into tumor progression.
- Monitoring Cellular Dynamics: Membrane staining aids in tracking changes as cells respond to stimuli. This can be crucial in developmental biology or pharmacology, where cell behavior is constantly under scrutiny.
"Studying cells without membrane staining is like trying to read a book in the dark. You might see shapes, but the story will always remain obscured."
"Studying cells without membrane staining is like trying to read a book in the dark. You might see shapes, but the story will always remain obscured."
The ability to visualize membranes accurately allows researchers to craft hypotheses and design experiments with much clearer insight. Given the wavelength specificity that fluorescent techniques offer, researchers can even analyze multiple targets in the same sample, providing a more comprehensive view of the cell's landscape.
In summary, membrane staining immunofluorescence offers vital insights into cellular dynamics. Without it, our understanding of life at the cellular level would be severely compromised. As research and techniques advance, this area of study continues to evolve, promising even greater revelations about the workings of cells.
Principles of Immunofluorescence
Immunofluorescence is not just a fancy term thrown around in biological studies; it is the backbone of precise cellular visualization techniques. This section aims to peel back the layers of what makes immunofluorescence so crucial, outlining its core principles along with key benefits and sometimes overlooked considerations.
Fundamental Concepts
At its core, immunofluorescence hinges on the interaction between antigens and antibodies — those workhorse proteins that bind to specific cellular structures. When these antibodies are tagged with fluorophores, they emit light upon exposure to particular wavelengths. The system operates on the premise that by selectively binding a target antigen, one can visualize specific cellular structures under a fluorescence microscope.
It's akin to using a spotlight on a stage; while the rest of the theater may be darkened, the performer (the antigen) shines brightly because of the carefully placed lights (fluorescent antibodies). Here are a few essential concepts:
- Antibody-Antigen Interaction: The specificity of antibody binding allows for targeted staining, which is crucial for understanding complex cellular environments.
- Fluorescence Excitation and Emission: Knowledge of how fluorophores operate under different wavelengths can significantly enhance results and improve image quality.
- Signal Amplification: Secondary antibodies can be used to amplify the signal, enabling lower amounts of target proteins to be visualized.
Understanding these basic principles prepares researchers to employ immunofluorescence with greater finesse and efficacy.
Fluorescent Antibodies
The notion of fluorescent antibodies significantly contributes to the reliability and clarity of results in immunofluorescence studies. These antibodies, often conjugated with a variety of fluorophores, are designed to specifically bind to target proteins within the cell membranes. This specificity not only underscores the dynamic behavior of proteins in different cellular contexts but also deepens our understanding of cellular function.
Here are key takeaways regarding fluorescent antibodies:
- Types of Fluorescent Antibodies: There are various types, ranging from monoclonal antibodies that target a single epitope to polyclonal antibodies that can bind to multiple epitopes. Each has its own advantages depending on the research objective.
- Cross-Reactivity: It's essential to consider the potential for cross-reactivity, where antibodies might inadvertently bind to non-target proteins, leading to interpretations that miss the mark.
- Stability: The photostability of fluorophores can have a bearing on experimental outcomes. Some fluorophores fade rapidly, diminishing the clarity of results, while others maintain brightness over time, allowing for longer observation periods.
Incorporating fluorescent antibodies thoughtfully can turn the tide in research experiments, paving the way for insights that would otherwise remain hidden in the shadows.
"Immunofluorescence stands at the crossroads of basic biology and applied research, offering insights that advance both the scientific community and practical applications in medicine."
"Immunofluorescence stands at the crossroads of basic biology and applied research, offering insights that advance both the scientific community and practical applications in medicine."
Types of Membrane Staining Techniques
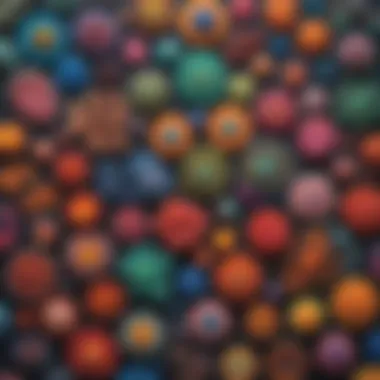
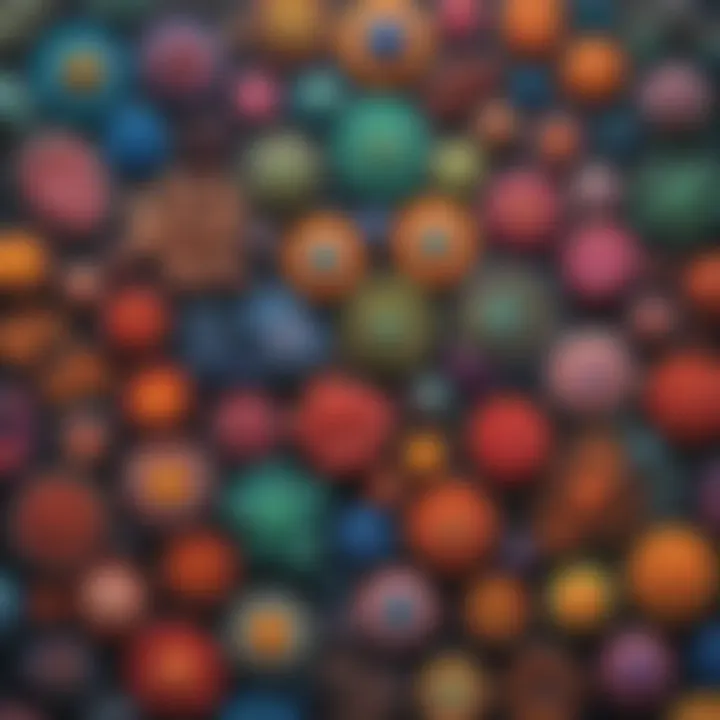
The realm of membrane staining techniques opens up a variety of avenues for researchers eager to understand the complexities of cellular dynamics. Each method presents its own set of advantages and implications, making the choice of technique a crucial first step in any immunofluorescence project. By understanding the differences between these methodologies, one can better tailor their approach to suit specific research needs and enhance the clarity of their visualizations.
Direct versus Indirect Staining
Direct staining is often praised for its simplicity. In this method, a fluorophore-conjugated antibody binds directly to the target antigen on the cell membrane, enabling immediate visualization. This approach can be quite effective, especially when the antigen of interest is abundant. The benefit here is clear – with fewer steps involved, there's less room for variability, and results can usually be obtained quite rapidly.
However, it's not all sunshine and roses. The major drawback is a lack of signal amplification. In cases where the target is low in abundance, the direct method might not provide sufficient intensity for visual identification.
On the other hand, indirect staining brings to the table a system of amplification that can drastically improve sensitivity. When using this method, a primary antibody binds to the target antigen, followed by a secondary antibody that is conjugated with a fluorophore. This secondary antibody attaches to the primary antibody, effectively multiplying the signal. As a result, indirectly stained samples can reveal proteins that might otherwise be missed with direct staining.
Yet, the added steps mean an increase in the complexity of the staining protocol. More procedures mean more opportunities for errors. Therefore, researchers must weigh the pros and cons carefully when deciding on their staining strategy.
Single vs. Multiple Labeling
The decision to utilize single or multiple labeling techniques is another pivotal consideration in membrane staining immunofluorescence. Single labeling allows for clear identification of a specific protein, making it relatively straightforward to analyze. This technique is especially beneficial in the initial stages of research when identifying the location of one protein is of utmost importance.
However, life within the cellular environment is not as simple as having one lone wolf roam about. Cells often interact with various proteins simultaneously, and understanding these interactions may require a broader view. This is where multiple labeling comes into play, enabling researchers to use several different fluorophores to label multiple antigens within a single sample.
With multiple labeling, one can glean insights not only about the presence of specific proteins but also how they may be co-localizing or interacting with each other. However, one must be cautious with this approach – too many labels can lead to spectral overlap, causing confusion and difficulties in interpretation. Interestingly, though modern imaging techniques are making it easier to differentiate between close-lying signals, researchers still must maintain an awareness of the risk involved in complex labeling schemes.
In summary, the choice between direct and indirect staining, as well as single versus multiple labeling, has significant implications for the outcomes of immunofluorescence experiments. Each technique brings its own set of strengths and weaknesses that necessitate careful consideration depending on the specifics of one's research objectives.
Selection of Fluorophores
Choosing the right fluorophore is a cornerstone in immunofluorescence staining, impacting both the clarity and accuracy of the results. With a variety of options available, the selection process must consider the specific requirements of each experiment. From the nature of the specimen to the type of microscopy being employed, the decision on which fluorophore to utilize can color the entire outcome.
Types of Fluorophores
Fluorophores are diverse in their chemical properties, and understanding these categories helps in making informed choices. Some of the commonly used types include:
- Organic Dyess: These are small molecules like fluorescein and rhodamine. They're appreciated for their broad availability, often yielding bright signals, but can suffer from photobleaching.
- Fluorescent Proteins: Examples are enhanced green fluorescent protein (EGFP) and mCherry, tags that can be genetically fused to proteins of interest, providing less interference in native biological processes.
- Quantum Dots: These semiconductor nanoparticles may offer unique spectral properties that allow multi-color experiments with excellent stability. However, their handling requires special consideration.
- Nanobodies: These small antigen-binding fragments can also carry fluorophores, providing high specificity and enhancing resolution in imaging.
Each type has its pros and cons shaped by various factors, including photostability, brightness, and their ability to be conjugated to antibodies efficiently. Consideration of these characteristics can make the difference between a successful imaging experiment and one that falls flat.
Considerations for Selection
When embarking on the journey to pick a fluorophore, several considerations come marching to the forefront:
- Spectral Properties: Selecting a fluorophore whose emission and excitation spectra align with the filters of your microscopy setup is crucial. Mismatching can lead to poor visibility and wasted efforts.
- Photostability: If your experiment requires prolonged observation, it’s wise to favor fluorophores that endure intense light exposure without fading.
- Compatibility: Take into account the antibody conjugation protocols and the availability of other reagents that might interact with the selected fluorophore.
- Multiplexing Capabilities: For analyses involving several targets, choosing fluorophores with distinct spectral profiles is imperative to avoid bleed-through interference.
- Biocompatibility: With a focus on live-cell imaging or in vivo studies, selecting non-toxic and easily removable fluorophores is essential to maintain cell health.
Key Point: Proper selection not only enhances visual clarity but also improves reproducibility and accuracy in data interpretation. The right choice can transform a good experiment into a great one.
Key Point: Proper selection not only enhances visual clarity but also improves reproducibility and accuracy in data interpretation. The right choice can transform a good experiment into a great one.
Taking all these factors into account, the task of selecting the optimal fluorophore becomes a balancing act between performance, feasibility, and the specific needs of the experimental setup. A well-considered fluorophore choice lays the groundwork for success in membrane staining immunofluorescence.
Preparation of Samples
In the realm of membrane staining immunofluorescence, the preparation of samples stands out as a pivotal step that defines the quality and reliability of subsequent analyses. Without adequate preparation, even the most sophisticated techniques may yield misleading or inconclusive results. Thus, proper sample preparation plays a crucial role in ensuring that cellular dynamics are accurately depicted during microscopy.
Cell Culture Techniques
To begin with, understanding the intricacies of cell culture techniques is fundamental for achieving optimal results. Cell culture serves as the groundwork upon which experiments are built; it allows researchers to manipulate and observe living cells under controlled conditions. Here are some key points to consider:
- Choosing the Right Medium: The selection of an appropriate culture medium is intimately tied to the type of cells being utilized. Different cell lines have unique nutrient requirements. For instance, HeLa cells thrive in Dulbecco's Modified Eagle Medium (DMEM) enriched with fetal bovine serum (FBS), while other lines may prefer different formulations.
- Subculturing Protocols: Routine subculturing must be conducted to maintain cell health and viability. This often involves detaching cells, which can be performed via trypsinization or enzymatic treatments. However, care must be taken to avoid over-trypsinization, as it can cause cellular damage.
- Contamination Control: Keeping a sterile environment is essential. Aseptic techniques should be employed to prevent bacterial or fungal contamination. Contamination can derail an experiment's validity, leading to erroneous conclusions.
- Monitoring Growth: Regularly check cell morphology and growth patterns under the microscope. This helps to assess overall health and suitability for staining protocols.
These techniques are crucial because they lay the foundation for reliable immunofluorescence outcomes. When cells are properly cultured, they resemble in vivo conditions more closely, thus providing meaningful insights into cellular dynamics.
Fixation and Permeabilization
Fixation and permeabilization are critical processes that prepare biological specimens for staining. Each step guards the integrity of the cells while also allowing access to cellular structures for antibody penetration.
- Fixation: This step involves applying a fixative agent to stabilize cellular components and prevent degradation. Common fixatives include paraformaldehyde or methanol, each offering different advantages. For instance, paraformaldehyde maintains protein structure better, which is essential for antibody binding.
- Permeabilization: Once fixation is complete, permeabilization enables antibodies to infiltrate cell membranes. For this, detergents like Triton X-100 or saponin are commonly used. The right choice of detergent depends on the specific cellular structures being targeted and the fixation method employed. Missteps here could hamper antibody access, leading to gaps in data.
- Timing and Concentration: The duration of fixation and the concentration of permeabilizing agents must be optimized. Over-fixation can mask antigens, and under-fixation may fail to preserve the cell's architecture. In practice, small test batches can be pivotal in fine-tuning these parameters.
Effective fixation and permeabilization enable clearer imaging and more accurate interpretation of cellular dynamics. If these steps are skimped, results can be as good as throwing darts blindfolded, with low chances of hitting the mark.
"The devil is in the details"; ensuring meticulous sample preparation guarantees that the resulting data is both robust and reliable.
"The devil is in the details"; ensuring meticulous sample preparation guarantees that the resulting data is both robust and reliable.
Conducting Immunofluorescence Staining
Understanding the practical aspects of conducting immunofluorescence staining is crucial for any researcher venturing into cellular biology. This section dives into the methodologies surrounding the protocols employed in staining, as well as the incubation conditions that influence the efficacy of the staining process. Successfully executing these steps not only ensures reliable results but also enhances the overall quality of the research findings.
Staining Protocols
Membrane staining is a meticulous chore that requires careful consideration of the techniques involved. Each protocol can vary widely based on the type of sample and the specific fluorophore being used. A basic staining protocol encompasses several key steps:
- Preparation: Begin with appropriate sample preparation, which sets the foundation for effective staining. This usually involves obtaining live or fixed cells, following strict aseptic procedures to prevent contamination.
- Blocking: To minimize non-specific binding of antibodies, inclusion of a blocking step is paramount. Agents like serum or BSA are commonly employed to saturate any potential binding sites on the membrane.
- Primary Antibody Incubation: This step involves incubating the sample with a primary antibody specific to the target membrane protein. Temperature and duration are crucial factors here; typically, 1-2 hours at room temperature or overnight at 4°C often provides optimal results.
- Washing: After primary antibody binding, thorough washing reduces background staining and improves signal clarity. Using buffers like PBS may help in effective rinsing.
- Secondary Antibody Application: Apply a secondary antibody that is conjugated with a fluorophore, which binds to the primary antibody. This step amplifies the signal.
Careful adherence to these protocols can significantly improve the integrity and reliability of your immunofluorescence results. A useful approach is to keep a detailed lab notebook documenting all variations, as this can guide future experiments.
Incubation Conditions
The incubation phase is often where the success or failure of the immunofluorescence technique hinges. Underestimating the significance of environmental conditions can lead to disappointing outcomes in an otherwise well-planned experiment. Here are some factors to consider:
- Temperature: The temperature at which samples are incubated can influence antibody binding affinity. Lower temperatures tend to reduce non-specific interactions but may also slow down the binding kinetics. In contrast, higher temperatures may enhance binding but could also increase background fluorescence. Hence, choosing the right temperature is a delicate balancing act.
- Time: The duration of incubation is just as critical. Too short a time may yield weak fluorescence, while prolonged exposure can lead to increased background noise due to non-specific binding. It's often beneficial to perform a time-course experiment to determine the optimal incubation time for the specific antibodies in use.
- Media Types: The choice of buffer or media used during incubation influences the staining results as well. Certain buffers can help maintain the integrity of the cellular structure, which is essential for accurate imaging. Opting for media that include stabilizing agents can sometimes yield better fluorescence signals.
In summary, the processes around conducting immunofluorescence staining encompass a blend of science and art. Each aspect requires deliberate attention to detail and should not be taken lightly. A well-executed staining protocol combined with optimal incubation conditions can lead to significant breakthroughs in cellular research.
"The quality of your immunofluorescence is only as good as the protocols you follow. Rushing through the protocol can diminish your results."
"The quality of your immunofluorescence is only as good as the protocols you follow. Rushing through the protocol can diminish your results."
These protocols and conditions are not merely arbitrary; they reflect years of refinement and are essential for advancing our insight into cellular dynamics.
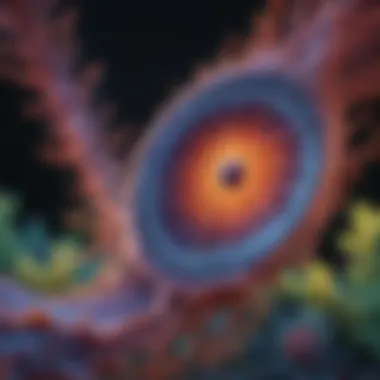
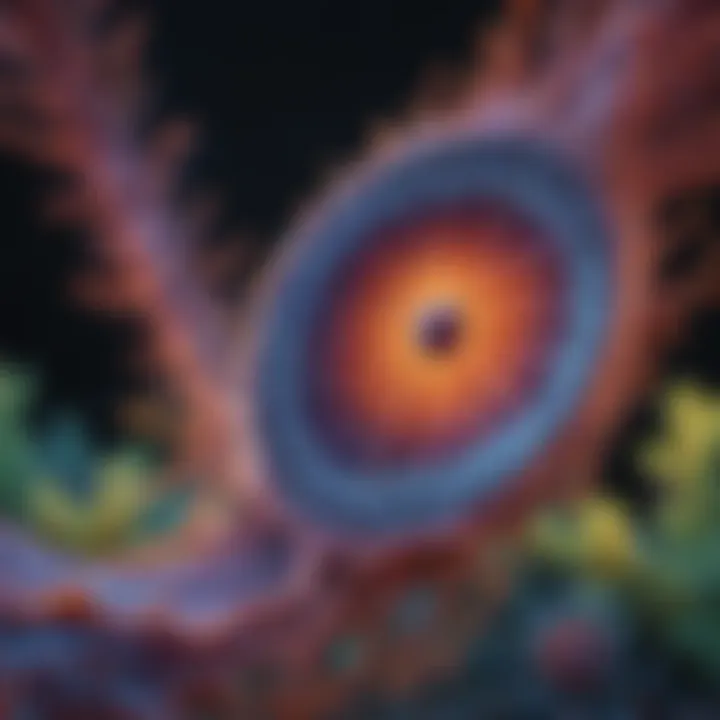
Imaging Techniques
The realm of membrane staining immunofluorescence is largely defined by the imaging techniques employed to visualize and analyze cellular structures. Without effective imaging, even the most sophisticated staining protocols render little value. The quality and type of imaging chosen can affect the overall insight gained from the experiment.
Imaging techniques provide the key to unlocking data about cellular dynamics. Through high-resolution visualization, researchers can discern not just the presence of particular proteins but also their localization within the context of cellular architectures. This segregation can sometimes be the difference between understanding a disease process and remaining in the dark about critical pathways.
Microscopy Methods
Microscopy serves as the backbone of imaging in immunofluorescence. Multiple types of microscopes come into play, each with its unique merits. For instance, the confocal microscope is often lauded for its ability to attain higher axial and lateral resolution compared to traditional widefield microscopes. This method employs a scanning mechanism that permits optical sectioning, thus minimizing background signal and enhancing clarity.
Another viable option is the multi-photon microscope, which excels in imaging deeper tissues. It operates by utilizing longer wavelengths of light, which helps in penetrating dense specimens.
Here's a quick breakdown of popular microscopy methods used in membrane staining:
- Confocal Microscopy: Provides high-resolution images and allows for 3D reconstruction.
- Multi-Photon Microscopy: Excellent for deep tissue imaging with reduced photodamage.
- Super-Resolution Microscopy: Offers even greater resolution than confocal, enabling the visualization of structures only nanometers apart.
Selecting the right microscopy method often hinges on the specific cellular dynamics under investigation. Each method presents unique benefits, but also comes with its own set of challenges.
"The quality of imaging techniques directly influences the interpretation of results in immunofluorescence studies."
"The quality of imaging techniques directly influences the interpretation of results in immunofluorescence studies."
Image Acquisition and Processing
Next comes image acquisition and processing, a critical phase where theoretical knowledge meets practical application. Capturing the fluorescence images accurately is crucial as any misstep can distort the outcome of the whole experiment. During this step, setting the right exposure times and gain levels determines the quality of data you will eventually analyze.
Post-acquisition, image processing techniques are essential for extracting meaningful information from your raw images. Tools such as deconvolution can significantly enhance image clarity and resolution by removing out-of-focus light. Furthermore, software like ImageJ allows for quantitative analysis and custom measurements to be made, offering a more nuanced understanding of the cellular dynamics at play.
Key steps in the image acquisition and processing workflow include:
- Calibration: Ensuring the microscope is calibrated for the fluorophores used.
- Optimal Settings: Adjusting gain and exposure settings for the best image quality without saturation.
- Deconvolution: Utilizing advanced algorithms to improve the clarity of images.
- Normalization: Tracking intensity variations across images to keep results consistent.
Ultimately, the synergy between effective microscopy methods and sophisticated image acquisition and processing not only elevates the quality of data collected but also enhances the ability to interpret complex cellular interactions.
Data Interpretation
Understanding and interpreting the data from membrane staining immunofluorescence is crucial for a plethora of reasons. First off, it provides a window into the complex realm of cellular processes. When researchers stain cell membranes with fluorescent markers, they expect to see certain patterns that can reveal much about cellular dynamics, such as protein localization, membrane integrity, or even interactions between different cellular components. The interpretation of these patterns isn’t trivial; it is a multi-layered process that demands both technical know-how and a solid grasp of underlying biological principles.
Analyzing Fluorescence Patterns
When scientists analyze fluorescence patterns, they’re not just looking at colors or intensity levels; they’re delving into a narrative that the cells are eager to tell. Each stain can highlight specific proteins or structures, and the resulting images can demonstrate various cellular states or responses. For instance, a high intensity around a membrane might signify active receptor clustering, while diffuse staining could indicate a different signaling modality. Therefore, careful examination is paramount.
Some vital considerations for analyzing these patterns include:
- Specificity of Antibodies: It’s paramount to ensure that the chosen antibodies specifically bind to their intended targets. Cross-reactivity can generate misleading patterns.
- Background Noise: Background fluorescence must be accounted for, as it can obscure true signals and cause misinterpretations.
- Channel Overlap: When utilizing multiple fluorophores, it's vital to ensure that the emission spectra do not overlap in ways that could confuse the readings.
To visualize these considerations, one can examine images side-by-side – an image with clear membrane boundaries versus one filled with artifacts. The differences can tell you a lot about the reliability of your initial interpretations.
Quantification of Results
Quantifying the results of immunofluorescence staining takes interpretation a step further. While qualitative observations provide insights into the presence or absence of particular proteins, quantification helps calibrate those observations against numerical values. This adds a measurable dimension to data interpretation, allowing for comparisons across experiments.
Common methodologies for quantification include:
- Fluorescence Intensity Measurement: Utilizing software to measure intensity can help quantify protein expression levels in different conditions.
- Image Analysis Software: Programs like ImageJ or Fiji can simplify this task by allowing users to define regions of interest and compute statistics on fluorescence.
- Comparative Analysis: Comparing fluorescence between treated and untreated samples gives a perspective on the effect of a treatment or intervention.
In practice, quantification should be approached with caution. Factors such as variability in sample preparation and staining efficiency can heavily influence results. Therefore, having adequate control samples is key to ascertain that the quantification reflects true biological states and not experimental artifacts.
"In the world of cellular immunofluorescence, every pixel has a story to tell, and understanding that narrative is essential for advancing our grasp of cellular mechanics."
"In the world of cellular immunofluorescence, every pixel has a story to tell, and understanding that narrative is essential for advancing our grasp of cellular mechanics."
Effective data interpretation, therefore, emerges as a process requiring critical thinking, attention to detail, and a firm grounding in experimental biology. Through careful analysis of fluorescence patterns and subsequent quantification, researchers can not only confirm their hypotheses but also pave the way for new discoveries.
Common Applications
Membrane staining immunofluorescence serves as a cornerstone in various fields of biological research, shedding light on the intricate behaviors of cells in diverse contexts. Understanding its applications not only enhances our grasp of cellular dynamics but also illuminates the pathways leading to breakthroughs in medicine, developmental biology, and beyond. Here, we explore the synergy between membrane staining techniques and their practical applications, highlighting their essential role in advancing scientific knowledge.
Pathological Studies
In the realm of pathology, membrane staining immunofluorescence provides a lens through which we can scrutinize the abnormal behaviors of cells. This technique is particularly valuable in diagnosing diseases such as cancer. By utilizing specific antibodies that target proteins overexpressed in tumorous cells, researchers can visualize the presence and localization of these markers within tissue samples. For instance, observing heightened expression of HER2 in breast cancer can guide therapeutic decisions, steering patients towards targeted treatments.
Moreover, membrane staining helps identify changes in cellular morphology associated with diseases. Consider neurodegenerative disorders, where altered membrane compositions and protein aggregates shed light on disease mechanisms. Visualizing these alterations can be pivotal in understanding how these conditions progress and may inform therapeutic strategies.
Developmental Biology
Developmental biology thrives on the insights gained through membrane staining immunofluorescence. Tracking the formation and differentiation of cells during embryonic development is crucial for understanding how complex organisms form from a single cell. Using specific fluorophores to tag cell surface proteins enables researchers to observe cell migration and interaction in real-time.
For example, in studies of neural development, staining can reveal patterns of neuron migration and synaptogenesis. This information uncovers the subtle orchestration of cellular events that sculpt developing tissues. By understanding these processes, scientists can gain insights into congenital anomalies and potential interventions.
Drug Discovery
The drug discovery process heavily relies on membrane staining immunofluorescence to evaluate the effects of potential therapeutic compounds on cellular behaviors. In high-throughput screening, for instance, researchers can assess how drug candidates alter the distribution of specific membrane proteins. This application is instrumental in identifying new targets for therapeutic intervention.
Furthermore, observing dose-dependent responses through fluorescence can facilitate the understanding of drug mechanisms. For example, if a compound inhibits the uptake of a fluorescently-labeled glucose analog, it suggests a target interaction that cripples cellular metabolism—valuable information in the context of cancer therapies.
In summary, the applications of membrane staining immunofluorescence extend far beyond mere visualization. They encompass a vital toolkit in pathological studies, developmental biology, and drug discovery, ultimately weaving a complex narrative that enhances our understanding of cellular dynamics. As advancements in technology unfold, the potential for new applications continues to grow, broadening the horizons for both research and clinical practice.
Limitations of Membrane Staining Immunofluorescence
Membrane staining immunofluorescence plays a pivotal role in cellular biology, yet it’s not without its limitations. Understanding these constraints is critical for researchers and practitioners to accurately interpret results and design robust experiments. Recognizing the hurdles one may encounter can lead to better methodologies and ultimately, more significant findings.
Technical Challenges
One of the foremost struggles in membrane staining is maintaining the integrity of the samples during preparation. Sample fixation—a necessary step to preserve cellular structures—can sometimes lead to artifacts. These artifacts might confound results, especially in delicate or dynamic systems. A further complication arises from the need for optimal permeabilization. If cells aren't adequately permeabilized, the antibodies may not gain sufficient access to intracellular targets. On the flip side, over-permeabilization can dismantle structural properties that are essential for accurate imaging and analysis.
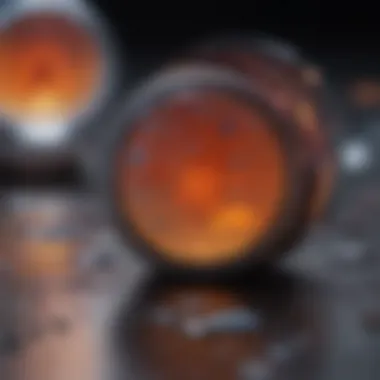
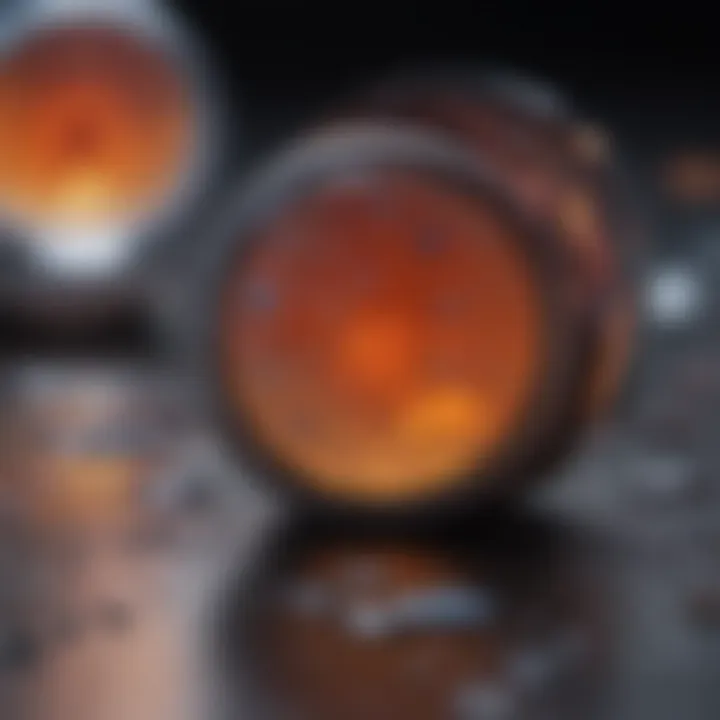
Furthermore, the choice of antibodies presents its own set of challenges. Antibodies can vary significantly in their specificity and sensitivity. A highly specific antibody may yield beautiful staining on a well-preserved sample, while that same antibody can provide confusing results if the sample's fixation isn't spot on, or if non-specific binding occurs. In this context, researchers must often engage in tedious optimization processes. Achieving reliable and reproducible staining can feel like looking for a needle in a haystack, particularly when working with rare cell types or conditions that lack standardized protocols.
Another notable technical hurdle is illumination intensity and photobleaching of the fluorophores, an issue that can severely affect the quality of imaging. Over time, as light hits the fluorescent dye, it can lose its ability to fluoresce. Photobleaching can distort the acquired data, necessitating timely imaging and specific light conditions to mitigate this degradation.
Interpretation Pitfalls
When it comes to interpreting the data gathered from membrane staining techniques, context is everything. Readers—be they researchers or academics—must tread carefully, as misinterpretations can lead to misconceived conclusions. One common pitfall is the overreliance on visual data without sufficient quantitative analysis. While striking fluorescence images can be captivating, they do not always provide a comprehensive understanding of cellular dynamics. This pitfall is particularly evident when differences between control and experimental groups are subtle.
Moreover, researchers might fall into the trap of believing that bright staining equals high expression levels. This assumption can be misleading, as it doesn't account for potential signal saturation or differences in antibody affinity. Additionally, different cell types may express varying levels of the same membrane protein, further complicating interpretations.
The importance of rigor in comparing fluorescence intensity across different samples cannot be overstated. Misjudgments here can lead to flawed hypotheses and wasted resources.
The importance of rigor in comparing fluorescence intensity across different samples cannot be overstated. Misjudgments here can lead to flawed hypotheses and wasted resources.
Lastly, the complexity of cellular environments cannot be overlooked. Cells are not isolated systems; they exist in dynamic contexts, influenced by signaling pathways and extracellular factors. This complexity means that even well-designed experiments can yield ambiguous results that require careful analysis. Therefore, drawing definitive conclusions solely based on membrane staining results, without incorporating complementary methodologies or controls, can lead researchers down the wrong path.
Acknowledging these limitations is vital for progress in the field. By understanding these challenges and pitfalls, researchers can better navigate the vast landscape of immunofluorescence, ultimately leading to more reliable and impactful findings. Recognition of these hurdles also paves the way for innovations and improvements within staining methodologies.
Future Directions in Immunofluorescence
As the field of cellular biology evolves, so too does the methodology surrounding membrane staining immunofluorescence. This section highlights the importance of keeping up with the core advancements shaping future practices in this essential technique. Scientists are increasingly using these innovations not only to enhance the visualization of cellular structures but also to deepen their understanding of complex biological processes. By examining emerging techniques and the integration of immunofluorescence with other modalities, researchers can enhance their approaches to studying cellular dynamics.
Emerging Techniques
The landscape of immunofluorescence is indeed shifting as new technologies and materials come into play. One notable trend is the development of next-generation fluorescent probes. These probes are designed to provide higher photostability, extensible spectral ranges, or a broader variety of labels. For researchers, this means that experiments can often achieve clear and detailed imaging without the frequent photobleaching that characterizes traditional fluorophores. A few emerging techniques include:
- Super-resolution Microscopy: This innovative approach allows researchers to visualize structures at the nanometer level, which was previously deemed unattainable with conventional microscopy.
- Quantum Dots: These semiconductor nanocrystals offer unique optical properties, leading to brighter and more stable fluorescence compared to standard dyes. Their tunable emission spectra could pave the way for multiplexing studies.
- Optogenetics: This technique harnesses light to control cells within living tissues. By integrating optogenetics with immunofluorescence, scientists can explore real-time cellular responses with unprecedented accuracy.
Immersing oneself in these emerging techniques provides avenues for more complex experiments and the potential to uncover biological mysteries that have long been overlooked.
Integration with Other Modalities
Merging immunofluorescence with other imaging and analytical methods marks a significant advance in biological research, enhancing the depth and breadth of investigations. The integration of techniques allows for a more holistic understanding of cellular dynamics, leading to discoveries that standalone methods might not reveal. Some compelling integrations include:
- Mass Cytometry: This technique combines immunofluorescence with mass spectrometry, enabling the simultaneous quantification of numerous parameters at a single-cell level with minimal spillover. It represents a leap forward in understanding heterogeneous cell populations.
- Live-cell Imaging: Coupling immunofluorescence techniques with live-cell imaging enables the capturing of dynamic processes within cells as they occur. This can reveal vital interactions and behaviors that provide insight into cellular mechanisms in real time.
- Single-cell RNA Sequencing: The combination of immunofluorescence and transcriptomic profiling offers a powerful approach to correlate protein expression with gene expression at the single-cell level, which could lead to groundbreaking insights into cellular function and heterogeneity.
The future of immunofluorescence lies in embracing these integrations, which expand the capability to study complex cellular processes in more informative contexts.
The future of immunofluorescence lies in embracing these integrations, which expand the capability to study complex cellular processes in more informative contexts.
Epilogue
The conclusion wraps up the discussion on membrane staining immunofluorescence by emphasizing its crucial role in advancing our understanding of cellular dynamics. The ability to visualize cellular structures accurately is not just a nice-to-have; it is a linchpin in the world of cell biology and molecular research. By employing techniques that illuminate membrane components, researchers can uncover intricate details about cellular processes that were previously obscured.
Summary of Key Points
Throughout this article, various aspects of membrane staining immunofluorescence have been dissected. Key points include:
- Definition and Importance: Membrane staining serves as a fundamental technique for monitoring cellular behavior and function.
- Principles of Immunofluorescence: The basic concepts behind fluorescent tagging have been elucidated, showcasing how precise labeling can produce vivid imagery.
- Diverse Techniques: Various methodologies—such as direct and indirect staining—provide flexibility depending on research needs. Single and multiple labeling strategies enable nuanced investigations into cellular interactions.
- Selection of Fluorophores: The choice of fluorophores is not merely a matter of personal preference; it greatly influences the quality of results and subsequent analyses.
- Applications in Research: From developmental studies to drug discovery, membrane staining is instrumental in a variety of scientific inquiries.
- Limitations and Challenges: Understanding the hurdles associated with this technique prepares researchers for potential obstacles in their investigations.
- Future Directions: The landscape of immunofluorescence is evolving, with groundbreaking techniques and interdisciplinary approaches paving the way for novel insights.
Implications for Future Research
Looking forward, the implications of this technique are vast. For students and seasoned researchers alike, continued investigations leveraging membrane staining immunofluorescence can open new avenues in cellular biology. Emerging technologies such as super-resolution microscopy and image analysis software are enhancing the resolution and interpretability of fluorescence data.
Strategies for integration with other modalities—like live-cell imaging and single-cell RNA sequencing—will likely yield a more holistic view of cellular behavior. Furthermore, collaborations across disciplines may foster innovations that further refine staining techniques or develop entirely new ones.
In summary, the future of membrane staining immunofluorescence not only holds the promise of more refined observations but also hints at a transformative impact on the life sciences as a whole. The journey of scientific discovery continues to depend on the tools we employ today, and mastering techniques like these will be essential for addressing the complex questions of tomorrow.
Importance of References
In this context, references provide multiple benefits:
- Credibility: They establish the work as well-researched and founded on solid ground. Mentioning studies conducted in established labs or published in peer-reviewed journals elevates the article's trustworthiness.
- Context: References help situate the discussion within broader scientific discourse. For example, citing seminal papers that first defined immunofluorescence sets the stage for understanding how methodologies have evolved.
- Resources for Further Study: Providing readers with targeted references enables them to explore the topic with depth, fostering a richer understanding of the complexities involved in membrane staining techniques.
Considerations for Selecting References
Not all references are created equal; the selection of sources requires thoughtfulness. Here’s what to keep in mind:
- Relevance: It’s not just about quantity; it’s essential that each reference be directly applicable to the topic at hand.
- Recency: Research in cellular biology is ever-evolving. Citing newer studies can reflect the latest advancements and innovations.
- Diversity of Sources: Utilizing a mix of articles, textbooks, and online resources helps provide a more rounded perspective. For instance, referencing both experimental results and theoretical frameworks can enrich the narrative.
"A referencing strategy that includes a variety of perspectives helps cultivate a more informed discussion, paving the way for potential future research."
"A referencing strategy that includes a variety of perspectives helps cultivate a more informed discussion, paving the way for potential future research."
End
Thus, references are not merely footnotes. They are critical pathways through which knowledge is shared and validated, encouraging readers to venture deeper into the study of membrane staining immunofluorescence. These citations illuminate the text, offering a comprehensive guide that reflects the ever-expanding world of cellular dynamics. Proper use of references cements the author's position as a thoughtful commentator within the scientific community, fostering greater understanding and innovation.
Acknowledgments
In any academic or scientific endeavor, it’s crucial to recognize the contributions of those whose insights, hard work, and support made the project possible. For this article, the need for acknowledgment is particularly significant. This ensures that we honor individuals, teams, and institutions whose efforts facilitated the understanding of membrane staining immunofluorescence and its implications in cellular dynamics.
Recognizing Contributions
Membrane staining immunofluorescence doesn't exist in a vacuum. There are countless researchers and professionals. Every experiment, publication, and mentorship ultimately shapes the knowledge pool.
- Researchers: Their pioneering work lays the groundwork for current techniques. Without their findings, the methodologies discussed here would lack depth.
- Institutions: University laboratories and research facilities are often the breeding grounds of innovation. Their commitment to advancing the field fosters collaboration and idea exchange.
- Funders and Sponsors: Financial backing from grants or organizations can propel research efforts. It allows scientists to explore and experiment, which are vital for progress in understanding cellular processes.
Benefits of Acknowledgments
Acknowledging contributions serves several important purposes:
- Encouragement: It boosts morale and encourages continued academic work. Knowing one’s efforts are valued can be motivating for researchers.
- Transparency: It promotes honesty by demonstrating the collaborative nature of scientific discovery. Recognizing help maintains integrity and fosters trust within the scientific community.
- Networking Opportunities: Highlighting individuals or groups provides pathways for future collaborations. Connections made during the research process can lead to exciting advancements.
Considerations in Acknowledgments
While it’s essential to acknowledge contributions, it’s equally important to do it thoughtfully. Over or under-acknowledgment can create misunderstandings.
- Inclusivity: Care should be taken not to overlook anyone. Proper recognition enhances inclusivity.
- Balance: Striking a balance in the length and detail of acknowledgments is key. Brief but comprehensive tributes are generally preferred.
Overall, acknowledgments in scientific articles reflect both gratitude and professionalism. They remind readers that knowledge in the field of membrane staining immunofluorescence is built upon the shoulders of many committed individuals. This ongoing collaborative effort is crucial for future breakthroughs, providing a solid foundation for more discoveries in cellular dynamics.