Understanding Active Oxygen: Roles and Research Insights
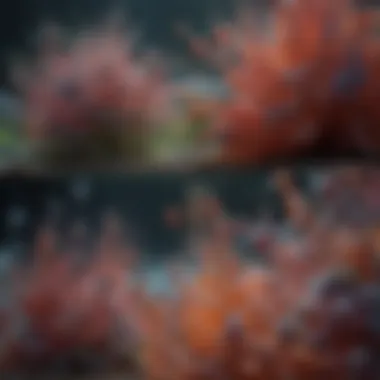
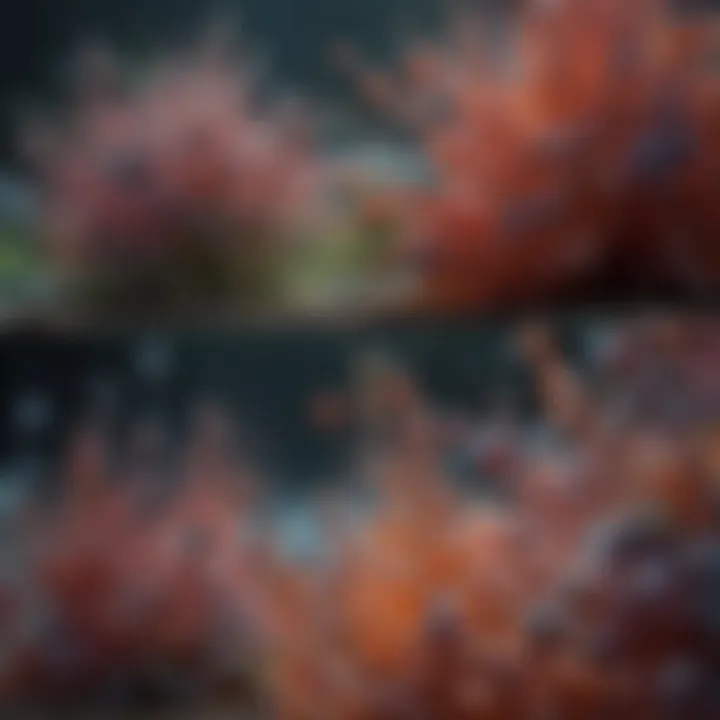
Intro
Active oxygen, often associated with the phrases like "reactive oxygen species" (ROS), looms large in discussions about biological processes and health implications. This multifaceted topic intertwines with a host of biochemical pathways, presenting both an intricate puzzle and a beacon of opportunities in several scientific fields. Understanding active oxygen is like unveiling a tapestry that, at first glance, seems chaotic but, upon closer inspection, reveals a structured narrative.
Throughout this article, we'll unravel the threads binding together the significance of active oxygen, lean into the nitty-gritty of its roles within cellular activities, and elucidate how it can both aid and harm the very systems it participates in. From its biochemical roots in oxidative stress to its pivotal roles in therapeutic applications, we'll dive deep into this essential aspect of modern science.
Prolusion to Active Oxygen
Active oxygen, often overlooked in the grand narrative of biological processes, plays a pivotal role in the intricate tapestry of cellular life. Understanding this topic is akin to finding the missing piece of a complex puzzle, one that reveals how life sustains itself amid constant chemical interactions. It dives deep into not just the components involved, but also the very essence of energy transfer and metabolic functions. Emerging evidence suggests that active oxygen forms—collectively known as reactive oxygen species (ROS)—are crucial for both maintaining homeostasis and mediating cellular responses to various stressors.
Defining Active Oxygen
Active oxygen refers to a range of reactive molecules formed from oxygen. These species are characterized by an unpaired electron, making them highly reactive and capable of interacting with other biological molecules, such as lipids, proteins, and DNA. A key point here is the distinction between these reactive species and stable oxygen molecules; active oxygen is fundamentally different in terms of its chemical behavior and effects on living systems. This reactivity can lead to beneficial outcomes, such as pathogen elimination, while also posing risks, particularly when produced in excess.
Types of Reactive Oxygen Species
Understanding the various types of reactive oxygen species enriches our appreciation of how they influence biological systems. These species range from harmless to potentially deleterious.
Singlet Oxygen
Singlet oxygen, a high-energy form of molecular oxygen, plays a distinct role in biological systems. Its contribution isn't limited to being merely a curious phenomenon; it's central to processes such as photosynthesis and various signaling cascades. What sets singlet oxygen apart is its unique electronic configuration, which allows it to participate in reactions that other forms of oxygen cannot. It can inflict damage on cellular components, leading to oxidative stress, thus presenting both benefits in controlled amounts and significant risks in excess. In this article, singlet oxygen is notable for how it bridges beneficial and harmful effects, adding layers to our understanding of oxidative processes.
Superoxide
Superoxide is often viewed as a double-edged sword—helpful in moderate quantities, yet toxic when overproduced. Its ability to act as a signaling molecule is noteworthy, playing a part in pathways that regulate cell growth and respond to stimuli. The key characteristic of superoxide is its tendency to convert into more reactive species, which facilitates further reactions that might lead to damage if not regulated. This renders superoxide a popular choice in discussions about oxidative processes, showcasing the fine line between health and disease that it navigates.
Hydrogen Peroxide
Hydrogen peroxide is another substantial player in the active oxygen family. Its stability allows it to diffuse across membranes, where it can exert effects to modulate cellular functions—a unique advantage compared to more reactive species. Often seen as a signalling molecule, hydrogen peroxide has a lesser-known role in host defense mechanisms. However, its greater concentrations can lead to significant oxidative damage. It is embraced in both environmental and medical contexts, making it an ideal focus in this discussion.
Hydroxyl Radical
The hydroxyl radical is often cited as one of the most reactive species among all oxygen derivatives. It acts quickly in biological systems, making it an exceedingly powerful oxidant. Its role in damaging macromolecules is well-documented, leading to mutations and cellular dysfunction. Thus, it is accurate to describe hydroxyl radicals as both beneficial in regulated signaling and detrimental in excessive amounts—hence its importance in the conversation surrounding active oxygen. Hydroxyl radicals exemplify the theme of duality in active oxygen's role, aligning closely with the overarching narrative of balance in biochemical processes.
"Active oxygen stands at the crossroads of life and damage, revealing the delicate balance that lies at the heart of cellular function."
"Active oxygen stands at the crossroads of life and damage, revealing the delicate balance that lies at the heart of cellular function."
The exploration of these different species of reactive oxygen is vital. It not only highlights their distinct behaviors but also emphasizes the intricate web of biochemical interactions that make life possible. In subsequent sections, we will further dissect these implications and applications in biological systems, allowing for a broader understanding of active oxygen's true nature and potential.
Biochemical Mechanisms of Active Oxygen
The study of active oxygen forms a crucial aspect within the scientific dialogue surrounding bioenergetics and cell physiology. Understanding the biochemical mechanisms that lead to the formation and regulation of reactive oxygen species (ROS) illuminates their complex roles in sustaining life and maintaining cellular homeostasis. The duality of these molecules as both signaling agents and potential cellular aggressors makes their examination essential for grasping both health and disease states.
Formation of Reactive Oxygen Species
Natural Processes
Natural processes underpin the formation of reactive oxygen species in living systems. These include mechanisms like photosynthesis, where chlorophyll captures sunlight to convert carbon dioxide and water into glucose and oxygen. A key characteristic of natural processes is their efficiency in generating energy while producing ROS as byproducts. This integration is beneficial for sustaining ecological balance; as cells metabolize, they produce ROS that can signal for necessary adjustments in cellular function and metabolism.
Among the unique features of these processes is their self-limiting characteristic. In an optimal cellular environment, the body has developed defenses concurrently to stabilize ROS levels. Antioxidant mechanisms, such as enzymatic degradation and scavenging, showcase an advantage as they prevent oxidative damage while still allowing for the functional roles that ROS play. However, when there’s an imbalance—increased ROS production versus antioxidant capacity—cells may experience oxidative damage, potentially leading to diseases.
Anthropogenic Sources
On the other hand, anthropogenic sources of reactive oxygen species arise from human activities, such as industrial emissions, automobile exhaust, and the combustion of fossil fuels. This kind of pollution represents a significant concern due to its direct contribution to elevated ROS levels in the environment, ultimately impacting human health and ecosystems. Urbanization typically amplifies these effects, making the understanding of anthropogenic contributions vital.
A notable characteristic of these sources is their capacity for indiscriminate proliferation of ROS. Unlike the regulated formation seen in natural processes, anthropogenic sources unleash ROS without the built-in mechanisms for homeostasis. This uncontrolled release poses risks, leading to chronic conditions like respiratory diseases and cardiovascular issues. The downside to these sources primarily lies in their capacity to induce oxidative stress on a broader scale, affecting both individual health and environmental quality.
Role in Cellular Metabolism
Energy Production
When examining active oxygen’s role in cellular metabolism, energy production stands out as a fundamental aspect. Active oxygen forms are intricately linked to mitochondrial function, where ATP—the energy currency of the cell—is generated. This process, known as oxidative phosphorylation, depends on the electron transport chain, which inherently produces reactive oxygen species as intermediate byproducts.
A key characteristic worth noting is the duality of produced energy and reactive molecules. The efficiencies gained from energy production are noticeably vital for cellular operations, but the simultaneous generation of ROS necessitates a balance. An effective way that ROS contributes positively is through mitochondrial biogenesis, thus enhancing cellular energy production over time. Nonetheless, if the ROS become excessive, the fine line between beneficial signaling and harmful oxidative stress gets blurred, pointing to potential cellular damage.
Signal Transduction
Signal transduction is another pivotal area where active oxygen plays a role. It involves the transmission of chemical signals from the cell’s exterior to its interior, leading to appropriate responses. ROS serve as second messengers in various signaling pathways, crucial for processes like cellular growth and apoptosis. Highlighting the characteristic of versatility, these reactive species act dynamically, enabling cells to respond to external cues effectively.
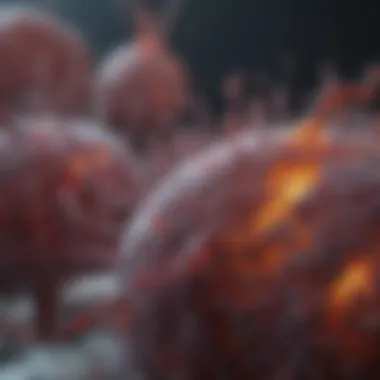
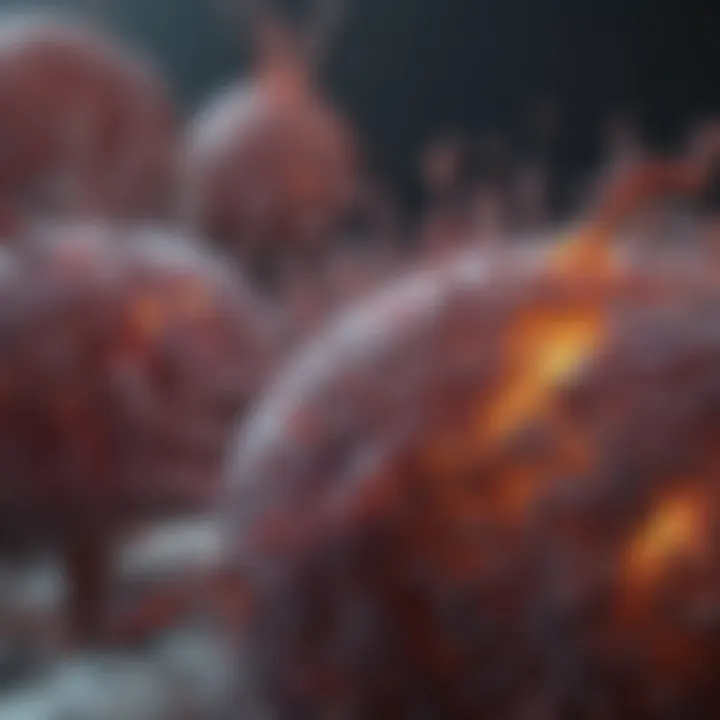
The specific connection between ROS and signal transduction reveals profound implications for cellular decision-making. ROS can alter protein functions, thus modifying signaling cascades. This adaptability is beneficial for cells, allowing for quick adjustments to their internal state or external environment. Despite these advantages, one must acknowledge the risks associated with aberrant signaling caused by excessive ROS, leading to programmed cell death or unregulated proliferation, paving the way for potential diseases.
"Understanding the balance between the beneficial and harmful roles of reactive oxygen species is key to unlocking the mysteries of cellular metabolism."
"Understanding the balance between the beneficial and harmful roles of reactive oxygen species is key to unlocking the mysteries of cellular metabolism."
Active Oxygen in Biological Systems
Active oxygen plays a pivotal role in biological systems, affecting various cellular processes. While they are crucial for normal functioning, these reactive oxygen species have a dual nature — they can be beneficial in moderation but detrimental in excess. Understanding their balance is key to comprehending their implications in health and disease.
Cellular Functions of Active Oxygen
Antimicrobial Activity
One of the prime aspects of active oxygen's role in biological systems is its antimicrobial activity. This character of reactive oxygen species, particularly hydrogen peroxide and superoxide, serves as a defense mechanism against pathogens. When immune cells encounter bacteria, they can generate these reactive species to obliterate the invaders. What makes this aspect even more fascinating is that many pathogens have not yet developed robust mechanisms to defend against these reactive molecules, thereby making them an effective weapon in the immune arsenal.
The unique feature of antimicrobial activity lies in its ability to promptly respond to infections, offering an advantage in combating opportunistic pathogens. However, if not properly regulated, this active oxygen can also harm surrounding healthy cells, leading to complications such as oxidative stress. In sum, while this activity is a valuable tool, the fine line between effective defense and damaging oversight is razor-thin, and that’s a consideration that anyone in the field should heed.
"Active oxygen is like a double-edged sword; its effectiveness in fighting infections can quickly turn into a detrimental force if not diligently controlled."
"Active oxygen is like a double-edged sword; its effectiveness in fighting infections can quickly turn into a detrimental force if not diligently controlled."
Cell Signaling
Another significant aspect of active oxygen in biological systems is cell signaling. Reactive oxygen species play an integral role as signaling molecules that modulate various physiological responses. They participate in processes like cell proliferation, differentiation, and apoptosis. Essentially, they facilitate communication within the cell, orchestrating a symphony of activities that maintain homeostasis.
A noteworthy characteristic of cell signaling is its versatility — reactive oxygen can either promote or inhibit biological processes depending on their concentration and context. In other words, they act much like a dial, with subtle tweaks resulting in vastly different outcomes. One advantage of this mechanism is the potential for targeted therapeutic interventions; by regulating signaling pathways influenced by active oxygen, we might better handle diseases linked to cellular dysfunctions. On the flip side, excessive or poorly regulated signaling can lead to pathological conditions, making it an intricate balance to maintain.
Oxidative Stress Mechanisms
Oxidative stress refers to an imbalance between reactive oxygen species production and the body’s ability to detoxify them or repair the resulting damage. Understanding its mechanisms unveils significant insights into various health conditions, including chronic illnesses.
Sources of Oxidative Stress
Oxidative stress emerges from a multitude of sources, encompassing both internal and external factors. Internally, normal metabolic processes yield reactive oxygen species, while factors like inflammation and certain health conditions can heighten their production. Externally, pollution, radiation, and toxic substances play key roles, bombarding our biological systems with excess active oxygen.
The key characteristic of these sources is their ubiquitous nature; they are ever-present in modern life. A careful grasp of these sources gives us a comprehensive view of why oxidative stress is becoming a leading factor in many diseases today. By identifying and mitigating these sources, we can take proactive stances toward health preservation.
Cellular Damage
The detrimental effects of excessive reactive oxygen species culminate in cellular damage. This damage manifests in various forms, such as lipid peroxidation, DNA damage, and protein modification, often leading to cell death or dysfunction. With components of our cellular machinery under siege, the implications for human health are monumental, paving the way for diseases like cancer, neurodegenerative disorders, and cardiovascular issues.
The unique feature of cellular damage is its cascading effect; once a cell gets compromised, the ripple effect can extend to neighboring cells, creating widespread issues. Recognizing this enables researchers and clinicians to understand disease mechanisms in more depth, leading to targeted strategies for intervention and prevention.
The Dual Nature of Active Oxygen
The role of active oxygen in biological systems is an intricate double-edged sword. While reactive oxygen species (ROS) can serve vital roles in cellular functions, they also possess the potential to wreak havoc when present in excess. Understanding this dual nature is essential for recognizing how active oxygen participates in both beneficial and detrimental processes within organisms.
Benefits of Reactive Oxygen Species
Defense Mechanisms
One of the significant contributions of ROS lies in their defense mechanisms. When pathogens invade, the immune system taps into the potency of reactive oxygen species to mount an attack. For instance, phagocytic immune cells utilize ROS to oxidize and destroy harmful bacteria in a process known as oxidative burst. This characteristic of offensive action is why such mechanisms are widely seen as an effective response to infections.
What sets these defense mechanisms apart is their speed and precision. ROS can rapidly react with various biomolecules, knocking out threats before they can cause significant damage. However, the double-edged nature emerges here; excessive ROS can damage not only pathogens but also nearby healthy cells, leading to inflammation and tissue damage. This adds a layer of complexity to their role, showcasing the need for a balance.
Cell Growth Regulation
In another realm, ROS are also crucial for cell growth regulation. They act as signaling molecules that can regulate various aspects of cellular function, including growth and differentiation. Here, the key characteristic is their ability to modulate pathways that direct cells to grow or divide. This is particularly important in wound healing, where active oxygen helps orchestrate cellular responses to repair damaged tissues.
Notably, the unique feature of cell growth regulation by ROS lies in their capability to raise the cellular alertness to growth signals. When cells perceive ROS, they ramp up their response mechanisms, leading to optimal growth conditions. However, the potential downsides cannot be ignored. An imbalance, favoring ROS, can lead to tumorigenesis, as cells may start growing uncontrollably. Thus, while ROS are beneficial in moderation, their mismanagement can tip the scales toward malignancy.
Risks Associated with Excessive Active Oxygen
Chronic Diseases
On the flip side, high levels of ROS have been linked to a variety of chronic diseases. The oxidative stress induced by excessive reactive oxygen species can damage cellular structures, including lipids, proteins, and DNA. This is an alarming situation because oxidative damage is implicated in diseases like cancer, Alzheimer's, and cardiovascular disorders. The unique aspect of ROS in this context is their persistent nature, causing cumulative damage over time.
By highlighting the connection between oxidative stress and chronic ailments, we shed light on a significant concern for public health. Managing ROS levels could be a critical factor in preventing or mitigating these conditions, emphasizing the importance of understanding the balance of active oxygen in our health.

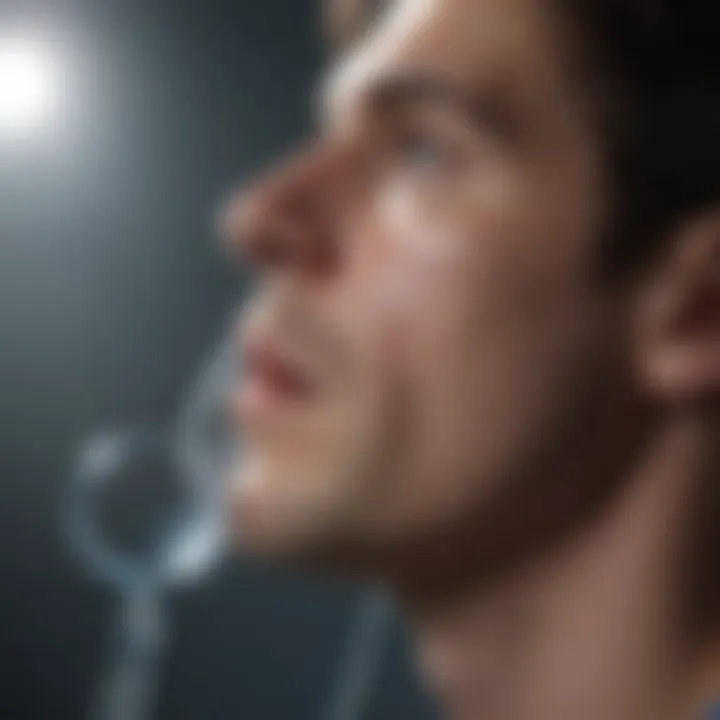
Aging
Lastly, the phenomenon of aging has a notable association with active oxygen levels. Over time, the accumulation of oxidative damage contributes to the aging process. The main characteristic here is that as organisms age, their ability to neutralize ROS diminishes. This can result in visible signs of aging, like wrinkles or frailty, as cellular integrity degrades.
The unique feature concerning aging is the reality that while some level of ROS may support cellular signaling and growth, an overabundance significantly accelerates degeneration. This underlines the importance of antioxidants in diets and lifestyle choices that can help mitigate this excess.
Thus, the connection between ROS and aging presents a compelling argument for a balanced approach toward health.
Thus, the connection between ROS and aging presents a compelling argument for a balanced approach toward health.
In summary, the dual nature of active oxygen highlights that while it serves essential roles in defense and growth, it can just as easily be the antagonist in chronic disease and aging. Understanding both sides of this coin is critical for advancements in therapeutic approaches and improving overall well-being.
Active Oxygen in Medicine
Active oxygen is gaining ground in medical applications due to its significant impact on diagnosis and treatment methodologies. By harnessing the power of reactive oxygen species, medical science is exploring innovative paths for combating diseases. These advancements can lead to better health outcomes but are not without their complexities and dangers, which we will explore further.
Therapeutic Uses of Reactive Oxygen Species
Cancer Therapy
One of the most prominent applications of reactive oxygen species lies in their use in cancer therapy. Researchers are investigating how these species can selectively target and kill cancer cells while sparing normal cells. This is crucial as traditional cancer treatments often damage healthy tissue along with malignant cells.
A key characteristic of cancer therapy utilizing active oxygen is its ability to induce oxidative stress specifically within tumor cells. This causes cellular damage that can result in apoptosis or cancer cell death. Notably, photodynamic therapy is a shining example of this. It uses light-activated compounds that produce reactive oxygen species when exposed to light, effectively targeting tumors without the systemic side effects of conventional chemotherapy.
However, while the advantages are promising – significantly less damage to healthy cells and reduced side effects – there are challenges. Ensuring that the therapy precisely targets the cancerous tissue requires further refinement. Not every type of cancer responds equally well, which creates a unique hurdle in treatment plans for clinicians.
Infection Control
Infection control utilizing reactive oxygen species also represents another impactful application in medicine. This strategy often employs germicidal techniques that can deactivate pathogens, including bacteria and viruses, through oxidative damage. Such methods are especially important in hospital settings where antibiotic resistance has become alarmingly prevalent.
The hallmark of infection control through active oxygen lies in its broad-spectrum efficacy. Unlike traditional antibiotics which may target specific types of bacteria, reactive oxygen species can address a wide range of microbial threats. One common method is using hydrogen peroxide-based solutions to disinfect surfaces and equipment, minimizing the likelihood of healthcare-associated infections.
On the downside, the application of reactive oxygen species must be carefully managed to avoid tissue damage. If not controlled appropriately, the oxidative stress that is lethal to pathogens can also be harmful to human tissues, leading to complications such as inflammation.
Risks of Targeting Active Oxygen
While the therapeutic potential of active oxygen is substantial, the medical field must tread carefully, balancing benefit against risk. This section outlines the potential side effects inherent in these treatments and the need for a delicate balance between efficacy and safety in applications.
Potential Side Effects
When discussing potential side effects in targeting active oxygen, one must consider the implications of oxidative stress. It is essential to recognize that while reactive oxygen species can serve therapeutic purposes, they also have the potential for harmful effects on healthy cells. This characteristic poses a paradox for medical practitioners.
The unique feature of monitoring potential side effects is paramount. Medical professionals should evaluate the dosage and application methods of reactive oxygen species to mitigate risks associated with excessive oxidative damage. Careful adjustment in treatment plans can reduce the chances of side effects, though it complicates the clinical approach.
Balancing Efficacy and Safety
Finding a balance between efficacy and safety is inherently challenging in medicine, especially when it involves active oxygen. Given its dual nature as both a therapeutic agent and potential aggressor, researchers must tread cautiously. For instance, while increasing the concentration of reactive oxygen species may enhance therapeutic outcomes, it may simultaneously elevate the risk of side effects.
Thus, a key characteristic of research in this area is the continuous need for a balance. Monitoring techniques and strategies, such as controlled release mechanisms or timed delivery systems, may prove beneficial in ensuring that treatments can maximize effectiveness while minimizing potential harm.
The unique aspect of pursuing this balance ensures that medical professionals maintain the integrity of treatment protocols while improving patient safety. The challenges are clear, but forward momentum in research provides encouragement for future applications as we strive to push the boundaries of current medical practices.
Environmental Impacts of Active Oxygen
Understanding the environmental impacts of active oxygen is crucial, as it sheds light on both the natural processes and human activities that drive its generation. The reactive oxygen species (ROS) that comprise active oxygen play a significant role in interactions within our atmosphere and ecosystems. This section will explore the multifaceted implications of active oxygen in atmospheric chemistry and ecosystem dynamics, outlining both benefits and potential drawbacks.
Active Oxygen in Atmospheric Chemistry
Ozone Layer Interaction
The ozone layer serves as a protective shield for the Earth, filtering out harmful ultraviolet (UV) radiation. The interaction of active oxygen with this layer is significant because it influences the health of our atmosphere. One of the striking features of this interaction is the formation and breakdown of ozone in the stratosphere.
Ozone itself is primarily composed of three oxygen atoms. In this respect, active oxygen is ongoingly at play. When pollutants such as nitrogen oxides and volatile organic compounds react in sunlight, they can lead to the generation of more ozone, albeit at ground level, where it becomes a harmful air pollutant. Thus, the ozone layer interacts with active oxygen in a dual manner; it is both a critical factor in ozone creation and a byproduct of anthropogenic activity.
This layer is crucial because it provides a much-needed shield against UV radiation, preventing widespread harm to living organisms. However, an excess of active oxygen from pollution can threaten its integrity. In essence, the key characteristic of this interaction lies in the balance of maintaining the ozone layer while mitigating the pollution that leads to its depletion.
Pollution Effects
Pollution, a pressing concern for environmental health, is deeply intertwined with the dynamics of active oxygen. The impact of pollution on active oxygen is multifaceted. On one hand, pollution contributes to the rise in reactive oxygen species, which can lead to heightened oxidative stress in the environment. On the other hand, these very species can further catalyze the deterioration of air quality.
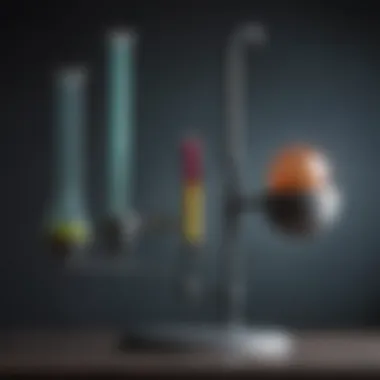
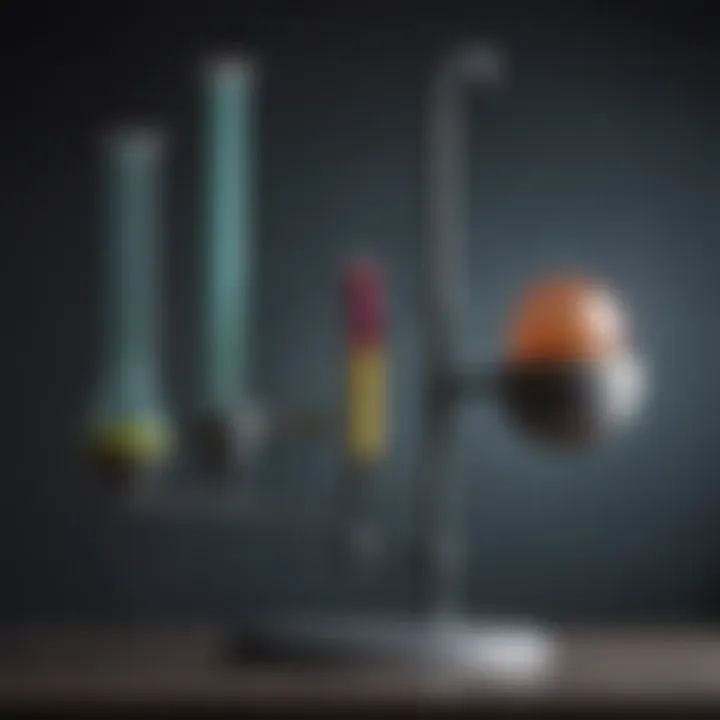
One central aspect of pollution effects is the generation of ground-level ozone, a component that is less beneficial compared to its stratospheric counterpart. Ground-level ozone contributes to respiratory problems and can harm vegetation. In this context, understanding the pollution effects of active oxygen is a practical choice for this article as it underscores the need for awareness and action to control emissions. The unique feature is that the very processes intended to produce energy and enhance human life can ultimately jeopardize our environmental health.
Role in Ecosystem Dynamics
Biogeochemical Cycles
Active oxygen contributes significantly to the biogeochemical cycles that sustain life on Earth. These cycles involve the movement of elements like carbon, nitrogen, and phosphorus through the ecosystem, where reactive oxygen species facilitate important chemical reactions. One admirable characteristic of these cycles is their ability to regenerate resources essential for living organisms.
For instance, the presence of reactive oxygen species can promote the breakdown of organic matter, which in turn releases nutrients back into the soil. This interaction illustrates the essential role active oxygen plays in maintaining balance within ecosystems. Consequently, recognizing biogeochemical cycles is vital, as they highlight how active oxygen can benefit plant and animal life while also indicating potential environmental stresses that might arise from disruption.
Plant Responses
Plants react uniquely to active oxygen in various stages of growth and development. During photosynthesis, for example, reactive oxygen species can signal pathways that lead to plant responses such as stress tolerance. An interesting aspect of plant responses to active oxygen is the way these organisms adapt to changing environmental conditions, effectively using this reactivity for their advantage.
Active oxygen can activate defense mechanisms against pathogens and herbivores, highlighting its role in plant immunity. However, too much of it may lead to oxidative stress, which can cause cellular damage and hinder growth. The dual nature of these responses emphasizes the need for balance; while active oxygen can trigger positive adaptations, excessive presence can spell disaster for plant health. This makes examining plant responses a thought-provoking aspect of the overall discussion around active oxygen.
"Active oxygen is not just a villain in the degradation of our environment; its story includes essential roles in nature's resilience and adaptability."
"Active oxygen is not just a villain in the degradation of our environment; its story includes essential roles in nature's resilience and adaptability."
In summary, the environmental impacts of active oxygen are nuanced and complex. From its interaction with the ozone layer to the dynamics within ecosystems, active oxygen presents a rich field of study that intertwines chemistry, biology, and environmental science. Understanding these impacts is key to addressing the pressing environmental issues we face today.
Research and Future Directions
Active oxygen’s intricate involvement in various spheres of life makes its study a prodigious focus in modern science. Research and future directions concerning active oxygen not only aid in understanding its biochemical behaviors, but they also unlock potential applications across multiple disciplines. As we press on to explore emerging methods and trends, the significance of this topic becomes abundantly clear. Active oxygen is more than just a biochemical element; it is a key player in medical therapies and environmental health. To fully appreciate its implications, we must examine cutting-edge detection techniques and innovative therapeutic approaches.
Emerging Trends in Active Oxygen Research
New Detection Methods
In recent times, the development of new detection methods for active oxygen has transformed our capacity to study reactive oxygen species. Enhanced imaging technologies and advanced spectroscopic techniques allow for high-precision measurements of these elusive molecules. This kind of technology is especially beneficial for analyzing biological systems, where active oxygen plays crucial roles yet is often challenging to quantify accurately.
One prominent characteristic of these emerging detection methods is their ability to operate in real-time. Unlike conventional methods that may involve cumbersome sample preparations, real-time detection facilitates immediate analysis, yielding insights that are more reflective of physiological conditions. The unique feature of in situ detection empowers researchers to track dynamic processes such as cellular oxidative stress or the response of cells to therapeutic strategies without compromising the integrity of samples.
However, the advantages come with some disadvantages. These advanced tools often require significant investment in equipment and training, potentially limiting accessibility for smaller laboratories. Despite this, their contribution to the understanding of active oxygen cannot be overstated. The nuances of reactive oxygen behaviors unravel, paving the way for informed applications in various fields.
Innovative Therapeutic Approaches
Parallel to detection advancements, innovative therapeutic approaches utilizing active oxygen are increasingly prominent. These techniques often harness the oxidative properties of reactive oxygen species to target tumor cells or bacterial infections specifically. Certain treatments have begun integrating reactive oxygen species as mediators, strategically generating oxidative stress to induce cell death in malignancies or resist pathogenic bacteria.
The key characteristic here is the precision with which these therapies can be tailored. New delivery systems, for instance, improve the specificity of treatments, minimizing collateral damage to healthy cells. As researchers explore these avenues, they examine how to maximize therapeutic benefits while reducing adverse effects, ensuring safety and efficacy. Moreover, these approaches showcase a unique feature: the incorporation of biocompatible materials that enhance the stability and controllability of active oxygen over targeted areas.
Nevertheless, there are potential drawbacks that accompany these innovative solutions. Understanding the full spectrum of effects on bodily systems and ensuring the safety of prolonged exposure to reactive oxygen species are areas still under extensive scrutiny. Overall, the potential benefits for cancer therapy and infection control indicate a fruitful pathway ahead.
Interdisciplinary Collaborations
Linking Biology and Chemistry
The intricate relationship between biology and chemistry forms the bedrock for advancements in active oxygen research. Collaborative efforts are essential in integrating knowledge across these spheres to deepen our understanding of reactive oxygen species and their impacts on living organisms. Here, synthetic biology has the potential to provide profound insights, allowing scientists to construct pathways that mimic the biological activities of active oxygen.
A key characteristic of this collaboration lies in its capacity to produce novel compounds and bioengineering solutions. By merging chemical expertise with biological applications, researchers can design new molecular targets that improve the efficacy of therapeutic agents. For instance, creating oxygen-sensitive drugs tailored for specific conditions can lead to breakthroughs in treatment efficacy. However, while interdisciplinary approaches hold promise, there’s the risk of miscommunication across fields that can hinder progress.
Environmental Studies Integration
In addition, integrating environmental studies into the discourse on active oxygen broadens our understanding of its ecological ramifications. Active oxygen plays a critical role in atmospheric chemistry and the health of ecosystems, affecting everything from pollutant degradation to oxygen cycles in aquatic environments. This inclusion of ecological perspectives fortifies the narrative around active oxygen, making it not just a biological subject, but a multifaceted issue involving environmental health.
The beneficial aspect of this integration is its capacity to inform both ecological and public health policies. Cross-references between laboratory results and environmental data can enhance forecasts of air quality or climate changes related to reactive oxygen species. Yet, it also poses challenges; the complexity of ecological systems often makes it difficult to draw straightforward conclusions. Overall, harnessing the knowledge from different disciplines drives forward a comprehensive understanding of active oxygen and its vast implications.
End
In wrapping up our examination of active oxygen, it’s important to emphasize how pivotal this topic is, not just in basic science but in many practical applications as well. The implications of reactive oxygen species (ROS) stretch from cellular functions to their potential role in medicine and environmental contexts. Understanding how active oxygen operates provides insights not only into its beneficial aspects but also the risks it poses when not properly regulated.
Recap of Key Concepts
Let’s revisit the essential concepts we have discussed:
- Defining Active Oxygen: Active oxygen encompasses various reactive oxygen species. These include singlet oxygen, superoxide, hydrogen peroxide, and hydroxyl radicals, each playing distinct roles in biological systems.
- Biochemical Mechanisms: The origins of these species stem from both natural processes, like photosynthesis, and human activities, such as pollution.
- Dual Nature: While some ROS are vital for processes like cellular signaling and defense against pathogens, an overload can lead to oxidative stress, contributing to chronic diseases and aging.
- Medical Relevance: In medicine, the therapeutic potential of active oxygen is being explored to combat cancer and infections. Yet, the balancing act of achieving efficacy without side effects remains a challenge.
- Environmental Considerations: ROS also play a critical role in atmospheric chemistry and ecosystem dynamics, affecting everything from climate to plant health.
"The comprehension of active oxygen's roles is significant not just to biologists but to interdisciplinary fields like medicine and environmental science."
"The comprehension of active oxygen's roles is significant not just to biologists but to interdisciplinary fields like medicine and environmental science."
Significance of Active Oxygen in Science
The study of active oxygen is significant in various areas for many reasons:
- Biological Impact: Understanding the roles of reactive oxygen species helps in developing treatments for diseases linked to oxidative stress, such as cancer, diabetes, and neurodegenerative disorders. It underlines the connection between our cellular processes and overall health.
- Interdisciplinary Approaches: The intersection of biology, chemistry, and environmental studies can spark innovative solutions to handle oxidative damage in both health care and ecological restoration.
- Future Research Directions: Ongoing research into detection methods and therapeutic applications ensures that active oxygen will remain an essential focus for developing advanced medical treatments and understanding environmental impacts.