Understanding Solar Cell Production and Its Effects
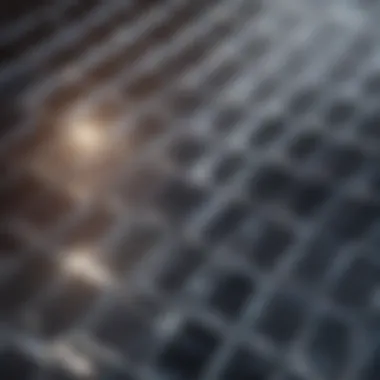
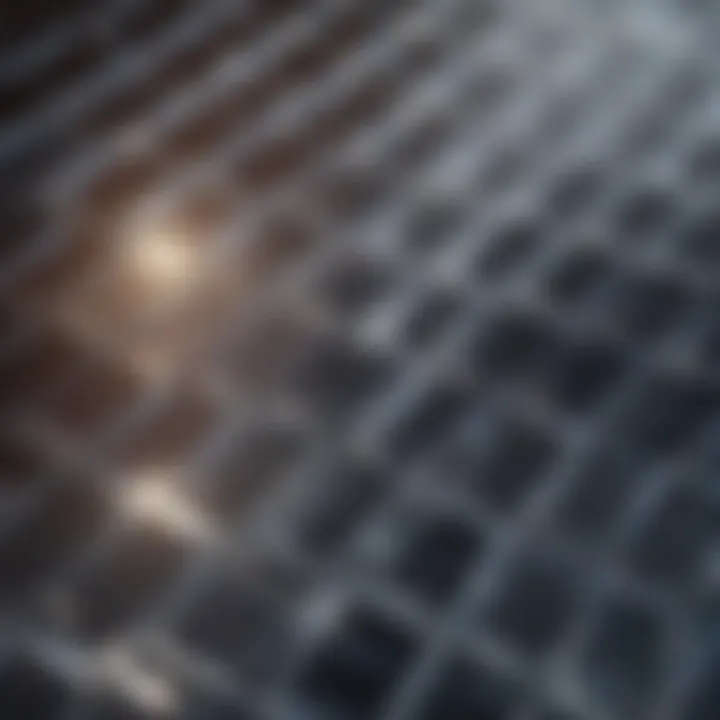
Intro
In the ever-evolving landscape of renewable energy, solar cells stand out as a beacon of innovation and promise. Production of solar cells encompasses a range of intricate processes that hinge on a mix of advanced materials, physics, and engineering practices. This article is crafted to take readers—from students to professionals—through the delicate mechanisms involved in solar cell production, mapping out the landscape including the environmental implications and socioeconomic factors at play.
Key Concepts
Definition of the Main Idea
Solar cells, often dubbed photovoltaic (PV) cells, are devices that convert sunlight directly into electricity by utilizing the photoelectric effect. But there’s much more beneath the surface. Understanding solar cell production means diving deeper than just a simplistic explanation. It involves discussions about materials such as silicon, cadmium telluride, and various emerging compounds. Each of these materials carries its unique properties, impacts efficiency, lifespan, and cost-effectiveness.
Overview of Scientific Principles
To make sense of how solar cells function, it’s essential to grasp a few key scientific principles:
- Photoelectric Effect: This is the fundamental phenomenon enabling solar cells to generate power. When photons hit the semiconductor material, they excite electrons, leading them to break free from their atoms.
- Band Gap Theory: Each material has a specific energy band gap that determines how well it can convert sunlight to electric energy. The band gap tells us which light wavelengths the material can absorb.
- Circuitry Integration: Beyond basic energy conversion, how solar cells are interconnected also plays a crucial role in efficiency. This conversation extends into how inverters convert DC to AC power for household use.
Current Research Trends
Recent Studies and Findings
Recent studies have been uncovering advancements that challenge traditional notions of efficiency and material use. For instance, a study published on Wikipedia indicated that organic photovoltaic cells have been increasing in efficiency, prompting a reconsideration of their place in the market. Researchers are also exploring bifacial solar cells that can harvest light from both sides, significantly boosting energy output.
Significant Breakthroughs in the Field
One breakthrough that garners attention revolves around perovskite solar cells. These cells promise higher efficiency rates and lower production costs, potentially leading to a revolution in solar technology. Reports in Britannica detail how their structure is much simpler than traditional silicon cells, bringing new strategies for large-scale manufacturing.
"The rise of perovskite technology symbolizes a noteworthy leap that could redefine the solar landscape in the coming years."
"The rise of perovskite technology symbolizes a noteworthy leap that could redefine the solar landscape in the coming years."
Moreover, integration of artificial intelligence in production processes is being researched to optimize manufacturing efficiency and product longevity.
Prelude to Solar Cells
Solar cells play a pivotal role in modern energy solutions, converting sunlight into electricity. Their significance stretches far beyond mere technology; they are a cornerstone in the transition towards sustainable energy sources, a necessary step in combating climate change and reducing carbon footprints. In a world grappling with depleting fossil fuels and environmental degradation, solar cells stand out not only for their efficiency but also for their potential to integrate seamlessly into various aspects of our lives—from powering homes and businesses to enabling electric vehicles.
As we delve deeper into the topic, several key elements emerge that underscore the relevance of solar cells:
- Renewable Energy Source: Unlike traditional fuels, solar energy is abundant and renewable, providing a steady supply of energy as long as the sun shines.
- Technological Advancements: Innovations in solar cell manufacturing and efficiency are constantly reshaping the landscape of renewable energy, making it more accessible and affordable for consumers.
- Economic Impact: The solar industry not only contributes to energy independence but also creates jobs and stimulates local economies, with growth opportunities in research, development, and installation sectors.
This section acts as a gateway into the fascinating world of solar technology, providing the backdrop against which the intricacies of solar cell production will unfold.
Definition and Functionality
At its core, a solar cell is a device that converts light energy directly into electrical energy through the photovoltaic effect. Essentially, when photons from sunlight hit the solar cell's material—usually silicon—it excites electrons, creating a flow of electric current. This process seems simple, yet it involves a series of complex interactions between light and matter.
Solar cells are typically made of semiconductor materials, and their design can vary significantly, affecting their efficiency and applications. The primary functionality involves capturing sunlight, converting it, and outputting electricity that can power anything from household appliances to large-scale solar farms. This functionality has made solar cells indispensable in our push toward cleaner energy sources.
Brief History of Solar Cell Development
The journey of solar cells began in the mid-19th century, with scientists like Antoine Henri Becquerel discovering the photovoltaic effect in 1839. Fast forward to 1954, when researchers at Bell Labs created the first practical silicon solar cell, boasting an efficiency of around 6%. This breakthrough set the stage for the solar revolution.
Over the years, several milestones have marked the evolution of solar technology:
- 1960s: The deployment of solar cells began to gain traction, especially in aerospace applications.
- 1970s: Government initiatives and fuel crises spurred interest in solar technology, making it a focus for energy research.
- 1990s: Advancements in material science led to the development of more efficient photovoltaic cells, making solar power more economically viable.
- 2000s to Present: The emergence of thin-film and multi-junction cells has drastically improved efficiency. Nowadays, many households and industries incorporate solar technology, thanks to decreased costs and increased awareness of environmental issues.
These advancements reflect a significant commitment to harnessing solar energy, setting the groundwork for ongoing innovation in solar cell production and broader applications in sustainable technology.
Types of Solar Cells
Understanding the various types of solar cells is fundamental to grasping the technological advancements within solar energy production. Solar cells can be broadly classified based on their material composition, construction methods, and performance characteristics. Each type brings distinct advantages and considerations that can influence energy output, efficiency, and even an installation's overall cost.
When comparing these different types of solar cells, factors like efficiency, lifespan, installation space, and production costs come into play. It is crucial whether you are a homeowner considering solar panels or a researcher examining new technologies. The choice of solar cells determines not just the energy generation potential, but also the environmental impact and viability of solar solutions in diverse applications.
Monocrystalline Solar Cells
Monocrystalline solar cells are readily recognized by their uniform dark color and rounded edges. They are crafted from a single crystal structure. This process yields high purity silicon, leading to greater efficiency levels typically ranging from 15% to 20% or even higher.
- Advantages
- Considerations
- High efficiency with less space needed for installation.
- Long lifespan, often backed by warranties stretching beyond 25 years.
- Performs better in low-light conditions compared to other types.
- Generally, the most expensive to produce, reflecting in higher retail prices.
- Sensitive to high temperatures, efficiency might drop in extreme heat.
The investment in monocrystalline systems can be substantial, but potential users are likely to see robust returns over time through increased energy production.
Polycrystalline Solar Cells
Polycrystalline solar cells are distinguished by their bluish hue and square edges, formed by melting multiple silicon crystals together. This method is more cost-effective than that of monocrystalline cells, making polycrystalline an attractive option.
- Advantages
- Considerations
- Lower production costs, providing a cheaper alternative.
- Easier and faster manufacturing process.
- Eco-friendlier, as the melting process generates less waste.
- Typically less efficient compared to monocrystalline, often scoring between 13% and 16% efficiency.
- Slightly requires more space for installation to generate equivalent power output.
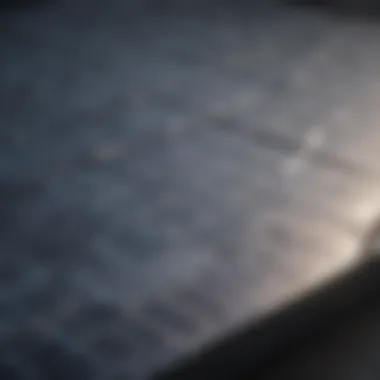
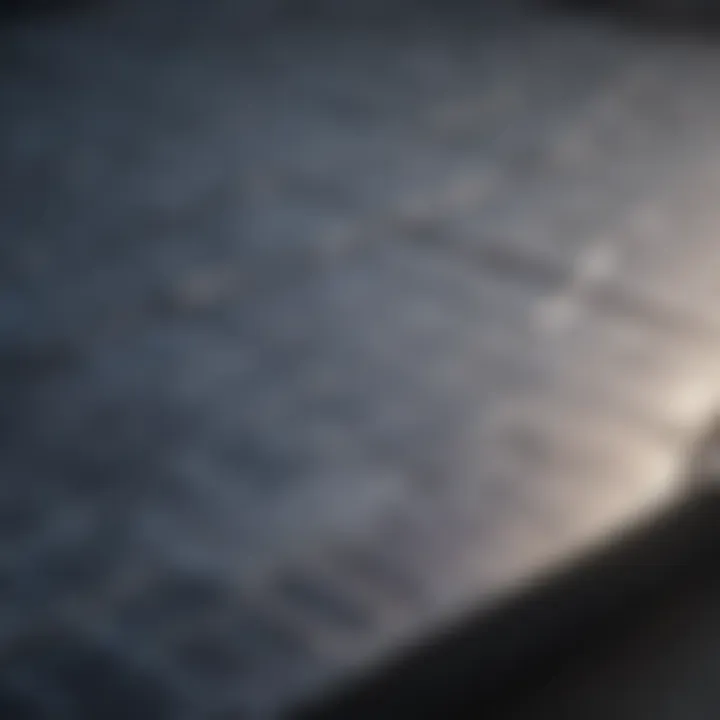
Polycrystalline solar cells are a popular choice for budget-conscious consumers and those looking for eco-friendly options in their solar installations.
Thin-Film Solar Cells
Thin-film solar cells offer a radically different approach by utilizing layers of photovoltaic material just a few micrometers thick. This design allows for incredible flexibility and versatility in applications, ranging from commercial use to residential rooftops.
- Advantages
- Considerations
- Lightweight and flexible, enabling application in varied surfaces and structures.
- Can perform better in high temperatures and partial shading conditions.
- Generally, cheaper to produce due to lower material costs.
- Lower efficiency, usually between 10% to 12%, necessitating larger areas for installation.
- Shorter lifespan compared to crystalline counterparts—typically lasting under 20 years.
Thin-film technology, while sometimes viewed as less efficient, holds promise for specific applications where space and weight limitations are significant factors.
The choice among monocrystalline, polycrystalline, and thin-film solar cells reflects not just individual needs but also broader implications for energy production in a rapidly changing world.
The choice among monocrystalline, polycrystalline, and thin-film solar cells reflects not just individual needs but also broader implications for energy production in a rapidly changing world.
Materials Used in Solar Cell Manufacturing
The choice of materials in solar cell manufacturing is crucial. It dictates the efficiency, cost, and environmental impact of solar technology. As the world shifts towards renewable energy, understanding these materials will help to grasp their effect on solar cell production and adoption.
Silicon: The Most Common Material
Silicon is the backbone of the solar cell industry. Present in abundant quantities in the Earth’s crust, it offers an attractive combination of properties for photovoltaic applications.
Silicon is widely used due to its crystalline structure, which effectively converts sunlight into electricity. When doped with elements like phosphorus or boron, silicon can create an electric field. This field is essential for allowing electron flow, generating current when exposed to light.
The production process of silicon solar cells involves:
- Purification: High-purity silicon is crucial. It is obtained through various methods, including the Siemens process, where silicon is transformed into trichlorosilane and then back into solid silicon in a reactor.
- Wafer Slicing: Large blocks of silicon, called ingots, are sliced into thin wafers. This step is precise and affects the efficiency of the final product. Missing the mark here can lead to more waste and higher costs.
- Cell Fabrication: After slicing, the wafers undergo further treatments to create an anti-reflective coating and electrical contacts.
"Silicon’s versatility has become its hallmark, yet as we dig deeper, its limitations also emerge."
"Silicon’s versatility has become its hallmark, yet as we dig deeper, its limitations also emerge."
Thus, while silicon solar cells dominate the market, their production carries some environmental concerns, primarily involving energy-intensive processes and waste generation. Efficient recycling methods are essential to mitigate these impacts.
Alternative Materials: Gallium Arsenide and Perovskite
Though silicon leads the pack, alternative materials are shaking up the solar cell scene. Gallium arsenide (GaAs) and perovskite are gaining attention for their exceptional properties.
Gallium Arsenide:
- GaAs is known for its high efficiency and performance under low light conditions. Its ability to convert sunlight into electricity reaches above 30%, making it superior to traditional silicon cells.
- However, it is expensive and primarily used in specialized applications, such as space technology.
Perovskite:
- This new player is celebrated for its ease of fabrication and potential for high efficiency. It’s a material with a unique crystal structure that allows for absorption of a broader spectrum of light.
- Perovskite solar cells can be manufactured at lower costs compared to silicon cells. Yet, questions around stability and long-term environmental impact remain.
Both alternatives highlight the breadth of innovation happening in solar technology, each with its own benefits and drawbacks.
Emerging Materials and Technologies
Next-gen materials are under the microscope. Research is bubbling up on several fronts, pushing the boundaries of solar cell production further into possibilities we might not have envisioned a few years ago.
- Organic Photovoltaics (OPVs): These utilize carbon-based materials. They are lightweight and can be produced via roll-to-roll processing, which is cost-effective. However, they currently lag in efficiency compared to silicon cells.
- Quantum Dot Solar Cells: Leveraging nanotechnology, quantum dots can absorb light across a wide spectrum, potentially allowing for higher efficiency and lower production costs.
- Bifacial Solar Panels: Employing dual-sided panels, these cells capture sunlight from both sides. This design enhances energy output and takes advantage of reflected light.
As we forge ahead, these advancements invite more sustainable practices while aiming to overcome the limitations faced by traditional materials. Evaluating their lifecycle and integration into existing technological frameworks will be pivotal in shaping a cleaner future.
The Physics of Solar Energy Conversion
Understanding the physics behind solar energy conversion is crucial for grasping how solar cells work and their potential to reshape our energy landscape. This knowledge provides insight into the mechanisms that convert sunlight into electricity. Solar energy is not just a renewable resource; it embodies a sophisticated interplay of materials, light, and electronic movements that convert photons into usable electrical energy.
Photovoltaic Effect Explained
At the heart of solar technology lies the photovoltaic effect, which is the phenomenon where certain materials generate an electric current when exposed to sunlight. This process begins when photons, the fundamental particles of light, strike the surface of a solar cell.
When these photons hit the solar cell, they can transfer their energy to electrons in the material, often silicon, creating electron-hole pairs. An electron is a negatively charged particle, while a hole is merely an absence of an electron, behaving like a positively charged particle. This interaction is summarized as:
- Photon absorption: Light energy is absorbed by the electron.
- Electron excitation: The absorbed energy is sufficient to knock the electron loose from its atom, creating a pair of electron and hole.
- Separation of charges: Electric fields within the solar cell push electrons towards the negative side and holes to the positive side, generating a flow of electric current.
This flow of current can then be harnessed to power electrical devices. Understanding this interplay not only reveals the mechanics behind solar cells but also highlights possible enhancements to their efficiency.
Efficiency Factors in Solar Cells
The efficiency of solar cells, defined as the ratio of electrical output to the solar energy input, plays a prominent role in the viability of solar technology. Multiple factors influence this efficiency, including:
- Material Quality: The purity and crystalline structure of the materials used greatly affect performance. For instance, higher quality silicon can lead to better light absorption and reduced energy loss.
- Temperature Conditions: Solar cells often perform better under cooler conditions. When temperatures rise, it can decrease the efficiency of energy conversion.
- Angle of Incidence: The angle at which sunlight strikes the solar cells also matters. Optimal positioning ensures maximum exposure to direct sunlight; thus, tracking systems are sometimes employed.
- Surface Texturing and Coatings: Enhancements such as anti-reflective coatings can maximize light entry by decreasing reflection, making sure more photons contribute to energy generation.
Keeping these factors in mind is important for developing new solar technologies and improving existing ones.
Manufacturing Processes
The manufacturing processes of solar cells is a pivotal topic within the broader discussion of solar technology. These processes not only determine the efficiency and performance of solar cells but also have substantial implications for cost and scalability in the solar industry. Understanding these processes equips stakeholders—from students to professionals—with a clear picture of how solar cells are produced and the integral role they play in harnessing solar energy for a sustainable future.
Silicon Wafer Production

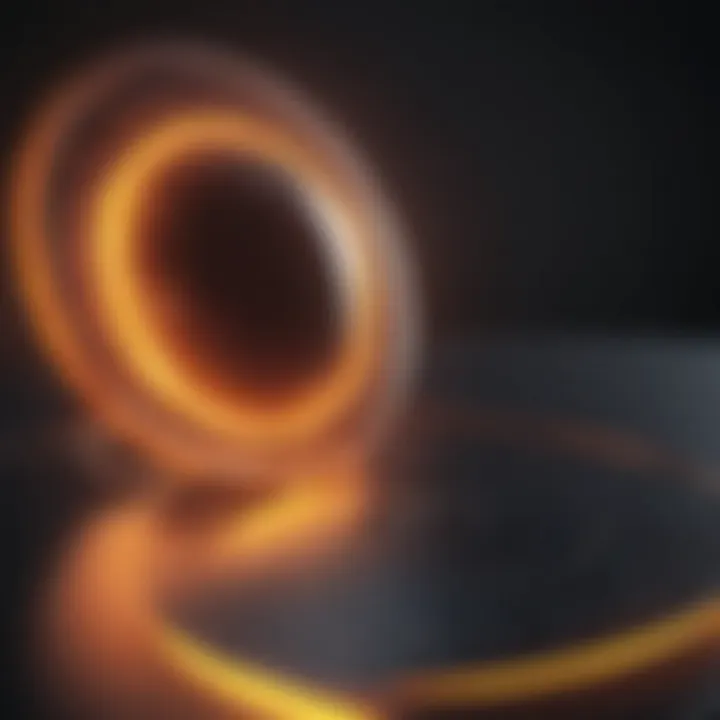
Silicon wafer production is fundamentally the first step in creating most solar cells, particularly the widely used monocrystalline and polycrystalline types. This process begins with the purification of silicon, which involves heating silica with carbon in an electric arc furnace, yielding metallurgical-grade silicon. This is just the tip of the iceberg, as further refinement is necessary to achieve the high purity levels required for efficient solar cell production.
Once silicon is purified, it undergoes crystallization. Two primary methods are employed, namely the Czochralski process and the float-zone process. The Czochralski method involves melting silicon and using a seed crystal to slowly pull out a solid, crystalline ingot. This ingot is then sliced into thin wafers. The size and thickness of these wafers significantly influence cell efficiency.
If you dive deeper, the quality of these wafers is paramount. A smooth surface and uniform thickness can reduce defects, enhance light absorption, and improve overall performance. Moreover, advancements in casting techniques have resulted in larger wafers, which offer greater efficiency rates. However, manufacturing larger wafers also introduces challenges, such as increased waste and processing costs.
Cell Assembly Techniques
Cell assembly techniques mark the transition from raw silicon wafers to functioning solar cells. This step is where the real magic happens. The assembly process typically starts with applying a thin layer of anti-reflective coating to silicon wafers. This coating serves multiple purposes; primarily, it minimizes light reflection, allowing more sunlight to penetrate the cell.
After coating, metallization is performed. During this phase, metallic contacts (often made of silver) are applied on the front and back sides of the cells. This is crucial for collecting and conducting electric current generated by the photovoltaic effect. The extremily thin lines are designed to minimize shadowing on the cell surface while ensuring efficiency in electricity flow.
Once individual cells are prepared, they can be combined into larger modules. This is typically achieved through interconnecting these cells in series and parallel to achieve the desired voltage and power output. The encapsulation process follows, which involves sealing the cells with protective layers to guard against environmental factors. It’s a tricky balancing act—too tight can impede thermal expansion, while too loose can let moisture in, creating long-term issues.
Quality Control Measures
Quality control is a non-negotiable aspect of solar cell manufacturing that ensures the products are not just effective but also reliable over their intended lifespan. In a nutshell, it’s about making sure everything is up to par at every step of production.
Tests are conducted during critical stages, such as examining silicon wafer integrity and performance under sunlight. Electrical tests measure the efficiency of both individual solar cells and complete modules, where parameters such as current-voltage characteristics are scrutinized.
Moreover, manufacturers use thermal imaging to detect hotspots that could indicate potential failure points, critical for ensuring the longevity and safety of solar installations. Even after assembly, rigorous accelerated life testing helps predict future performance, simulating years of wear in a matter of weeks.
Quality control doesn't merely ensure performance, it builds trust with consumers and investors. When stakeholders can clearly see a commitment to quality, they are more inclined to invest their time and resources in solar technology.
"In the solar industry, quality isn’t just an option; it’s a requirement for sustainability and reliability."
"In the solar industry, quality isn’t just an option; it’s a requirement for sustainability and reliability."
In summary, the manufacturing processes of silicon wafer production, cell assembly techniques, and stringent quality control measures are interlinked to create solar cells that are not only efficient but also durable and trustworthy. Understanding these aspects goes a long way in comprehending the dynamic nature of solar technology and its potential to transform our energy landscape.
Environmental Considerations
Environmental considerations in the production of solar cells play a significant role in shaping the future of renewable energy. As the world pivots to greener technologies, understanding the environmental impact of solar cell manufacturing is paramount. Not only does this process involve various industrial practices, but it also affects natural resources and ecosystems, underscoring the need for a holistic view. Analyzing these factors can lead to better sustainability guidelines and more informed decisions in the energy sector.
Lifecycle Analysis of Solar Cells
Lifecycle analysis of solar cells is crucial for assessing their environmental footprint from production to disposal. This concept covers several key stages:
- Raw Material Extraction: The extraction of materials like silicon and rare elements such as gallium and indium often involves mining, which can degrade land and affect biodiversity. It’s essential to evaluate the ecological impact of these activities.
- Manufacturing: The processes used to create solar cells can be energy-intensive, particularly in the production of silicon wafers. This stage also includes potential emissions and waste production, necessitating efficient manufacturing techniques and recycling options in order to mitigate environmental damages.
- Utilization and Operation: After installation, solar panels have minimal direct emissions. However, their overall efficiency and longevity significantly impact their environmental viability. Poorly designed or maintained systems can lead to inefficiency, requiring more panels to meet energy needs, thereby increasing the overall footprint.
- End-of-Life Management: How solar panels are disposed of or recycled at the end of their lifespan is perhaps the most critical factor in lifecycle analysis. Dismantling panels without proper recycling methods can lead to toxic waste, undermining the ecological benefits they provide during usage.
By establishing a thorough lifecycle analysis of solar cells, manufacturers and policymakers can highlight the potential areas for improvement, push for cleaner technologies, and minimize negative environmental consequences.
Impact on Land and Water Resources
The production and installation of solar cells carry significant consequences for land and water resources, necessitating careful consideration.
- Land Use: Large-scale solar farms require considerable land, potentially leading to habitat disruption. The competition for land between solar energy projects and agricultural or natural spaces raises critical questions about land-use priorities. Strategies such as dual-use farming, where solar panels co-exist with crops, can mitigate these concerns and enhance land efficiency.
- Water Consumption: Water is an essential resource in both the production of solar cells and their maintenance. Every stage, from cooling in manufacturing processes to cleaning installed panels, entails water usage. Some solar energy systems, particularly concentrated solar power (CSP), necessitate significant water for cooling, which draws from nearby sources, raising the stakes in arid regions where water scarcity poses a risk.
- Soil Integrity: The construction of solar farms can alter soil structure and composition. Compaction from heavy machinery can impede water infiltration, reduce soil biodiversity, and compromise agricultural productivity. Practices that promote soil health during the installation process are essential to maintain ecological balance.
In summary, understanding the impact of solar cell production on land and water resources is vital to creating more sustainable practices. Addressing these concerns ensures that solar energy contributes to a healthier environment rather than inadvertently exacerbating existing problems.
Economic and Social Factors
The intersection of economics and social factors in the realm of solar cell production is paramount. Solar energy is not just a technological concern but also a catalyst for change within communities and economies across the globe. As societies increasingly turn to renewable sources of energy, solar cell production emerges as a significant player both in markets and in social trends. The importance of understanding these economic and social dimensions can't be overstated; they reveal how solar technology can contribute to a sustainable future.
Market Trends and Growth Potential
One of the most compelling angles in the economic aspect of solar cell production is the market trend toward increasing adoption of solar energy. According to the International Energy Agency, solar power generation has more than tripled in the last decade. This growth can be attributed to several factors, including declining costs of solar technology, public policies favoring renewables, and a growing understanding of the environmental benefits.
- Cost Reductions: The cost of solar panels has plummeted, making solar energy accessible to a broader audience. This downward trend is crtitical for both consumers and businesses who are considering making the switch from traditional energy sources.
- Government Incentives: Many governments provide incentives like tax credits and rebates for solar installations. These policies encourage wider consumer adoption and justify investments in solar technology.
- Corporate Investments: Major corporations are jumping on the solar bandwagon, seeking to reduce their carbon footprint. Companies like Google and Apple have committed to using 100% renewable energy, creating competitive pressure in various industries.
Furthermore, innovation plays a crucial role in the growth potential of this industry. New technologies such as bifacial solar cells or integrated photovoltaic systems expand the capabilities and applications of solar energy, creating even more market opportunities.
Job Creation and Workforce Development
The shift to solar energy isn't just an environmental win; it also has significant social ramifications, particularly in job creation and workforce development. As the demand for renewable energy surges, so does the need for skilled workers in the solar industry. Recent studies indicate that jobs in the solar sector are growing at a far faster rate than those in traditional energy sectors.
- Diverse Opportunities: The solar industry offers a variety of jobs ranging from engineering and manufacturing to sales and installation. This is not limited to high-skilled trades; many positions can be filled by those with training at community colleges or vocational schools.
- Training Programs: Various organizations and educational institutions are now developing training programs to meet the skills gap in this rapidly evolving industry. These programs equip workers with the necessary skills, making them competitive in a job market that increasingly values green technology.
- Community Impact: Job creation in the solar sector positively affects local economies. Often these jobs are in areas that have historically depended on fossil fuels, providing new opportunities for work and revitalizing communities.
"As solar energy continues to penetrate the market, the potential for job growth within the industry becomes a beacon of hope for a sustainable economic future."
"As solar energy continues to penetrate the market, the potential for job growth within the industry becomes a beacon of hope for a sustainable economic future."
Challenges in Solar Cell Production
The solar cell industry, while promising, is not without its hurdles. Understanding these challenges is crucial for anyone keen on the mechanisms and impacts of solar cell production. After all, addressing these obstacles often determines the efficiency, cost-effectiveness, and ultimately the adoption of solar technology. Key components of this section will delve into resource scarcity, supply chain issues, and the technological barriers that hinder advancements in solar cell efficiency.
Resource Scarcity and Supply Chain Issues
Resource scarcity looms large in the production of solar cells. Silicon, the backbone of most solar technologies, seems abundant; however, the different grades needed for solar panels require a specific quality that is limited. Moreover, materials like gallium and germanium, vital for certain high-efficiency cells, face supply challenges due to their rarity and the complexity involved in extraction.
Simply put, the more demand for solar cells rises, the more pronounced these resource scarcity issues become. The reality is that every part of the supply chain plays a role—from the mining of raw materials to the distribution of finished products. A notable example can be seen in the solar supply chain disruptions caused by geopolitical tensions, which often affect the availability of critical minerals. Companies are increasingly on the lookout for alternative sources or materials to hedge against these risks
- Mining Impact: Increased mining activities for resources can lead to environmental degradation, further complicating the eco-friendly narrative of solar energy.
- Economic Significance: As production cannot keep up with soaring demand without a stable supply chain, costs may skyrocket, making solar energy less competitive against fossil fuels.
To mitigate these issues, the industry must invest in recycling technologies and innovative extraction methods that focus on sustainability rather than sheer volume.
Technological Barriers to Higher Efficiency
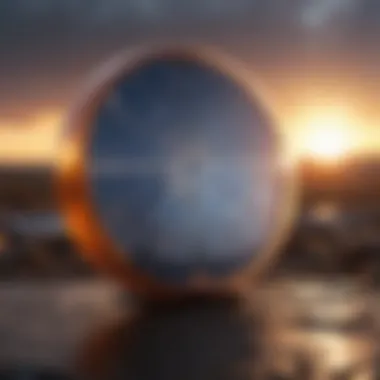
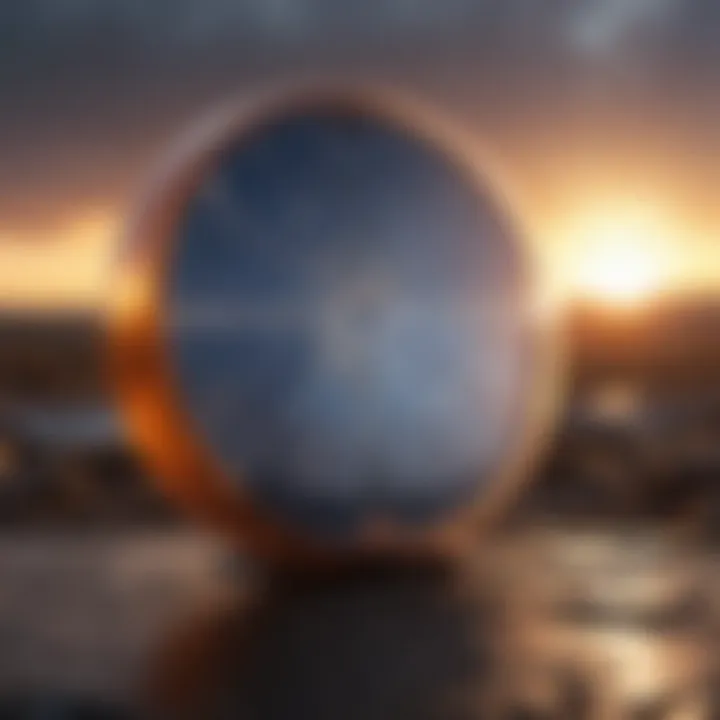
Technological hurdles can be as daunting as resource scarcity. Solar cells must convert sunlight into electricity efficiently, yet several roadblocks impede advancements. The efficiency of solar cells is measured by their ability to convert sunlight into usable energy, and while record-breaking efficiencies have emerged in laboratory settings, scaling these technologies commercially remains problematic.
One major barrier is the limitation of current materials. Traditional silicon solar cells, although widely used, have theoretical efficiency caps. To push past these barriers, the industry is exploring next-gen materials like perovskite and tandem cells. However, challenges abound, such as stability, longevity, and degradation under real-world conditions.
- Stability Concerns: Perovskite materials exhibit phenomenal efficiency ratios, but their performance usually deteriorates under harsh weather or moisture conditions.
- Cost of Innovation: Research and development of new technologies require substantial investment. Many companies hesitate, stuck between needing to invest in future technologies and the pressure to produce profit now.
"If we don't confront these technological barriers today, we'll find ourselves stuck with yesterday's solutions while the world moves on without us."
"If we don't confront these technological barriers today, we'll find ourselves stuck with yesterday's solutions while the world moves on without us."
As advancements are made, collaboration between scientists, manufacturers, and educators is critical; these stakeholders must work together to break down silos and foster an environment ripe for creative breakthroughs. By addressing the resource and technological issues, the industry can enhance solar cell output and drive down costs, paving the way for wider adoption and a more sustainable future.
Innovations Driving the Solar Industry
The solar industry is not a stagnant entity; rather, it evolves continuously, driven by innovation that fuels both efficiency and sustainability. This section examines how creativity and technology merge to enhance solar cell performance and integration into our daily lives. Understanding these innovations is crucial for students, researchers, educators, and professionals looking to grasp the dynamic nature of renewable energy solutions. The benefits range from improved energy extraction to multifunctional applications that blend seamlessly into existing architecture.
Advancements in Bifacial Solar Cells
Bifacial solar cells represent a significant leap in solar technology. Unlike traditional solar panels that only capture sunlight from one side, bifacial cells are designed to harness sunlight from both sides. This capacity allows them to capture reflected light from the ground or surrounding surfaces, effectively increasing their energy production.
- Benefits of Bifacial Technology:
- Enhanced Energy Yield: Studies suggest that bifacial solar panels can produce up to 20% more energy than their monofacial counterparts.
- Durability and Longevity: Bifacial cells typically have a longer lifespan and better performance in low-light conditions.
- Environmental Impact: The increased efficiency means fewer panels are needed to produce the same amount of energy, thereby saving materials and reducing landfill waste.
Citing a real-world example, some large solar farms have begun integrating bifacial technology, boosting their output without expanding their footprint.
Adopting bifacial systems may require an adjustment in installation strategies and site selection to maximize the benefits, but the outcomes justify the effort. These innovations encourage a more effective use of land and highlight solar energy's adaptability in various environments.
Integration with Building Materials
The integration of solar technologies with building materials is not just a trend; it's a necessity for sustainable urban development. This approach allows for the use of solar cells in everyday structures, turning passive architecture into an active participant in energy generation. Known as Building-Integrated Photovoltaics (BIPV), this method has a multitude of applications.
- Notable Benefits of BIPV:
- Aesthetics: Solar panels can be embedded in building facades, windows, and rooftops, offering energy solutions without compromising design.
- Space Efficiency: By utilizing existing surfaces, BIPV reduces the need for additional land dedicated to solar farms.
- Cost-Effectiveness: While the initial investment may be higher, BIPV can lower long-term energy costs and increase property value.
In cities worldwide, new architectures are incorporating solar technology into their design. For instance, structures fitted with solar glass not only provide natural light but also generate power that can be redirected for community use. This dual function exemplifies how solar innovations enhance urban living and reduce dependence on fossil fuels.
"Innovation is not just about inventing new technology; it's about finding smarter ways to make existing resources work for us."
"Innovation is not just about inventing new technology; it's about finding smarter ways to make existing resources work for us."
Considering the transition towards greener cities, the integration of solar materials into architecture isn't merely an option—it's a pivotal pathway towards reducing urban carbon footprints. As both bifacial solar cells and BIPV technologies continue to evolve, the future of solar energy looks increasingly promising.
Future Prospects for Solar Technology
The future of solar technology hinges on several pivotal elements that promise to reshape the energy landscape in the years to come. It is not just about generating power from rays of sunshine; it's about integrating solar seamlessly into our daily lives and those future innovations could not only enhance efficiency but also reduce costs. The ongoing development of more effective solar cells heralds a new dawn in renewable energy, making solar an indispensable player in global energy portfolios.
The Role of Solar in a Renewable Energy Portfolio
Solar energy will continue to carve out its niche in the renewable energy mix. In particular, the role of solar is increasingly recognized as crucial not only for sustainability but also for energy independence as nations strive to cut down on fossil fuels and reduce greenhouse gas emissions.
- Diversification of Energy Sources: Solar energy adds diversity to the energy mix. Its availability and potential for generation can fill in gaps when other renewable sources like wind and hydro are less productive.
- Grid Stability: Integrating solar into the grid helps stabilize energy supply. It can complement the fluctuations from other renewable sources, which are often weather-dependent.
- Local Economy Boost: By investing in solar technology, communities can create jobs in installation, maintenance, and manufacturing, fostering economic growth and independence.
- Decentralized Energy Generation: Solar panels can be installed on homes, fields, and rooftops, reducing the need for extensive transmission networks and enhancing energy resilience.
It's clear that as solar technologies continue to evolve, their contribution to a balanced and robust renewable energy portfolio will be more significant.
Potential for Technological Breakthroughs
The horizon of solar technology is rich with potential breakthroughs. Innovations that focus on improving efficiency and integrating solar solutions within everyday environments could dramatically change how we perceive and utilize energy.
- Higher Efficiency Cells: Ongoing research into materials, like perovskites and tandem solar cells, is expected to yield significant efficiencies, reaching beyond the conventional limits of silicon.
- Energy Storage Solutions: One major barrier for solar uptake has been energy storage. Advancements in battery technology could allow for greater energy retention, making solar power usable when the sun doesn't shine.
- Building-Integrated Photovoltaics (BIPV): These technologies represent a shift where solar cells are incorporated directly into building materials, eliminating the need for separate installation processes and maximizing energy collection.
- Smart Solar Grids: By employing AI and machine learning technologies, future solar grid systems will be capable of intelligently managing energy flows, predicting demand, and improving overall system efficiencies.
The potential for breakthroughs can ignite not only technological advances but also a cultural shift toward more sustainable energy practices. With these prospects in the pipeline, solar technology is poised to play a transformative role in the climate-change battle and in renewable energy transitions around the globe.
"As we look toward the future, solar energy can illuminate the path to sustainable progress, driving global change one photon at a time."
"As we look toward the future, solar energy can illuminate the path to sustainable progress, driving global change one photon at a time."
As society navigates the complexities of energy consumption and environmental stewardship, the advancements in solar technology are set to become instrumental in carving out a sustainable path forward.
The End
The completion of our exploration into solar cell production underscores its critical importance in the context of modern energy challenges. Understanding the mechanisms involved in solar cell manufacturing not only illuminates the complexities of the technology but also highlights its potential impact on sustainable development and greenhouse gas reduction. The information presented throughout this article reveals that solar technology is not merely a passing trend but a foundational pillar in the transition toward a more sustainable energy future.
Summary of Key Insights
In summarizing the insights gained from this exploration, key points emerge:
- Technological Foundations: Solar cells operate on the principles of the photovoltaic effect, converting sunlight directly into electricity. This fundamental physics is pivotal in understanding how energy conversion occurs efficiently.
- Material Considerations: The choice of materials like silicon, gallium arsenide, and emerging alternatives such as perovskite plays a crucial role in determining the performance and cost-effectiveness of solar cells.
- Environmental Implications: A comprehensive lifecycle analysis of solar cells reveals their relatively low environmental impact when compared to fossil fuels, contributing to cleaner air and less dependency on nonrenewable sources.
- Economic Factors: The solar sector presents significant growth opportunities, influencing job creation and workforce development, especially in regions transitioning from traditional energy sources to renewable technologies.
- Future Outlook: Continuous innovations in solar technologies, such as bifacial cells and integration with building materials, bring forth the possibility of greater efficiency and wider application scenarios.
Final Thoughts on Solar Cell Technology
Reflecting on the future of solar cell technology, it becomes apparent that its evolution is not solely a technological issue but a socio-economic one. The advancements in solar energy production necessitate collaboration across various sectors, including education, policy-making, and industry partnerships.
The ongoing efforts to enhance the efficiency of solar cells and reduce production costs are of paramount importance. As the technology matures, its integration into daily life will likely increase, influencing everything from urban planning to individual energy consumption. One must also consider the ethical implications and the importance of equitable access to solar technologies across different demographics.
As we stand at a pivotal juncture in the energy revolution, the continued investment in research and development will prove crucial. With favorable policies and public acceptance, solar cell technology has the potential to not only meet global energy needs but also to protect the environment for future generations.
"The sun does not wait for the perfect moment; it shines regardless. Likewise, humanity must take action and invest in solar technology to secure a sustainable future."
"The sun does not wait for the perfect moment; it shines regardless. Likewise, humanity must take action and invest in solar technology to secure a sustainable future."
Overall, the importance of solar cell production extends far beyond its mechanisms. Its implications reach into every facet of modern life, paving the way for a greener, more sustainable world.