Understanding Full DNA Sequencing: Principles and Implications
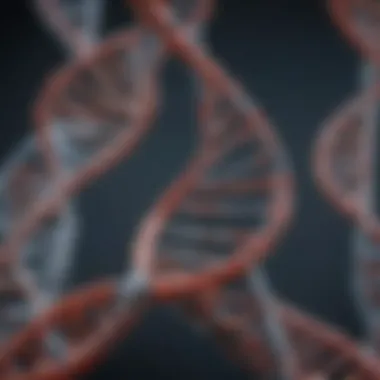
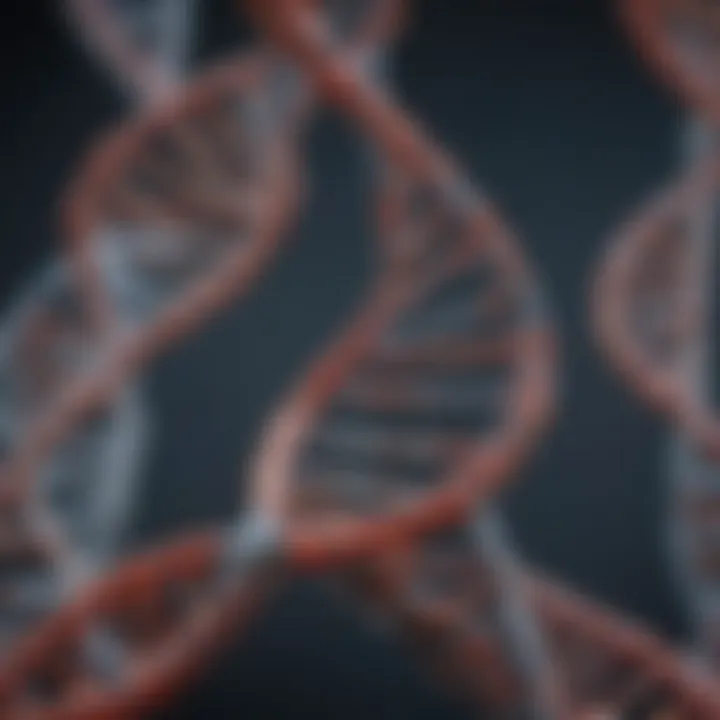
Intro
Full DNA sequencing is a transformative approach in genetics that allows for a detailed examination of the entire genetic material of an organism. Unlike targeted sequencing methodologies, which focus on specific segments of DNA, full DNA sequencing provides a comprehensive view of the genetic code. This all-encompassing technique holds vast implications for various fields, particularly in understanding health conditions, evolutionary biology, and advancements in biotechnology. As this technology continues to advance, its relevance in personalized medicine and genetic research becomes increasingly substantial.
Key Concepts
Definition of the Main Idea
At its core, full DNA sequencing refers to the process of determining the complete nucleotide sequence of an organism's DNA. This process involves analyzing both coding regions, which encode proteins, and non-coding regions that serve regulatory and structural roles. The comprehensive nature of full DNA sequencing allows researchers to gain insights into genetic variations, mutations, and their potential impacts on phenotypic traits.
Overview of Scientific Principles
The process of full DNA sequencing is grounded in various scientific principles. Central to this technology are the following key components:
- Sequencing Chemistry: This involves the use of nucleotides to create labeled fragments of DNA that can be analyzed.
- Next-Generation Sequencing (NGS): NGS technologies allow rapid sequencing of large amounts of DNA, revolutionizing the pace at which genetic data can be collected.
- Data Interpretation: Once sequencing is complete, the resulting data must be analyzed to identify variations and interpret biological significance.
"Full DNA sequencing has the potential to unlock secrets of genetics, providing insights that could lead to breakthroughs in medicine and biology."
"Full DNA sequencing has the potential to unlock secrets of genetics, providing insights that could lead to breakthroughs in medicine and biology."
This foundation of principles supports the entire process, ensuring accurate and meaningful genetic analysis.
Current Research Trends
Recent Studies and Findings
In recent years, numerous studies have emerged that utilize full DNA sequencing to explore diverse biological questions. Research spanning from cancer genomics to evolutionary biology is increasingly relying on this technique. For instance, a recent study published in Nature highlighted how whole-genome sequencing can uncover hereditary factors contributing to complex diseases, paving the way for targeted therapies.
Significant Breakthroughs in the Field
Several breakthroughs in sequencing technology have reshaped our understanding of genetics. Among these are:
- Improved Accuracy: Advances in sequencing methods have enhanced accuracy, reducing errors in identifying genetic variants.
- Cost Reduction: The cost of sequencing has dramatically decreased, making it accessible for broader research applications.
- Real-time Sequencing: Emergence of real-time sequencing allows instantaneous data analysis, which can be crucial in clinical settings.
These developments indicate a thriving field that continues to expand the boundaries of what we understand about genetics and its everyday implications.
Intro to Full DNA Sequencing
In the landscape of genetics, full DNA sequencing represents a significant advancement that reshapes our understanding of biological systems. Its importance lies not only in uncovering the blueprint of life but also in enhancing personalized medicine, improving agricultural outputs, and deepening our grasp of evolutionary biology. This article aims to elucidate the principles, techniques, and implications surrounding full DNA sequencing, presenting a comprehensive narrative for students, researchers, educators, and professionals.
Definition and Importance
Full DNA sequencing refers to determining the complete sequence of an organism's DNA, enabling the characterization of its genetic information. Unlike partial sequencing methods, full DNA sequencing provides a holistic view of the genome, revealing not just the coding regions but also the regulatory elements and non-coding regions. This approach is pivotal for several reasons:
- Precision in Medicine: Full DNA sequencing allows for identifying genetic predispositions to diseases, leading to targeted treatments tailored to individual genetic makeups.
- Insight into Biodiversity: It enhances our understanding of genetic diversity among species, crucial for conservation in ecological studies.
- Biotechnological Innovations: In agriculture, it facilitates the development of genetically modified organisms that can withstand diseases, pests, and climate challenges.
The comprehensive insights provided by full DNA sequencing foster breakthroughs across several fields and push the boundaries of genetic research.
Historical Context
The journey toward full DNA sequencing began with the discovery of the DNA double helix structure by James Watson and Francis Crick in 1953. This historical moment laid the foundation for subsequent research into DNA's role in genetics. In the 1970s, Sanger sequencing was introduced, revolutionizing the way scientists could decode genetic information. However, this method was labor-intensive and time-consuming.
The completion of the Human Genome Project in 2003 marked a turning point in genetic research. This monumental effort sequenced the entire human genome, catalyzing advancements in both sequencing technologies and bioinformatics. Subsequent innovations led to the development of next-generation sequencing (NGS) methods, drastically decreasing the time and cost of sequencing. Today, these techniques have made full DNA sequencing accessible not only in research but also in clinical settings. The evolution of these methods has made it vital for understanding genetics, shaping the future of medicine and biotechnology.
Fundamental Principles of DNA Sequencing
Understanding the fundamental principles of DNA sequencing is crucial as this foundation underpins all subsequent applications and innovations in genomics. This section unravels the core concepts that define how DNA sequencing works, highlighting the importance of DNA structure as well as the various sequencing technologies that have emerged.
DNA Structure and Function
DNA, or deoxyribonucleic acid, is the hereditary material in many living organisms. Each DNA molecule is composed of two strands that coil around each other to form a double helix. These strands are made up of simple units called nucleotides, each containing a phosphate group, a sugar molecule, and a nitrogenous base. The sequence of these bases—adenine, thymine, cytosine, and guanine—encodes genetic information.
An essential aspect of DNA's role is its function in the processes of replication and transcription. During replication, DNA makes a copy of itself before cell division, ensuring that each daughter cell receives an identical set of genetic codes. In transcription, segments of DNA are copied into RNA, which then guides the synthesis of proteins. This relationship between DNA structure and function is fundamental, as any error in these processes can lead to mutations that may have serious implications for health and disease.
Overview of Sequencing Technologies
The methodologies for sequencing DNA have evolved significantly over time. Each technology has its own distinct advantages and limitations. It is helpful to categorize them into three major types: Sanger sequencing, Next-Generation Sequencing (NGS), and Third-Generation Sequencing.
- Sanger Sequencing: Developed in the 1970s, Sanger sequencing was one of the first methods used to read DNA sequences accurately. It relies on selective incorporation of chain-terminating dideoxynucleotides. Sanger sequencing is still influential for small-scale projects, such as sequencing individual genes or verifying NGS results.
- Next-Generation Sequencing (NGS): This innovative technology allows for massively parallel sequencing of DNA. It sequences millions of fragments simultaneously, making it possible to conduct whole-genome analyses at an unprecedented scale and speed. NGS has become the standard approach for genome mapping and variant discovery due to its efficiency and cost-effectiveness.
- Third-Generation Sequencing: This emerging technology focuses on sequencing long stretches of DNA in real-time. Technologies like PacBio and Oxford Nanopore allow for reading entire molecules instead of fragments. This capability enhances the accuracy of structural variant detection and provides a more comprehensive view of genomic architecture.
"The advancement of sequencing technologies not only expands our understanding of genetics but also opens new avenues for research and personalized medicine."
"The advancement of sequencing technologies not only expands our understanding of genetics but also opens new avenues for research and personalized medicine."
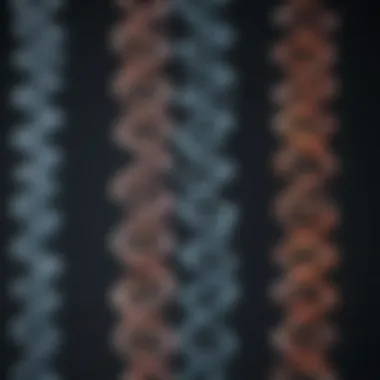
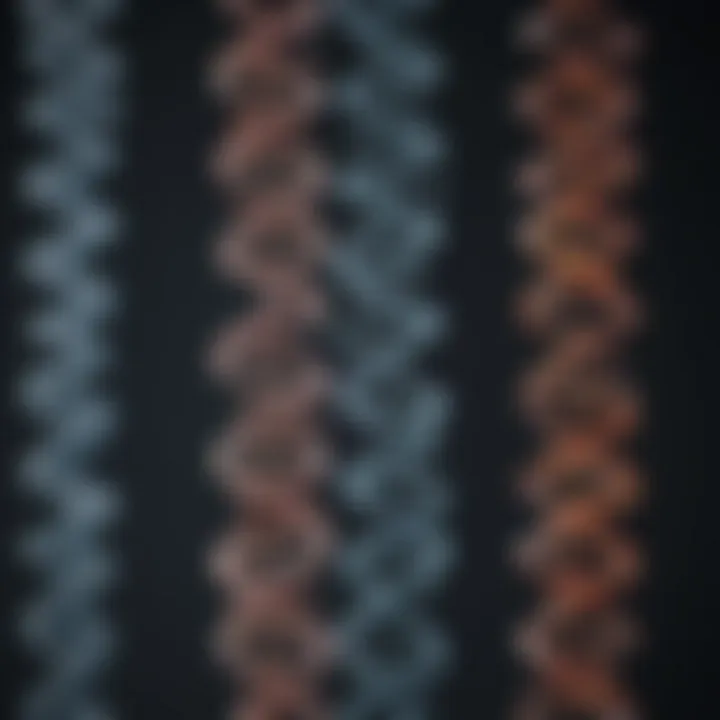
In summary, the fundamental principles of DNA sequencing revolve around the unique structure and vital function of DNA. The evolution of sequencing technologies continues to influence how we understand genetic information, thereby shaping the future of biological research and applications across various fields.
Techniques in Full DNA Sequencing
The methods used in full DNA sequencing are critical for understanding genetic material. These techniques not only establish the fundamentals of sequencing but also influence the quality and speed of genetic analysis. The distinct approaches to sequencing each have unique merits and limitations, which can guide researchers in choosing the most suitable method for their projects.
Sanger Sequencing
Sanger sequencing, often labeled as the first major breakthrough in DNA sequencing, has played a significant role in genetic research since its inception by Frederick Sanger in the 1970s. This method works by selectively incorporating chain-terminating dideoxynucleotides during DNA synthesis.
The strength of Sanger sequencing lies in its high accuracy and reliability, making it the gold standard for sequencing smaller fragments of DNA. It can effectively determine sequences of up to approximately 1,000 base pairs, which is sufficient for targeted applications like sequencing genes of interest.
However, there are notable downsides. Sanger sequencing is time-consuming and expensive when applied to whole genomes. For researchers who require comprehensive data from larger samples, this method can be limiting. Its primary use today is mostly in validation of results obtained from more advanced sequencing techniques.
Next-Generation Sequencing (NGS)
Next-Generation Sequencing represents a revolutionary shift in the field of genomics. Unlike Sanger sequencing, which processes one DNA strand at a time, NGS enables massively parallel sequencing. This means millions of fragments can be sequenced simultaneously, dramatically increasing throughput and reducing costs per base.
The versatility of NGS is noteworthy. It supports various applications including whole-genome sequencing, transcriptome analysis, and targeted resequencing, among others. This adaptability has made NGS a favored choice among researchers across multiple disciplines, including personalized medicine, cancer genomics, and microbial ecology.
One challenge with NGS is the complexity of data analysis. Due to the vast amount of data produced, processing and interpreting results require significant bioinformatics resources. Moreover, ensuring data quality amidst noise can be problematic, where distinguishing true variants from artifacts is essential.
Third-Generation Sequencing
Third-Generation Sequencing is an emerging technology that enhances our ability to understand complex genomes. Unlike both Sanger and NGS, which rely on amplification, third-generation techniques, such as nanopore sequencing or single-molecule real-time sequencing, read DNA directly. This method allows for the detection of longer sequences, often exceeding 10,000 base pairs.
The primary advantage of third-generation sequencing is its capacity to provide comprehensive insights into genomic structures, including repetitive regions and structural variants that are difficult to analyze with previous technologies. This flexibility makes it particularly useful in diverse applications like metagenomics, population genomics, and evolutionary studies.
However, challenges include lower accuracy compared to Sanger sequencing and higher error rates that can complicate data interpretation. As this technology matures, improvements are anticipated, likely broadening its utility in genetics research.
"The evolution of sequencing technologies like Sanger, NGS, and Third-Generation illustrate the dynamic nature of genomics, each presenting unique challenges and advantages that guide research directions."
"The evolution of sequencing technologies like Sanger, NGS, and Third-Generation illustrate the dynamic nature of genomics, each presenting unique challenges and advantages that guide research directions."
In summary, the techniques of full DNA sequencing reflect a continuum of innovation. Each method offers specific benefits suited to different research needs. Understanding these techniques enhances the application of full DNA sequencing across biological and medical sciences.
Data Analysis in Full DNA Sequencing
Data analysis serves as a crucial component in full DNA sequencing. The vast quantities of data generated during sequencing require meticulous analysis to yield meaningful insights. Analyzing DNA data helps identify genetic variants that could have significant implications in various fields. It also underpins research in areas such as personalized medicine, genetic disorders, and evolutionary biology.
Effective data analysis not only enhances our understanding of genetic structures but also improves decision-making processes in clinical and research settings. Here, we will delve into the technical aspects of bioinformatics, variant calling, and visualization tools that make up the data analysis landscape.
Bioinformatics and Data Processing
Bioinformatics integrates computer science, statistics, and biology to process and analyze biological data. In the context of full DNA sequencing, bioinformatics is essential for managing the enormous sets of data generated from sequencing technologies.
The processing of DNA sequences begins with quality control measures. These measures ensure that raw data is free from errors and inaccuracies. After quality assurance, the next phase involves alignment. This process refers to arranging sequences in a manner that makes it possible to compare them effectively. Algorithms like BWA and Bowtie are frequently utilized for this purpose.
The importance of bioinformatics is underscored in the analysis pipeline. Advanced software tools are necessary to facilitate each stage of data processing:
- Quality assessment software (e.g., FastQC)
- Alignment algorithms (BWA, Bowtie)
- Variant detection and annotation tools (GATK, ANNOVAR)
This systematic approach ensures that researchers can extract pertinent information accurately and efficiently. It sets the groundwork for further analyses that reveal biological implications.
Variant Calling and Analysis
Variant calling is a pivotal step in understanding genetic differences within and between populations. This process involves identifying variations from the reference genome. Variants can include single nucleotide polymorphisms (SNPs), insertions, deletions, and structural variants.
Detecting these variants has profound significance. For instance, SNPs can indicate susceptibility to diseases or response to drugs, which assists in tailoring treatment regimens in personalized medicine.
Several tools and approaches exist for variant calling. Each has its strengths and specific use cases:
- GATK (Genome Analysis Toolkit) is widely used for SNP and variant detection due to its comprehensive algorithms.
- FreeBayes and samtools are alternatives that perform well in different contexts.
Validating the quality and reliability of variant calls is necessary, as erroneous calls can lead to misleading conclusions. Additionally, understanding the distribution of variants aids in recognizing evolutionary relationships and population genetics.
Visualization Tools
Visualization plays an essential role in the interpretation of complex genetic data. Through graphical representations, researchers can discern patterns and insights that are not immediately obvious in raw data. Visualization tools allow for the effective communication of findings.
Various tools enable effective data visualization in DNA sequencing:
- IGV (Integrative Genomics Viewer) offers an interactive visualization of genomic data. It allows users to explore the alignments and variant calls efficiently.
- Circos provides circular visualizations that depict relationships among genes, variants, and other genomic features.
- R and Python libraries such as ggplot2 and Matplotlib may also be employed for custom visualizations.
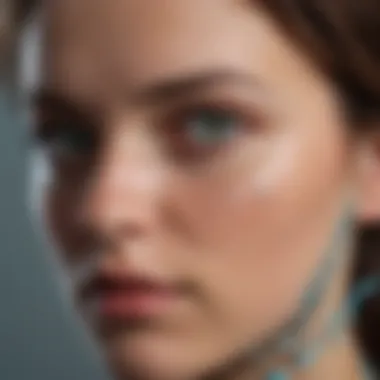
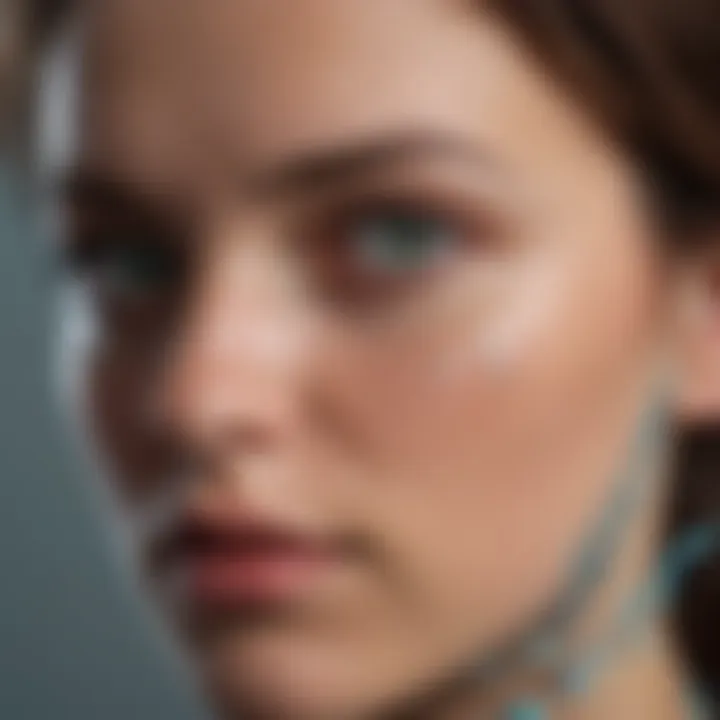
These tools help present findings to diverse audiences, from researchers to policy-makers. They are instrumental in developing a narrative around the data, enriching discussions regarding genetic research and its implications.
"Data analysis transforms raw DNA sequences into actionable insights, bridging the gap between genetics and practical applications in medicine and biology."
"Data analysis transforms raw DNA sequences into actionable insights, bridging the gap between genetics and practical applications in medicine and biology."
Applications of Full DNA Sequencing
Full DNA sequencing is not merely a scientific novelty. It holds significant importance across diverse fields such as medicine, agriculture, and anthropology. Understanding its applications allows researchers and professionals to leverage its potential fully and enhance outcomes in various sectors. This section provides insights into how full DNA sequencing can lead to groundbreaking advancements and transformations in these areas.
Clinical Implications
The clinical applications of full DNA sequencing are profound. One of the main benefits is its role in personalized medicine. By analyzing an individual's genetic makeup, healthcare professionals can tailor treatments to meet the specific needs of each patient. This ensures higher efficacy and minimizes adverse effects. For instance, oncologists can utilize full DNA sequencing to identify mutations that drive cancer progression, helping in the selection of appropriate therapies.
Another crucial aspect is the early detection of genetic disorders. Whole-genome sequencing can uncover predispositions to hereditary diseases, allowing for proactive measures and targeted interventions.
The following points summarize the clinical implications:
- Personalized treatments: Tailored therapies based on genetic information.
- Early detection: Uncovering genetic predispositions for timely interventions.
- Pharmacogenomics: Understanding how genes affect drug response.
"Full DNA sequencing is reshaping the landscape of health care by promoting precision medicine tailored to individual genetic profiles."
"Full DNA sequencing is reshaping the landscape of health care by promoting precision medicine tailored to individual genetic profiles."
Agricultural Biotechnology
In agriculture, full DNA sequencing plays a pivotal role in enhancing crop yields and resilience. By understanding the genetic traits of plants, researchers can develop improved varieties that resist pests, tolerate harsh climates, and yield better produce. This application is particularly vital as the world faces growing food security challenges.
Moreover, full DNA sequencing allows for the identification of desirable traits in crop species, facilitating marker-assisted selection. This is important for accelerating the breeding process without the need for extensive field trials, which can be time-consuming and costly.
Key benefits in agricultural biotechnology include:
- Improved crop varieties: Developing crops that can withstand environmental stresses.
- Sustainable practices: Enhancing food production with minimal environmental impact.
- Biodiversity conservation: Assisting in the preservation of genetic resources.
Evolutionary Studies
Full DNA sequencing offers unparalleled insights into evolutionary biology. By analyzing the genomes of various organisms, scientists can trace lineage and understand the genetic basis of evolution. This contributes to our comprehension of how species adapt to changing environments and the mechanisms behind speciation.
Additionally, DNA sequencing aids in paleo-genomics, allowing researchers to extract genetic information from ancient remains. This helps reconstruct past ecosystems and understand how past climate changes affected biodiversity.
Essential contributions to evolutionary studies include:
- Phylogenetics: Understanding evolutionary relationships through genetic analysis.
- Adaptation mechanisms: Investigating how species adjust to environmental pressures.
- Conservation genetics: Informing strategies to protect endangered species based on genetic diversity.
Overall, the applications of full DNA sequencing not only enhance knowledge in their respective fields but also promote advances that have far-reaching implications for humanity and the natural world.
Ethical Considerations in DNA Sequencing
The rise of full DNA sequencing technology has brought profound advancements in genetic research and applications. However, with these advancements come significant ethical considerations that must be addressed. These considerations not only impact the individuals undergoing sequencing but also affect society, policy, and future research directions. It is crucial to recognize these ethical dimensions in the context of the broader implications of DNA sequencing.
Privacy and Data Security
DNA data holds sensitive information about individuals' genetic predispositions and ancestral backgrounds. Thus, safeguarding this information is paramount. Protecting privacy involves not only preventing unauthorized access but also ensuring that individuals are informed about how their genetic data will be used.
- Data Encryption: Implement robust encryption methods to protect stored genetic information. This helps prevent data breaches and unauthorized access to sensitive genetic material.
- Access Controls: Only allow certain individuals or teams to access genetic data. Establishing strong protocols ensures that only those who need the data for research or clinical purposes can view it.
- Data Anonymization: Anonymizing data can further secure individuals' identities while still allowing for meaningful analysis of trends and correlations in genetic data.
By prioritizing privacy and data security, researchers can foster trust among participants and promote wider acceptance of DNA sequencing technologies.
Informed Consent
Informed consent is pivotal in any medical or research procedure, and DNA sequencing is no exception. Individuals must be fully comprehended of what participation entails, including any potential risks and benefits. The importance of informed consent in DNA sequencing can be categorized as follows:
- Understanding Risks: Participants should know about the possible outcomes of being sequenced, such as incidental findings that may indicate health risks.
- Autonomy: Individuals should retain control over their genetic information. They should have the right to decide whether or not to participate in sequencing and how their data is utilized.
- Ongoing Communication: The consent process should not be a one-time event. Researchers need to ensure ongoing communication with participants as new findings and ethical standards evolve.
Ensuring informed consent builds a framework of trust and ethical integrity within the research community.
Implications for Genetic Discrimination
With the advancements in genomic sequencing, there exists a risk of genetic discrimination based on an individual’s genetic information. Genetic discrimination can manifest in various areas, including employment and insurance. The implications include:
- Employment Decisions: Employers may misuse genetic information to make hiring or promotion decisions, potentially disadvantaging individuals with unfavorable genetic predispositions.
- Insurance Coverage: Insurers might refuse coverage or charge higher premiums based on one's genetic risk factors, leading to inequities in healthcare access.
- Social Stigma: Those with specific genetic markers may face prejudice and stigmatization, impacting their mental health and social interactions.
Legislative measures, like the Genetic Information Nondiscrimination Act (GINA) in the United States, aim to mitigate these risks. However, as DNA sequencing becomes more widespread, ongoing vigilance is necessary to protect individuals against potential abuses of their genetic data.
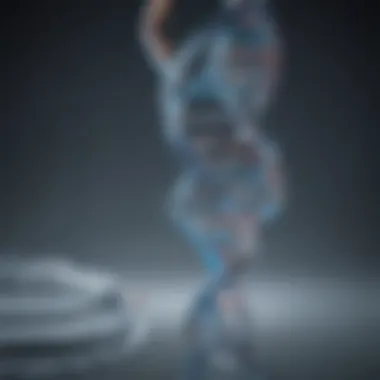
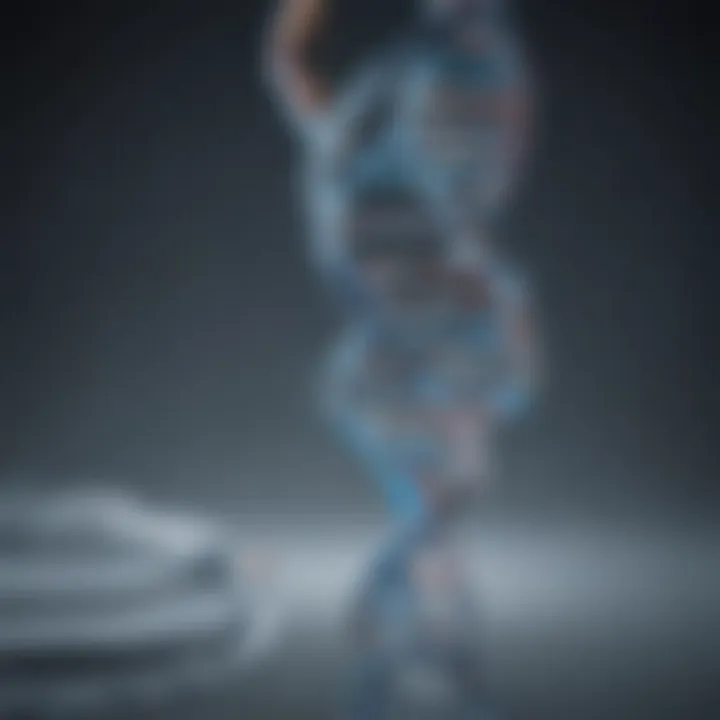
Ethical considerations in DNA sequencing are not just legal obligations; they are essential for nurturing public trust and ensuring that the benefits of genetic research are accessible to all without discrimination.
Ethical considerations in DNA sequencing are not just legal obligations; they are essential for nurturing public trust and ensuring that the benefits of genetic research are accessible to all without discrimination.
Challenges Facing Full DNA Sequencing
Full DNA sequencing has opened exciting avenues in genetics, yet it comes with particular challenges interconnected to its techniques and application. These challenges significantly affect how researchers and practitioners utilize sequencing data. Understanding these obstacles is crucial for guiding future developments in technology and methodology.
Technical Limitations
One major challenge in full DNA sequencing relates to technical limitations inherent in various sequencing technologies. Each method, whether it is Sanger sequencing or Next-Generation Sequencing (NGS), has its own strengths and barriers. One notable limitation is read length. For instance, NGS provides shorter reads compared to Sanger sequencing, which can complicate assembly of complex genomes. This half creates difficulties in accurately identifying repetitive sequences and structural variants. Additionally, the sensitivity of these techniques can vary, affecting the detection of low-frequency variants, particularly in heterogeneous samples, like cancer genomes.
Improving accuracy also presents itself as a significant hurdle. Errors in base calling during sequencing can lead to misinterpretation of genetic data. This challenge often requires additional steps in data analysis, including the use of sophisticated algorithms to filter noise from useful information. The integration of error-correction platforms is a potential solution, yet it adds layers of complexity to the data analysis process.
Data Overload
The issue of data overload is a pressing concern in full DNA sequencing. As sequencing technologies advance, the volume of data generated increases exponentially. A single sequencing project can now yield terabytes of data. This sheer amount of information can overwhelm both computational infrastructure and researchers. It necessitates the need for significant storage and efficient databases for data management. Furthermore, analyzing such vast datasets to extract meaningful insights poses another challenge.
Researchers often struggle with data interpretation, making it difficult to discern between clinically relevant variations and benign polymorphisms. Large datasets also require robust bioinformatics tools and skills, which may not be readily available in all research settings. Thus, developing streamlined data processes and educative tools becomes essential in managing this deluge of genetic data.
Interdisciplinary Collaboration
Lastly, effective interdisciplinary collaboration is crucial in addressing the challenges of full DNA sequencing. The complexity of genetic data requires cooperative efforts among geneticists, bioinformaticians, data scientists, and healthcare professionals. Successful collaboration can lead to innovations and strategies that enhance sequencing capabilities and data utilization.
Collaboration across disciplines fosters a richer understanding of intricate genetic interactions, ultimately enriching interpretation and application of sequencing data.
Collaboration across disciplines fosters a richer understanding of intricate genetic interactions, ultimately enriching interpretation and application of sequencing data.
However, communication gaps among these experts can result in misunderstandings and mismanagement of data. Creating a common language and framework for collaboration remains essential. Professional development programs and workshops that encourage interdepartmental exchanges can help overcome these obstacles, ensuring that the full potential of DNA sequencing is realized in various fields, from medicine to agriculture.
Future Directions of Full DNA Sequencing
Full DNA sequencing is rapidly evolving, proving its worth across various disciplines such as healthcare, agriculture, and evolutionary biology. Understanding its future directions is vital for realizing its potential. The advancements in sequencing are not merely about increased speed or reduced costs; they encompass innovations that redefine the understanding of genetics itself. Progress in areas such as technology improvements and artificial intelligence integration herald a new era in genomics.
Innovations in Sequencing Technologies
Future innovations in sequencing technologies promise to enhance accuracy, decrease time, and lower costs. One important focus is on developing faster sequencing methods. Companies like Illumina are working on techniques that achieve results in real-time, providing quicker insights into genetic information. Furthermore, improvements in single-molecule sequencing can offer higher quality data without the need for amplification, ensuring that the sample integrity remains intact.
Another area of focus is the miniaturization of sequencing devices. Portable sequencers, such as the ones from Oxford Nanopore Technologies, allow for DNA sequencing to be conducted in remote locations, which is particularly beneficial for fieldwork in environmental and agricultural applications.
In addition, emerging technologies such as quantum computing could revolutionize the way we process sequencing data, enabling analysis at an unprecedented scale and speed. Thus, the future of sequencing technology is not just about efficiency but also about accessibility and expanded capabilities.
"Advancements in sequencing technologies are essential for translating genetic information into actionable insights in healthcare and beyond."
"Advancements in sequencing technologies are essential for translating genetic information into actionable insights in healthcare and beyond."
Integration with Artificial Intelligence
The integration of artificial intelligence (AI) with DNA sequencing is another promising avenue. AI algorithms can analyze vast amounts of genetic data quickly and accurately. They are essential for identifying patterns and variants that would take significant time and effort for human analysts to detect.
Machine learning models can improve variant calling accuracy, enabling personalized medicine strategies based on an individual's distinct genetic makeup. For instance, AI systems can predict genetic disorders by analyzing a patient's complete genomic sequence in comparison to large annotated datasets of known conditions.
Moreover, AI can enhance bioinformatics, the field responsible for interpreting and managing the data generated by sequencing technologies. Advanced algorithms can streamline workflows, reduce human error, and make sense of complex genomic information. As the size and complexity of genetic data continue to grow, the collaboration between sequencing technologies and AI becomes increasingly critical.
In summary, understanding the future of full DNA sequencing involves recognizing the importance of technological advancements and the role of AI in driving further discoveries in genetics. Together, they lay the groundwork for breakthroughs that can impact various fields, ultimately expanding the horizons of biological research and medical applications.
End
The conclusion serves as a vital component in this exploration of full DNA sequencing. It encapsulates the major themes of the article and emphasizes the continuing relevance and potential of this technology in various fields.
Recap of Key Insights
Full DNA sequencing allows for thorough examination of genetic materials, significantly impacting personalized medicine and biotechnology. Here’s a brief summary of essential points:
- Understanding Genetic Information: It enables researchers to uncover the complexities of genetic codes, which can lead to better disease prediction and treatment plans.
- Technological Advances: Innovations in sequencing methods, such as Next-Generation Sequencing (NGS), have drastically reduced time and costs related to sequencing projects.
- Ethical Considerations: Key ethical concerns, including privacy and consent, must be taken into account as the capability to sequence DNA becomes widespread.
These insights not only highlight the current capabilities of full DNA sequencing, but also the challenges that accompany its implementation and use in society.
The Ongoing Impact of Full DNA Sequencing
The impact of full DNA sequencing continues to evolve as the field experiences rapid advances. It has transformed research and healthcare in several profound ways:
- Personalized Medicine: With full DNA sequencing, tailored therapeutic strategies based on individual genetic profiles become increasingly reliable.
- Genomic Research: It plays a crucial role in understanding hereditary conditions and developing targeted therapies.
- Agricultural Improvements: Sequencing technologies aid in the development of genetically modified organisms that can withstand environmental stressors.
"The integration of full DNA sequencing into routine medical diagnostics will reshape our approach to health management and disease prevention."
"The integration of full DNA sequencing into routine medical diagnostics will reshape our approach to health management and disease prevention."
In summary, full DNA sequencing is not merely a scientific endeavor; it is a cornerstone for future innovations in medicine and biotechnology. Its effects resonate beyond the laboratory, influencing public health policies and ethical standards in genetics. As researchers and technologists continue to push boundaries, the implications of this technology will surely expand, driving new discoveries and advancements in multiple disciplines.