Quantum Dot Imaging: A Comprehensive Exploration
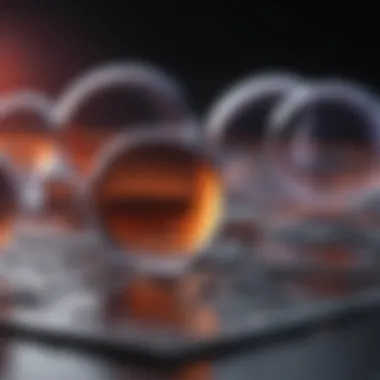
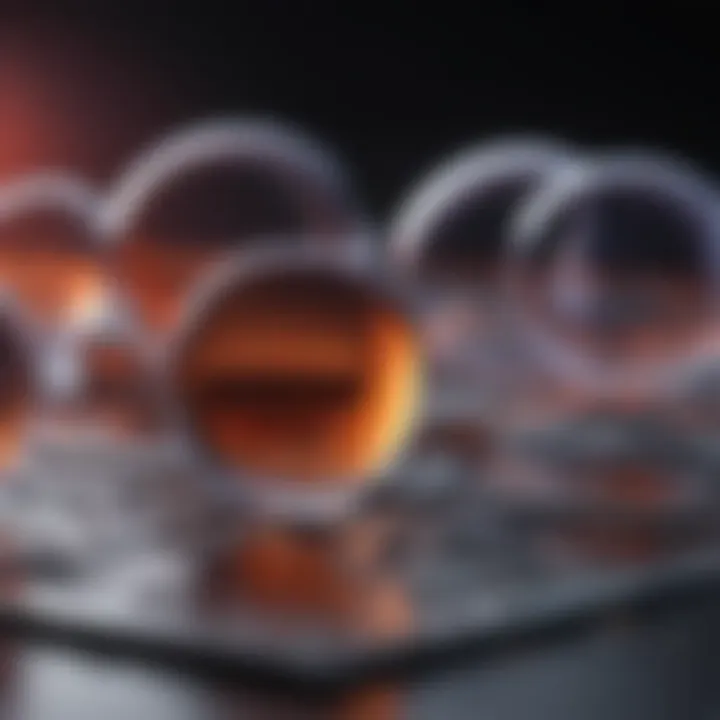
Intro
Quantum dot imaging has emerged as a revolutionary technique in the field of biomedical research and diagnostics. This technology harnesses the unique properties of quantum dots—nanoscale semiconductor particles that exhibit remarkable photoluminescence. Due to their size, quantum dots can emit light at specific wavelengths, which makes them invaluable for imaging biological systems. In this article, we will explore the essential concepts, current research trends, and potential future applications of quantum dot imaging.
Key Concepts
Definition of the Main Idea
Quantum dot imaging refers to the use of quantum dots for visualizing biological structures at the cellular and molecular levels. These nanoparticles are typically composed of heavy metals, such as cadmium or lead sulfide, and are coated with a protective shell. They can be engineered to emit specific colors of light upon excitation, enabling researchers to discriminate between different types of cells and tissues.
Overview of Scientific Principles
The function of quantum dots relies on the principles of quantum mechanics. When light is absorbed by a quantum dot, electrons are excited and move to a higher energy state. As the electrons return to their ground state, energy is released in the form of light. The wavelength of this emitted light, and therefore its color, is determined by the size of the quantum dot. Smaller dots emit blue light, while larger dots emit red light. This tunability is a key advantage in imaging applications, allowing investigators to utilize multiple dots simultaneously for multi-color imaging.
Current Research Trends
Recent Studies and Findings
Recent studies have expanded the knowledge base surrounding quantum dot imaging. Innovations in surface chemistry have enhanced the stability and biocompatibility of these nanoparticles. Researchers have shown that functionalizing quantum dots with biomolecules such as antibodies increases their specificity for target cells. Moreover, new imaging techniques have been developed to incorporate quantum dots into live-cell imaging, providing deeper insights into cellular processes.
Significant Breakthroughs in the Field
Several breakthroughs have marked the evolution of quantum dot imaging. For instance, a 2023 study published in Nature Communications detailed a method for using quantum dots to visualize the progression of tumors in real time. This platform allows for better understanding of tumor biology and can aid in treatment decisions. Such developments not only demonstrate the promising applications of quantum dot imaging but also underscore the importance of ongoing research in this domain.
"Quantum dots represent a significant leap in our ability to visualize biological systems at an unprecedented resolution."
"Quantum dots represent a significant leap in our ability to visualize biological systems at an unprecedented resolution."
Finale
The exploration of quantum dot imaging reveals its vast potential in biological sciences. As research continues to advance, we can anticipate more sophisticated and versatile applications of this technology. The integration of quantum dot imaging into routine clinical practices could substantially enhance diagnostic precision and treatment efficacy. By understanding the underlying principles and engaging with current trends, we foster an environment of innovation and discovery.
Understanding Quantum Dots
Quantum dots are significant in the field of imaging technologies. They serve as a bridge between fundamental physics and practical applications in various disciplines, particularly in biological imaging. Understanding quantum dots is critical, as these nanoscale semiconductor particles exhibit unique optical properties that can be exploited for enhanced imaging contrast and resolution. The discussion around quantum dots includes key elements such as their definition, composition, synthesis techniques, and the contrasting advantages they have over traditional fluorescent labels.
Definition and Composition
Quantum dots are semiconductor nanocrystals that range in size from 2 to 10 nanometers. Their size and material composition allow them to have size-dependent optical and electronic properties. This means that the emission color of a quantum dot can be tuned by simply changing its size, a feature that renders them highly versatile. They are typically composed of materials like cadmium selenide (CdSe) or lead sulfide (PbS). The core of the quantum dot can be surrounded by a shell of another semiconductor, enhancing its stability and quantum yield. By integrating various elements, researchers can create quantum dots with tailored properties to meet specific needs in imaging applications.
Synthesis Techniques
Colloidal synthesis
Colloidal synthesis is a method that involves the chemical reaction of precursors in a solution to form quantum dots. This technique is advantageous because it allows for better control over size, shape, and uniformity of the resulting particles. The main characteristic of colloidal synthesis is its ability to produce a high yield of quantum dots with narrow size distribution, which is beneficial for imaging applications. However, there are limitations, such as potential impurities in the products that may affect their optical properties.
Vapor deposition methods
Vapor deposition methods refer to techniques where vaporized material condenses on a substrate to form quantum dots. This synthesis process is well-regarded for producing high-purity quantum dots. One of the key features of vapor deposition methods is their scalability, allowing for the production of larger quantities suitable for practical applications. Nonetheless, these methods can be more complex and may require advanced vacuum systems, making them less accessible for some research environments.
Solid-state synthesis
Solid-state synthesis involves the reaction of solid ingredients to create quantum dots. This method is characterized by its simplicity and cost-effectiveness. The unique feature of solid-state synthesis is that it does not require solvents, minimizing environmental concerns. However, this method often results in a broader size distribution of dots compared to colloidal synthesis, potentially affecting the consistency of imaging results.
Quantum Dots vs. Traditional Fluorescent Labels
Compared to traditional fluorescent labels, quantum dots offer several notable advantages. Traditional labels often suffer from issues like photobleaching, where the fluorescent signal fades over time. In contrast, quantum dots exhibit greater photostability and a brighter signal, which makes them more suitable for long-term imaging studies. Furthermore, while traditional fluorescent labels are limited to specific wavelengths, quantum dots can emit different colors based on their size, providing a more versatile option for multiplexing in imaging applications.
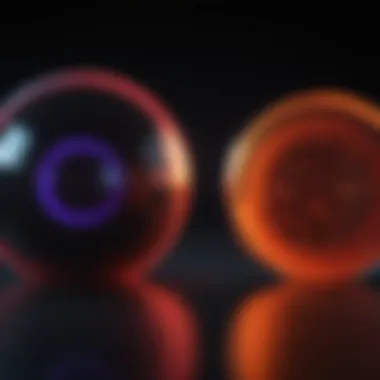
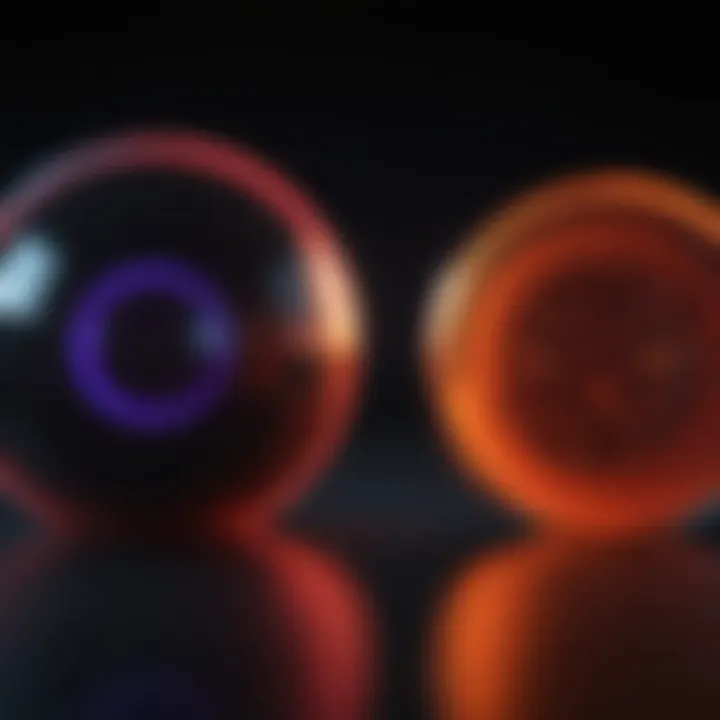
Quantum dots represent a paradigm shift in imaging technologies due to their unique properties.
Quantum dots represent a paradigm shift in imaging technologies due to their unique properties.
Principles of Quantum Dot Imaging
Quantum dot imaging represents a pivotal advancement in biomedical visualization. Understanding the principles that govern quantum dot behavior enhances the application of these nanoparticles in various imaging techniques. This section will outline the key optical properties and imaging mechanisms that create the foundation of quantum dot imaging. These elements are crucial for exploiting the unique capabilities of quantum dots in research and clinical settings.
Optical Properties of Quantum Dots
The optical properties of quantum dots are fundamentally what contribute to their effectiveness in imaging applications. Their ability to emit light in response to excitation is shaped largely by their size and composition, allowing for tailored applications in different contexts.
Photoluminescence
Photoluminescence is one of the most critical characteristics of quantum dots. This process involves the absorption of photons, followed by the re-emission of light. Its contributions to imaging are significant since it allows for high-resolution visualization of biological specimens. The key characteristic of photoluminescence is its strong emission and narrow spectral profile, which enables distinguishing between closely located quantum dots.
This property is considered beneficial because of how it enhances signal clarity in various imaging setups. A unique feature of photoluminescence is its tunable nature according to the size of the quantum dots, allowing researchers to select specific wavelengths for targeted imaging. However, photoluminescence may have disadvantages, such as photobleaching, where prolonged exposure to light can lead to the fading of the signal over time.
Size-dependent emission
Size-dependent emission refers to the phenomenon where the emitted light color changes based on the size of the quantum dots. Smaller dots emit blue light, while larger dots emit red light. This aspect is pivotal for multiplex imaging, where different sizes can be used to track multiple targets simultaneously. The benefit of this property is its capacity for precise spectral control, enabling researchers to identify various biomolecules in a sample without overlap.
The unique feature lies in how size manipulation allows researchers to engineer desired emission properties for specific applications. However, the disadvantages focus on the complexity of synthesizing dots with uniform sizes, which is necessary for reproducible results.
Quantum yield
Quantum yield measures the efficiency of photoluminescence, indicating how many photons are emitted from an excited state. A high quantum yield is desirable since it translates to stronger brightness in imaging. This is an important characteristic because it directly influences the sensitivity of detection methods employed in imaging.
The beneficial aspect of high quantum yield involves enhanced visualization capabilities in low-abundance or rare biological targets. The unique feature of quantum yield relates to its correlation with surface defects on a quantum dot, wherein higher defects can lower the efficiency of light emission. A disadvantage that arises here is the challenge in maintaining high quantum yield across various environmental conditions, particularly in biological systems.
Mechanisms of Imaging
The mechanisms that utilize quantum dots in imaging techniques determine their practical application across various platforms in biomedical research.
Fluorescence microscopy
Fluorescence microscopy employs the principle of light excitation, allowing for visualization at low light levels. This technique is valuable as it provides high sensitivity while enabling the observation of live cells in real-time. A key characteristic of fluorescence microscopy is its ability to generate detailed images at the cellular level.
Fluorescence microscopy is beneficial due to its relatively simple setup, allowing widespread usage in laboratories. The unique feature is the capacity to obtain multi-channel views of different samples simultaneously. However, it does present challenges, such as potential photobleaching and poor penetration depth when imaging thicker tissues.
Multi-photon microscopy
Multi-photon microscopy offers a more advanced method by utilizing near-infrared light to excite quantum dots. This approach allows for deeper tissue penetration and reduced out-of-focus fluorescence. Its key characteristics include low phototoxicity and the capacity to visualize live samples over extended periods.
The advantage of multi-photon microscopy lies in its ability to visualize structures beyond the initial layers of tissue, providing depth insights. A unique aspect is its ability to generate three-dimensional images of biological tissues. Disadvantages include a more complex setup and the requirement for specialized laser systems, which may limit accessibility.
Spectral imaging techniques
Spectral imaging techniques rely on capturing the spectrum of emitted light from quantum dots, allowing for a more comprehensive analysis of samples. This method is crucial for distinguishing multiple quantum dots emitting at similar wavelengths. The key characteristic of this technique is its capability of spectral decomposition.
This method is beneficial since it can provide quantitative information about the concentration of multiple species in a single sample. It is a unique approach that leverages the complete spectral profile of quantum dots, aiding in complex analyses. However, the complexity of data analysis and the need for advanced algorithms can present challenges in application.
The various principles of quantum dot imaging enhance the capability of researchers to visualize and analyze biological samples at unprecedented levels of detail, paving the way for significant innovations in medical and biological research.
The various principles of quantum dot imaging enhance the capability of researchers to visualize and analyze biological samples at unprecedented levels of detail, paving the way for significant innovations in medical and biological research.
Applications in Biological Imaging
The application of quantum dots in biological imaging marks a significant advancement in the field of microscopy and imaging technologies. Quantum dots serve as fluorescent labels that enhance the visibility and accuracy of biological specimens. Their unique properties help researchers conduct more detailed studies of biological processes and interactions at a molecular level. This section elaborates on several crucial aspects of quantum dot imaging in biological contexts, focusing on cellular imaging, tissue imaging, and in vivo imaging. Each application highlights the benefits and considerations unique to quantum dot technology.
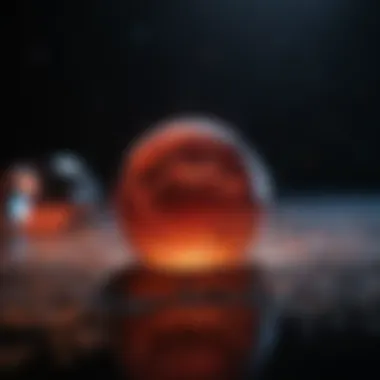
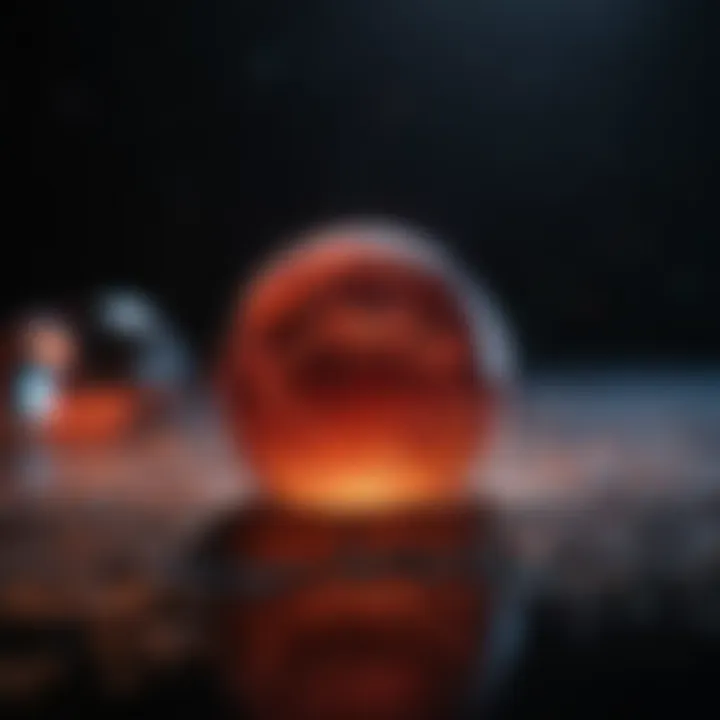
Cellular Imaging
Tracking cellular processes
Tracking cellular processes with quantum dots allows researchers to observe the dynamics of cells in real time. This aspect is vital for understanding cellular behavior, such as migration, division, and response to stimuli. The key characteristic of using quantum dots for this purpose is their high photostability, which enables prolonged imaging sessions without significant signal loss. This durability is particularly beneficial when investigating complex cellular dynamics over extended periods.
One unique feature of tracking cellular processes is the ability to conjugate quantum dots with specific biomolecules. This allows targeting to particular cellular components, aiding in precise tracking. While this has significant advantages, such as increased imaging resolution, one must consider potential drawbacks. For instance, improper conjugation may lead to non-specific binding, complicating data interpretation.
Study of cell surface interactions
The study of cell surface interactions is another critical area where quantum dots excel. This involves analyzing how cells communicate with their environment, including other cells and extracellular matrices. A key characteristic of using quantum dots for monitoring these interactions is their ability to emit distinct colors based on size, allowing for multiplexing. This means multiple interactions can be studied simultaneously, making it a valuable approach in research.
Moreover, quantum dots can be easily modified to bind with various ligands. This unique feature facilitates a broad spectrum of applications, from studying immune cell interactions to exploring cancer cell metastasis. Despite these benefits, researchers must assess the potential toxicity of quantum dots to cells, which can influence the results of the studies.
Tissue Imaging
Histological analysis
Histological analysis using quantum dots provides a powerful tool for examining tissue structure and composition. This application is crucial for diagnosing diseases and understanding organ architecture. The primary benefit of using quantum dots in histology is their strong luminescence, which enhances visibility and contrast compared to traditional dyes. This allows for more detailed visualization of tissue samples, improving diagnostic accuracy.
A distinctive feature of histological analysis with quantum dots is their ability to be tailored for specific biomarkers in tissues. By conjugating quantum dots with antibodies or other specific agents, it is possible to visualize particular cell types or molecular targets accurately. However, there are limitations, including the requirement for careful handling to avoid degradation and ensure reliable results.
3D imaging of tissues
3D imaging of tissues represents another exciting application of quantum dot technology. This method offers a comprehensive view of tissue architecture and function, allowing for the analysis of complex spatial relationships within biological specimens. The key characteristic of 3D imaging is its ability to reconstruct intricate structures in ways that traditional 2D imaging cannot.
Using quantum dots in 3D tissue imaging is advantageous due to their brightness and stability, which facilitate clearer imaging of deep tissue sections. A unique aspect of this approach is the potential for high-resolution imaging at multiple depths. However, challenges remain in terms of the high equipment costs and the expertise required to interpret the extensive data sets generated by 3D imaging techniques.
In Vivo Imaging
Diagnostic imaging
Diagnostic imaging is a prominent application of quantum dots in live organisms. This method enhances the detection of diseases at early stages by providing detailed images of biological processes in real time. Quantum dots offer a significant advantage due to their tunable emission wavelengths, allowing for targeted imaging of various diseases based on the quantum dot composition.
A unique aspect of using quantum dots in diagnostic imaging is their versatility. They can be incorporated into different imaging modalities, including fluorescence and photoacoustic imaging. However, caution is necessary given the concerns regarding biocompatibility and potential toxicity, which could impact the health of the animal during studies.
Research applications in live animals
The research applications of quantum dots in live animals have opened new avenues for understanding biological phenomena in a natural context. By allowing researchers to visualize cellular behavior in vivo, this technology can provide insights into disease progression and treatment responses. The key characteristic of this method is its dynamic observation capabilities, which yield information that static studies cannot provide.
A distinctive feature of research applications using quantum dots is their ability to label multiple targets simultaneously. This multiplexing is particularly useful in studies of complex biological interactions, such as immune responses to pathogens. Nevertheless, ethical considerations and the necessity for comprehensive regulatory compliance remain serious discussions points within the field, as they will influence the future trajectory of quantum dot use in living models.
Technological Advancements
Technological advancements in quantum dot imaging have revolutionized the way researchers approach biological and medical imaging. These innovations enhance the capabilities of quantum dots, making them more effective tools for visualization and analysis. Importantly, improvements in synthesis techniques lead to better quality quantum dots with enhanced stability and emission properties. This directly affects the accuracy and sensitivity of imaging, ensuring that researchers can obtain more precise data when studying cellular and tissue dynamics.
Recent Innovations in Quantum Dot Synthesis
Recent innovations in the synthesis of quantum dots include the development of more efficient colloidal synthesis methods. These methods allow for better size control and uniformity, resulting in more consistent optical properties across batches. Researchers have also explored alternative materials, such as carbon-based quantum dots, which offer potential advantages in biocompatibility and reduced toxicity. Improved techniques continue to minimize defects in quantum dots, leading to enhanced quantum yields and longer lifespans.
Integration with Other Imaging Modalities
Integrating quantum dots with established imaging modalities reveals new possibilities for enhanced imaging resolution and depth.
Combining quantum dots with MRI
Combining quantum dots with MRI harnesses the strengths of both technologies. Quantum dots can serve as contrast agents that enhance the MRI signal, thus improving images of biological tissues. Their unique optical characteristics, including size-tunable fluorescence, add another layer of information that traditional MRI alone cannot provide. This combination is increasingly attractive for researchers aiming to gather comprehensive data from a single imaging session, allowing for real-time monitoring of processes at a cellular level. A key characteristic is the ability to visualize molecular interactions that would be undetectable with MRI alone.
On the downside, there are challenges, such as ensuring compatibility between quantum dots and the magnetic resonance environment, which can complicate imaging protocols.
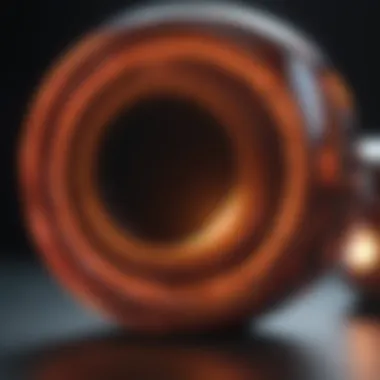
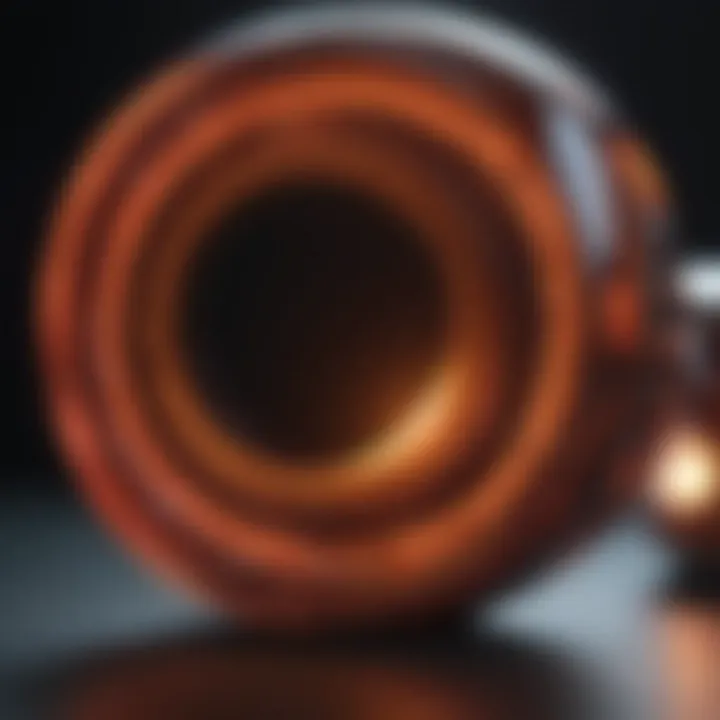
Quantum dots in PET imaging
Incorporating quantum dots in PET imaging represents a promising advancement. The specific aspect of this integration lies in the ability of quantum dots to enhance the spatial resolution of the PET images. They bring an additional layer of detail, making it possible to observe small biological changes over time. The key characteristic of this approach is its capacity to synergize the quantitative features of PET with the qualitative benefits of quantum dots. This leads to superior imaging capabilities in monitoring tumorigenic processes or tracking various diseases.
However, challenges remain, particularly regarding the optimization of quantum dot characteristics for PET compatibility. Their chemical stability can sometimes be an issue, impacting the imaging results. Thus, while combining quantum dots with PET imaging is beneficial, careful consideration of these limitations is essential.
Challenges and Limitations
In the realm of quantum dot imaging, several challenges and limitations significantly impact its practical applications and further development. Understanding these issues is crucial for researchers and practitioners alike. It allows for better planning and strategic thinking in using quantum dots for various imaging purposes. Identifying these challenges can encourage innovation to counteract them, ultimately making quantum dot technology more effective and reliable in biological and medical imaging.
Toxicity Concerns of Quantum Dots
One of the central issues in the application of quantum dots is their potential toxicity. Many quantum dots are made from heavy metals such as cadmium or lead. This raises concerns about their safety in biological systems. Studies have shown that exposure to these materials can lead to cellular damage, inflammation, and even tumorigenesis in some cases. While some advancements have been made to develop less toxic alternatives, such as carbon-based quantum dots, further research is necessary to guarantee their safety.
Additionally, understanding how the body metabolizes and excretes these nanoparticles is vital. If quantum dots accumulate in specific organs, they may pose long-term health risks. The challenge ahead involves developing thorough toxicity assessments and risk management strategies to ensure the safe use of quantum dots in living systems.
Stability and Degradation Issues
Another significant challenge is the stability and degradation of quantum dots under various environmental conditions. Quantum dots can lose their optical properties due to factors like temperature fluctuations, UV light exposure, and chemical environments. This instability can compromise their effectiveness in imaging applications, leading to unreliable results.
Moreover, surface modifications intended to enhance stability can sometimes alter their behavior undesirably. Researchers must work diligently to improve the robustness of quantum dots. Developing protective coatings that maintain their quantum efficiency and overall performance is crucial. This line of research is essential to assure their reliability for in vivo imaging applications.
Regulatory Perspectives
As quantum dot technology evolves, regulatory scrutiny is increasing. The regulatory frameworks surrounding medical imaging and biocompatibility need to adapt to encompass the specific characteristics and behaviors of quantum dots. This includes assessing their safety, efficacy, and potential long-term effects on human health and the environment.
Regulatory agencies worldwide are in the process of developing guidelines for the use of nanoparticles in medical applications. Staying compliant with these regulations is paramount for researchers and companies involved in quantum dot development. Aligning research with regulatory expectations will ensure quicker translation from the laboratory to clinical settings.
"Addressing the challenges of quantum dots is not just about overcoming technical obstacles; it also involves navigating a complex landscape of ethics, safety, and regulatory compliance."
"Addressing the challenges of quantum dots is not just about overcoming technical obstacles; it also involves navigating a complex landscape of ethics, safety, and regulatory compliance."
Future Directions
The exploration of quantum dot imaging continues to unfold, revealing numerous potential avenues for advancement. Understanding the future directions of this field is essential for grasping its broader implications in science and medicine. Quantum dot technology is constantly evolving, leading to improved imaging techniques with enhanced performance characteristics. As researchers push the boundaries of this technology, we are likely to see a shift in how we approach biological imaging, diagnostics, and possibly therapeutic interventions.
One important aspect includes the development of quantum dots with better photostability. This improvement ensures that images remain clear and consistent over time, thereby increasing the reliability of results in long-term studies. Additionally, enhancing the biocompatibility of quantum dots addresses the previously mentioned toxicity concerns, making them safer for use in live subjects, thus broadening their application.
Emerging Trends in Quantum Dot Research
Several trends in quantum dot research have emerged that are shaping the future landscape of imaging. For instance, hybrid materials that integrate quantum dots with other nanomaterials are gaining traction. This combination can lead to multifunctionality in imaging applications, such as improving resolution while providing new insights into molecular interactions.
Key trends include:
- Core-shell structures: These enhance photophysical properties and reduce toxicity, allowing for better imaging outcomes while mitigating risks.
- Targeted quantum dots: Development of quantum dots that can selectively bind to specific biomarkers in cells, aiding in more precise imaging techniques for cancer and other diseases.
- Quantum dot lasing: Advances in quantum dot-based lasers could lead to significant enhancements in the depth and detail obtainable in imaging technologies, pushing resolution limits further.
From the creation of brighter and more efficient quantum dots to their integration with other imaging modalities, these trends signal a promising future for this field.
Potential Applications in Medicine and Beyond
Quantum dot imaging has potential applications that extend far beyond traditional usage in research labs. In medicine, their ability to facilitate non-invasive imaging could transform diagnostic processes. For example, examining tumor microenvironments in real-time is a critical step in improving cancer treatment methodologies.
Moreover, quantum dots can be utilized in:
- Disease diagnosis: By enabling rapid and accurate detection of early-stage diseases through enhanced imaging techniques.
- Theranostics: The convergence of therapeutics and diagnostics. Quantum dots can provide imaging capabilities that allow for the tracking of drug delivery in patients.
- Biosensors: Their unique characteristics can enhance the sensitivity and specificity of biosensors used to detect pathogens or biomarkers in clinical settings.
These applications highlight the significant impact quantum dots could have in medical fields, paving the way for innovations in patient care and diagnostics.
Interdisciplinary Collaborations
The future growth of quantum dot imaging heavily relies on interdisciplinary collaborations. By bringing together experts from fields such as nanotechnology, biology, chemistry, and engineering, the full potential of quantum dots can be realized.
Efforts that integrate various scientific disciplines can foster innovation in:
- Material science: Enhancements in quantum dot synthesis methods can lead to better-performing materials, essential for high-quality imaging.
- Clinical research: Close partnerships can help in translating lab research into viable clinical applications, speeding up the deployment of quantum dots in medical imaging.
- Regulatory policies: Collaborative discussions involving scientists, policymakers, and the industry members can address safety and effectiveness, aiding in the development of regulatory frameworks for new quantum dot technologies.