Exploring RNase Treatment in Molecular Biology
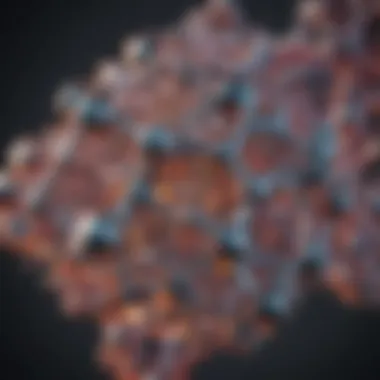
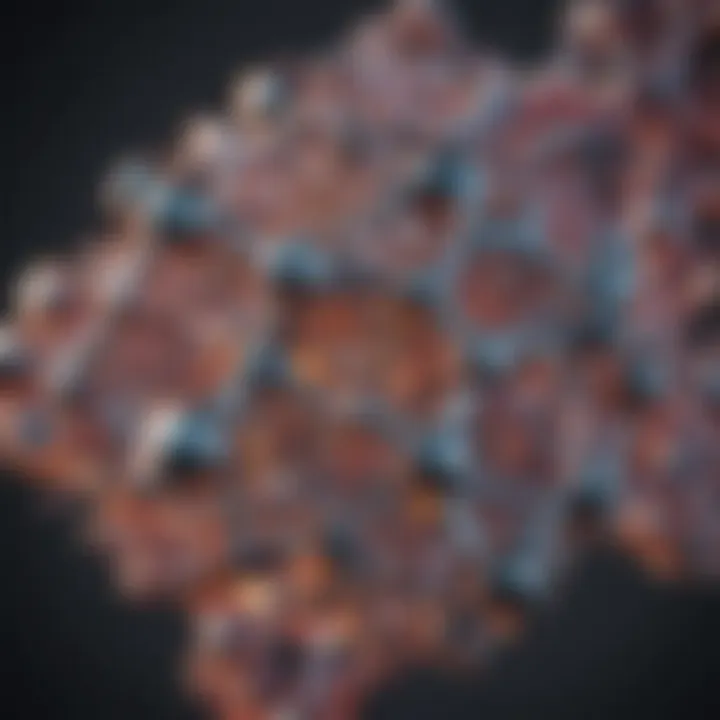
Intro
Ribonucleases (RNases) play a critical role in molecular biology, especially in the manipulation and analysis of RNA. These enzymes are responsible for degrading RNA molecules, impacting various experimental protocols and therapeutic approaches. Understanding how RNases function, their types, and their applications is essential for researchers working in the field. This examination covers the basic concepts, current trends, and future advancements in RNase treatment.
Key Concepts
Definition of the Main Idea
RNase treatment refers to the process where ribonucleases are used to degrade RNA in a controlled manner. This treatment is essential for eliminating unwanted RNA contamination in various biological samples. Notably, the efficacy of RNase treatment relies on understanding the specific mechanisms by which these enzymes interact with RNA.
Overview of Scientific Principles
RNases can be classified broadly into two categories: endoribonucleases and exonucleases. Endoribonucleases cleave RNA at internal sites, while exonucleases remove nucleotides from the ends of RNA. Each RNase has distinct enzymatic properties that determine its specificity and activity under various conditions.
"Ribonucleases are essential tools for researchers, enabling precise control over RNA in their experiments."
"Ribonucleases are essential tools for researchers, enabling precise control over RNA in their experiments."
Effective RNase treatment requires careful handling to avoid undesired degradation of RNA molecules. Typical protocols involve optimizing temperature, pH, and enzyme concentration to achieve desired results without harming the RNA of interest. Moreover, various precautions must be taken to ensure that the tools and reagents used are RNase-free.
Current Research Trends
Recent Studies and Findings
Recent investigations into RNase treatment have produced valuable insights. Studies have shown variations in RNase activity under different ionic conditions and temperatures. This knowledge has led to the development of more refined protocols for RNA purification and analysis.
Significant Breakthroughs in the Field
A promising trend is the integration of RNase treatment in therapeutic applications, particularly in RNA-targeted drug development. As the understanding of RNases expands, researchers are finding innovative ways to harness these enzymes for gene therapy and cancer treatment. This line of inquiry holds great potential for improving therapeutic outcomes and developing new strategies within molecular biology.
By systematically delving into the mechanisms, applications, and advancements in RNase treatment, we can enhance our overall comprehension of RNA processes, ultimately benefiting a wide spectrum of scientific inquiry.
Preamble to RNase Treatment
The field of molecular biology has advanced significantly due to various techniques and methods, one of which is RNase treatment. Understanding this topic is crucial, as RNases play an essential role in controlling RNA levels and ensuring that experiments yield accurate results. The manipulation and degradation of RNA can profoundly affect the outcomes of various research projects, from basic studies to intricate therapeutic applications.
Defining RNase Treatment
RNase treatment refers to the process of using ribonucleases to selectively degrade RNA within a sample. Ribonucleases, commonly known as RNases, are enzymes that target RNA molecules, breaking them down into smaller components. This treatment can be essential in many scenarios within research, such as preparing samples for analysis, eliminating unwanted RNA in cell cultures, and furthering our understanding of RNA biology.
In molecular biology, the specificity of RNases allows researchers to target specific RNA species, which helps in elucidating various biological pathways. Successful RNase treatment optimizes experimental conditions and protocols, thus minimizing unwanted reactions or degradation of target molecules. As such, defining the appropriate RNase to use and understanding its functional properties can greatly impact the efficiency of experimental design.
The Importance of RNA in Molecular Biology
RNA plays several vital roles in molecular biology. It is involved in various cellular processes, including protein synthesis and gene expression regulation. The importance of RNA cannot be overstated, as it acts as a messenger between DNA and ribosomes, where proteins are synthesized. Additionally, newer studies highlight the roles of non-coding RNAs in gene regulation, cellular defense mechanisms, and even cellular signaling.
The effective manipulation and treatment of RNA can therefore provide insights into the complexity of biological functions. Researchers utilize RNase treatment not only to interpret the roles of specific RNA molecules but also to explore therapeutic routes to tackle diseases. For instance, targeting certain RNAs can lead to innovative treatments for viral infections or cancer.
By understanding the nuances of RNase treatment, including its applications and the underlying biological significance of RNA, students and professionals can better engage with fundamental and advanced concepts in molecular biology. This knowledge sets the foundation for exploring the basic mechanisms of RNase action, their various types, and their practical applications.
Basic Mechanisms of RNase Action
Understanding the basic mechanisms of RNase action is crucial in molecular biology. RNases, or ribonucleases, are enzymes that play a pivotal role in the handling and processing of RNA. They can degrade RNA by cleaving its phosphodiester bonds. Knowing how RNases function can lead to improved experimental design and outcomes. This section will elaborate on the types of RNases and their enzymatic properties, which are fundamental in harnessing these enzymes for various applications.
Types of RNases
Endoribonucleases
Endoribonucleases are enzymes that cleave RNA molecules internally. They do so at specific sites within the RNA, resulting in fragments of varying lengths. This characteristic makes them valuable in research and therapeutic settings. By selectively trimming RNA molecules, endoribonucleases can help in studying RNA structure and function. They are also useful for purifying RNA by removing unwanted sequences effectively.
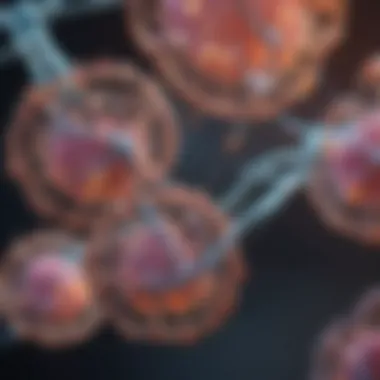
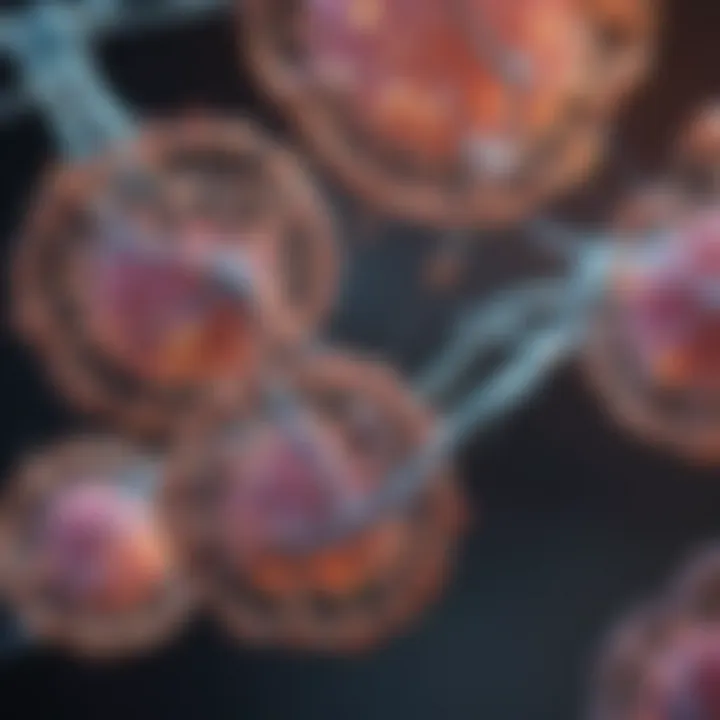
A key feature of endoribonucleases is their specificity. They recognize and act on certain RNA sequences, which allows researchers to target precise RNAs without affecting others. This makes them a favorable option for RNA manipulation in molecular biology. However, the downside is that improper use can lead to unintended degradation of essential RNA molecules.
Exoribonucleases
Exoribonucleases differ from their endonuclease counterparts in that they degrade RNA from either the 5' or 3' end. This action results in the progressive removal of nucleotides, which can be beneficial for quality control in RNA studies. Exoribonucleases allow for the fine-tuning of RNA fragments, which is often necessary in transcriptomic analyses.
The primary advantage of exoribonucleases lies in their ability to produce smaller RNA fragments consistently. This can be particularly helpful in generating RNA samples suitable for further analysis. One limitation, however, is the potential for loss of important RNA sequences during the degradation process.
Enzymatic Properties of RNases
Specificity
The specificity of RNases is a critical concept. It refers to an enzyme’s ability to target specific RNA substrates without affecting others. This property is essential in applications such as RNA purification and transcriptomics. Knowing which RNase to use depends on understanding its specificity. Using highly specific RNases minimizes off-target effects, thus preserving the integrity of the RNA that researchers want to study.
The unique feature of specificity allows for versatile applications in molecular biology. However, misleading results may arise if specificity is not accurately matched with the RNA targets. Therefore, it is crucial for researchers to select RNases that are aligned with their experimental goals.
Activity Conditions
The activity conditions of RNases refer to the optimal environments necessary for their effective function. Factors such as temperature, pH, and ion concentration can significantly affect RNase activity. Understanding these conditions is important to ensure that RNases operate at peak efficiency during experiments.
Optimal activity conditions enhance the reliability of experimental results. For instance, some RNases may require specific temperatures to maintain stability and activity. If these factors are not closely monitored, the results may yield inconsistent data. Careful adjustment of activity conditions based on the chosen RNase can improve the success rates of RNA treatment protocols.
Applications of RNase Treatment
The significance of RNase treatment in molecular biology cannot be understated. RNases have evolved into invaluable tools for various applications. Their versatility offers benefits in purifying RNA, advancing transcriptomics, and creating therapeutic strategies. Understanding the applications of RNase treatment allows researchers to harness the full potential of these enzymes, leading to improved methodologies and outcomes in their studies.
RNase in RNA Purification
The purification of RNA is crucial in molecular biology research. RNases play a central role in this process. They effectively degrade unwanted RNA contaminants, thus enhancing the purity of the target RNA. This purification method is essential when isolating RNA for subsequent analyses, such as quantitative PCR or sequencing.
In practical terms, using RNases in RNA purification often involves specific protocols to ensure efficacy. Care must be taken to avoid contamination, as RNases are potent enzymes that can degrade RNA indiscriminately. Researchers usually work in RNase-free environments to minimize risks.
Key points to note in RNA purification include:
- Selection of RNase: Choosing the right type of RNase, like endoribonucleases, can influence the purification quality.
- Concentration and exposure time: Optimal concentrations and exposure can lead to efficient purification without excessive degradation of the target RNA.
- Post-treatment analysis: Following RNase treatment, it is essential to confirm the integrity of RNA using methods like gel electrophoresis.
Role of RNase in Transcriptomics
Transcriptomics has drastically changed our understanding of gene expression and regulation. RNases are pivotal in transcriptomic studies by enabling researchers to concentrate on specific RNA molecules of interest. The treatment with RNases aids in the filtration of unnecessary RNA from biological samples, which can confound results.
Moreover, RNase treatment is often integrated into workflows for RNA-Seq (RNA sequencing). By ensuring that only intact mRNA is sequenced, researchers can gain clearer insights into gene expression profiles. It is especially important in high-throughput sequencing, where quality and specificity of RNA samples are critical.
Factors influencing the role of RNase in transcriptomics include:
- Sample complexity: Different tissues may contain varying RNA compositions, which require tailored RNase treatments.
- Technology integration: Consideration of detection methods combined with RNase treatment can enhance data quality.
Therapeutic Applications of RNases
The therapeutic potential of RNases is an emerging area of research. Certain ribonucleases exhibit anticancer properties and have been explored as treatment options. For example, the ribonuclease ONCONASE has undergone clinical trials as a cancer therapy. This application underscores the significant role of RNases beyond basic research.
In therapeutic settings, the specificity and activity of RNases against tumor cells can be crucial. These properties have implications in designing drugs that leverage RNases for targeted treatment of diseases. Understanding the nuances of RNase behavior can help in developing more effective therapies.
Researchers must consider:
- Target selectivity: The ability of RNases to differentiate between healthy and cancerous cells.
- Delivery mechanisms: Effective means to deliver RNases to target sites in the body.
"The use of RNases in therapy not only highlights their functional versatility but also opens new avenues for clinical applications."
"The use of RNases in therapy not only highlights their functional versatility but also opens new avenues for clinical applications."

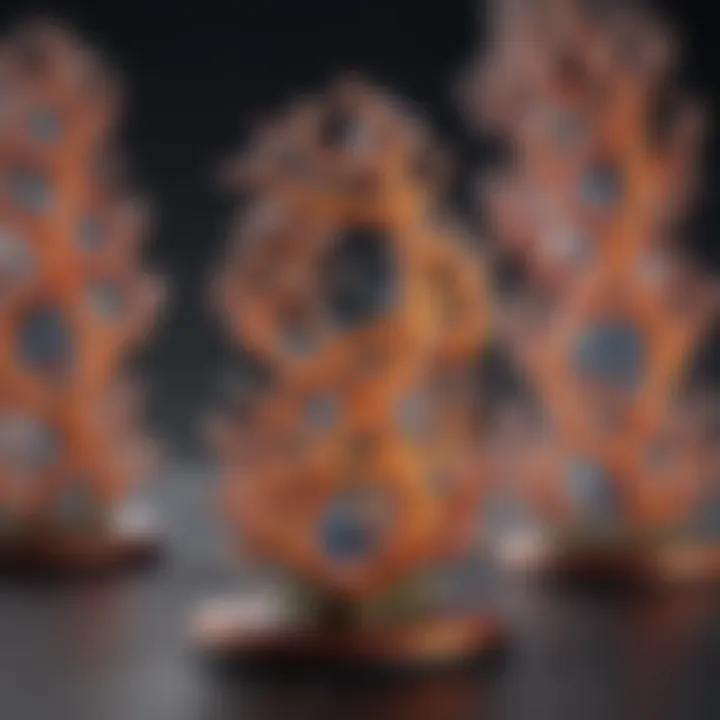
In summary, the applications of RNase treatment span across RNA purification, transcriptomic analysis, and therapeutic development, making them indispensable in contemporary molecular biology.
Protocols for Effective RNase Treatment
The protocols for effective RNase treatment are crucial in ensuring the successful application of ribonucleases in various molecular biology experiments. These protocols detail the methodologies that maximize the efficiency of RNA treatments while preserving the integrity of the desired RNA samples. Effectively followed protocols can lead to reproducible results, essential for both academic research and practical applications in therapeutics.
Proper preparation of RNase solutions, optimal treatment conditions, and careful handling are critical aspects of these protocols. They also mitigate the risk of unintended RNA degradation, which could compromise experimental outcomes. Addressing these factors in detail will enhance the reader's understanding of how to implement RNase treatment effectively.
Preparation of RNase Solutions
The preparation of RNase solutions is a fundamental step in using ribonucleases effectively. This process involves selecting the appropriate RNase based on the specific needs of the experiment and dissolving the enzyme in a suitable buffer. A commonly used buffer is Tris-HCl, as it maintains a stable pH, which is vital for enzyme activity. When preparing RNase solutions, it is important to ensure that the final concentration of the enzyme meets the requirement of the experiment.
Furthermore, the solutions should be prepared in an RNase-free environment to prevent contamination. Using RNase-free water, tubes, and pipette tips is essential. These precautions help maintain the purity of the solution and enhance the effectiveness of RNase treatment.
Optimal Treatment Conditions
Optimal treatment conditions encompass the two pivotal factors of temperature and time duration. Each greatly influences the effectiveness of RNase treatment and therefore must be defined clearly for every particular protocol.
Temperature
Temperature is a vital element in RNase treatment. The activity of ribonucleases can vary significantly with temperature changes. Generally, a temperature of around 37°C is often used, as it closely mimics physiological conditions. The main characteristic of this temperature is that it is advantageous for most RNases to function efficiently without denaturing. However, higher temperatures can provide rapid activity but may increase the risk of enzyme instability. Thus, the unique feature of ambient or physiological temperature offers a balance between activity and stability, making it a popular choice in many experiments.
Time Duration
Time duration is equally important in the context of RNase treatment. The duration of treatment directly correlates with the extent of RNA degradation. Protocols must precisely define the treatment length according to the specific RNase in use and the concentration of RNA present.
Typically, short treatment times, such as 5 to 30 minutes, can yield effective results, allowing for specific RNA degradation without excessive damage. The unique characteristic of time duration is its flexibility based on experimental needs. However, longer durations can lead to unwanted degradation. Therefore, careful consideration of treatment time is essential for achieving optimal results.
Preventing Unwanted RNA Degradation
Preventing unwanted RNA degradation is a critical aspect of molecular biology research. RNA is inherently unstable and susceptible to degradation by ribonucleases (RNases). This degradation can interfere with experiments, leading to inaccurate results or loss of valuable samples. Therefore, a proactive approach is crucial for maintaining the integrity of RNA throughout experimental procedures.
One of the primary benefits of preventing RNA degradation is the assurance of reproducibility in experiments. When RNA is compromised, it not only affects the quality of the data but can also produce inconsistent results across multiple trials. This lack of reliability undermines the credibility of research findings and impedes progress in the field. Moreover, preventing degradation safeguards the financial investments made in reagent and material purchases, as valuable samples or ongoing experiments may have to be redone.
Contamination Prevention
In molecular biology, the prevention of contamination is paramount to maintaining RNA integrity. RNases are ubiquitous and can be found on skin, in dust, and even within laboratory equipment. To mitigate this risk, a combination of practices should be adopted.
- Use of RNase-free materials: Always utilize RNase-free tubes, tips, and reagents. Look for products labeled as "RNase-free" to ensure minimal risk of contamination.
- Personal protective equipment (PPE): Wearing gloves and using lab coats can help prevent transferring RNases from hands onto samples.
- Surface decontamination: Regularly clean work surfaces with RNase decontaminants. Additionally, ultraviolet light can be employed as a further safeguard against potential contaminating enzymes.
By implementing these measures, researchers create an environment that significantly reduces the risk of RNA degradation.
Use of RNase Inhibitors
RNase inhibitors play an essential role in preserving RNA integrity. These inhibitors are designed to specifically bind to and inactivate RNases, thus preventing degradation during experiments. Their use is particularly beneficial in experiments involving RNA isolation, amplification, or any sensitive application where RNA integrity is essential.
The choice of RNase inhibitor is critical and should be based on the specific requirements of the experiment. It's also worth noting that some common RNase inhibitors include:
- Superase-In: A highly effective inhibitor suitable for a wide variety of applications.
- RNase Inhibitor from New England Biolabs: Known for its potency even at low concentrations.
Moreover, it is important to ensure that the chosen RNase inhibitors do not interfere with downstream applications, such as qPCR or cloning. Proper control experiments should always accompany the use of these inhibitors to validate their effectiveness.
"Maintaining RNA integrity not only plays a vital role in the accuracy of results but also impacts the future prospects of molecular biology research."
"Maintaining RNA integrity not only plays a vital role in the accuracy of results but also impacts the future prospects of molecular biology research."
Challenges and Limitations of RNase Treatment
RNase treatment is essential for various aspects of molecular biology, but it also comes with its own set of challenges and limitations that can affect experimental outcomes. Understanding these issues is crucial for researchers who seek to employ ribonucleases effectively while minimizing errors in their protocols. The following sections will delve into specific issues that arise during RNase treatment, as well as the repercussions they may have on subsequent experiments.
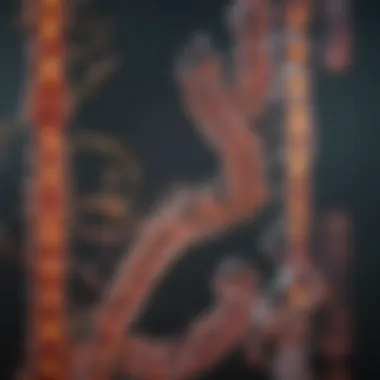
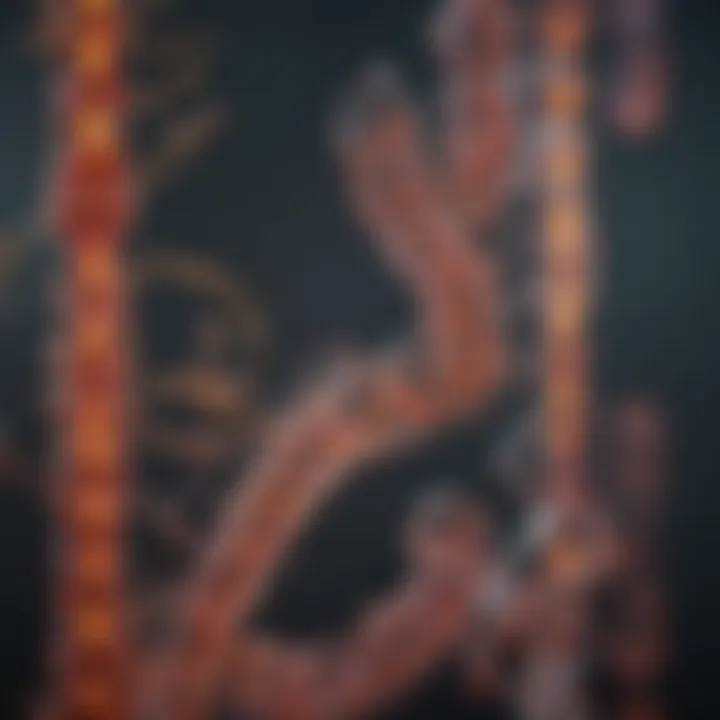
Specificity Issues
One of the primary challenges of RNase treatment relates to the specificity of ribonucleases. RNases vary in their substrate preferences, with some enzymes displaying broad-spectrum activity that can lead to unintended cleavage of non-target RNA. This lack of specificity may compromise the integrity of RNA samples by degrading essential transcripts that are necessary for downstream applications such as RT-PCR or RNA sequencing. When using RNases in experiments, it is vital to consider the enzyme's activity profile and select one that minimizes the risk of degrading unwanted RNA.
Moreover, the presence of other nucleic acids can complicate these specificity issues. Deoxyribonucleases (DNases) may also be present during procedures that include both DNA and RNA, leading to potential cross-contamination. This can result in a mixed degradation profile, making it challenging to identify the source of RNA loss.
"Understanding enzyme specificity is a key factor in avoiding pitfalls during RNase treatment."
"Understanding enzyme specificity is a key factor in avoiding pitfalls during RNase treatment."
Careful titration of RNases and the use of selective inhibitors can help mitigate these specificity concerns. Researchers must remain vigilant about the potential biases introduced by RNase treatment and ensure that comprehensive controls are in place.
Impact on Downstream Applications
The implications of RNase treatment extend well beyond the immediate action of the enzyme. The degradation of RNA can have cascading effects on downstream applications. For instance, if the target RNA is partially or entirely degraded, the results of quantitative PCR can be skewed. Accurate quantification is paramount in studies that rely on RNA analysis — any discrepancies can lead to erroneous conclusions.
Furthermore, applications such as RNA sequencing can be adversely affected by RNase treatment. High-throughput sequencing technologies require intact RNA samples for accurate library preparation and analysis. If the RNA has been compromised by RNase, the sequencing results may yield incomplete data or substantial biases in read counts.
Issues may also arise during functional studies reliant on RNA integrity. For example, experiments that involve RNA-protein interactions necessitate intact RNA for reliable interaction assessment. Degraded RNA may produce misleading results, posing challenges in validating biological hypotheses.
In summary, while RNase treatment is a powerful tool for RNA research, its challenges and limitations should not be overlooked. Addressing specificity issues and understanding their impact on downstream applications is vital for obtaining reliable and reproducible results in molecular biology.
Recent Advances in RNase Research
Research into ribonuclease (RNase) treatment has seen significant advancements in recent years. This progress is vital for the molecular biology field, as it addresses core issues associated with RNA manipulation and degradation. Understanding these advancements not only enriches the current knowledge base but also provides insights for optimization in laboratory protocols. The increased precision and effectiveness in RNase applications can lead to enhanced results in various experimental contexts.
Innovations in RNase Engineering
Recent innovations in RNase engineering have resulted in creating enzymes with improved specificity and reduced off-target effects. By using techniques like directed evolution and protein engineering, scientists have developed RNases that can selectively target certain RNA sequences. This advancement is crucial for therapeutic applications where precision is paramount.
For example, engineered RNases can be designed to avoid essential RNA elements in cells while still degrading unwanted RNA. This specificity ensures that the therapeutic impact of RNases is maximized while minimizing potential harm to normal cellular functions.
Moreover, advancements in delivery mechanisms have also played a role. New carriers can transport engineered RNases into cells effectively. This enhanced delivery system could be a game changer in targeting diseases at the molecular level. With better access to target RNA molecules, potential treatments for disorders linked to RNA dysfunction become more feasible.
Future Directions in RNase Applications
The future directions in RNase applications are promising, with opportunities to extend their use even further in research and clinical settings. As molecular biology continues to evolve, new findings about RNA structures and functions pave the way for innovative RNase applications.
Researchers are particularly focused on utilizing RNases in gene therapy. The ability to manipulate RNA could help rewrite disease pathways, possibly stopping diseases before they progress. Furthermore, combining RNases with CRISPR technologies could lead to more targeted and versatile therapeutic strategies.
Another emerging area is the incorporation of RNase treatment in synthetic biology. Here, RNases could be used to modulate RNA networks, creating engineered organisms or systems for specific biotechnology applications.
"The fusion of RNase applications with cutting-edge technologies marks a new era in molecular biology."
"The fusion of RNase applications with cutting-edge technologies marks a new era in molecular biology."
In addition, there is also an increasing interest in exploring the functionality of RNases in regulating gene expression and their potential in developing RNA-based vaccines. As we probe deeper into their roles within cellular pathways, the possibilities for new applications continue to expand, promising a transformative impact on both research and therapeutic developments in the coming years.
Epilogue
The conclusion serves as a critical component of this article, encapsulating the extensive discussion surrounding RNase treatment. It offers an opportunity to reflect on the essential insights gleaned from earlier sections. The knowledge about RNase treatment is indispensable for those engaged in molecular biology, as it holds profound implications for both experimental integrity and therapeutic advancements.
Summarizing Key Insights
The exploration of RNase treatment reveals several key insights that are vital for both emerging and established researchers.
- Fundamental Understanding: A clear grasp of ribonucleases, their types, and actions underscores the role they play in RNA manipulation and study. Different RNases possess unique enzymatic properties that impact their utility.
- Practical Applications: The diverse applications of RNases highlight their significance in RNA purification, transcriptomics, and potential therapeutic approaches. This showcases the versatility of RNases across various research domains.
- Protocols and Techniques: The effective protocols developed for RNase treatment emphasize the need for precise conditions to ensure the desired outcomes. Knowledge of optimal treatment conditions ensures efficient use of RNases, contributing to the reliability of experimental results.
- Challenges and Limitation: Understanding the challenges involved in RNase treatment prepares researchers to navigate specificity issues and the impact on downstream applications. Awareness of these limitations is crucial for troubleshooting and improving experimental design.
Implications for Future Research
Future research in RNase treatment and applications stands to contribute significantly to the field of molecular biology. Several implications arise from current findings:
- Innovation in RNase Engineering: Advances in engineering modified RNases could lead to developments with enhanced specificity and activity. This could open new avenues for therapeutic applications, making RNases even more effective in clinical settings.
- Investigation of Novel RNase Functions: Ongoing research may reveal new roles for RNases beyond what is currently understood. This exploration may unveil unique interactions and functionalities that can further enrich the landscape of molecular biology.
- Tailored Therapeutic Strategies: The integration of RNase applications within therapeutic frameworks can inspire personalized treatment options. Understanding individual responses to RNases can guide tailored therapeutic approaches in fields like oncology.
- Interdisciplinary Collaborations: The increasing recognition of RNases in various biological contexts promotes collaborative efforts between molecular biologists, biochemists, and clinical researchers. Such collaborations can lead to comprehensive strategies for addressing complex biological challenges.
In summary, the conclusion emphasizes the importance of continuous exploration in the field of RNase treatment, paving the way for innovative methodologies and applications that have the potential to reshape therapeutic landscapes.