Understanding Gene Mutants: Mechanisms and Implications
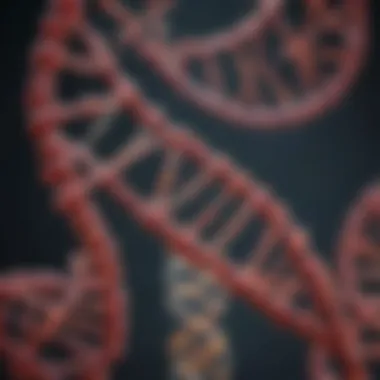
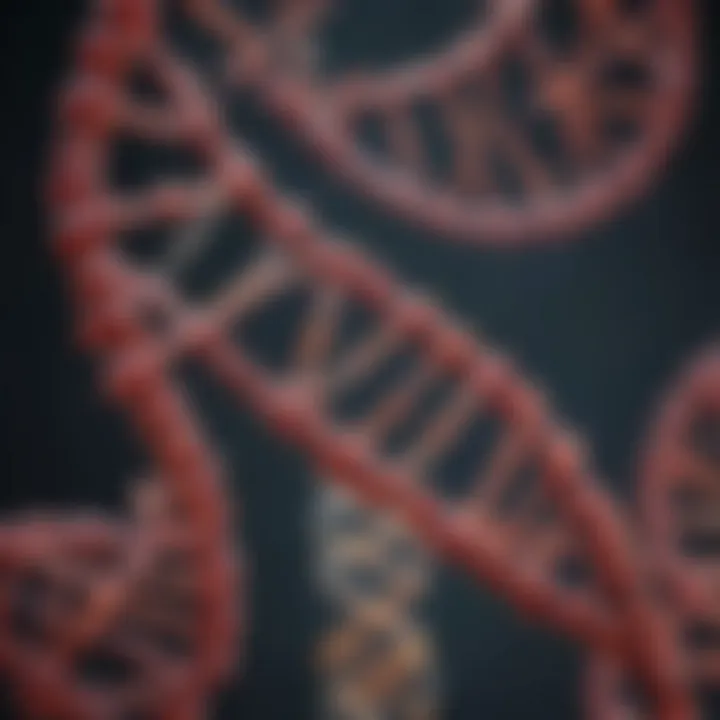
Intro
Gene mutants represent a captivating area of study in genetics, vital for understanding the diversity of life. Mutations can lead to significant changes in an organism's traits. These changes can be harmless, beneficial, or harmful, influencing both individual organisms and broader ecological systems. Recognizing the mechanisms behind gene mutations provides insight into evolution, disease, and biotechnological advancements.
Key Concepts
Definition of the Main Idea
Gene mutants are alterations in the genetic code. These changes can arise from various factors, including errors during DNA replication, environmental influences, and more. The main types of mutations include point mutations, insertions, deletions, and duplications. These mutations can affect how genes function, leading to a range of phenotypic outcomes.
Overview of Scientific Principles
At the core of understanding gene mutants are principles of molecular biology. The central dogma of molecular biology explains how genes are expressed. DNA is transcribed into RNA, which is then translated into proteins. Mutations can disrupt this flow, leading to altered or non-functional proteins. Environmental factors, such as radiation or chemicals, can exacerbate mutation rates, further complicating genetic health.
"Understanding gene mutations is essential to grasp how species adapt and evolve."
"Understanding gene mutations is essential to grasp how species adapt and evolve."
Current Research Trends
Recent Studies and Findings
The field of genetic research has seen significant advancements. New technologies, such as CRISPR-Cas9, allow for precise editing of genes. Recent studies show how specific mutations can contribute to diseases like cancer. Understanding these pathways opens doors to potential therapies. Researchers are using animal models to study the impact of these mutations in more detail, providing direct correlations between genetics and disease outcomes.
Significant Breakthroughs in the Field
Breakthrough studies have reshaped our comprehension of gene mutants. Notable research demonstrates how gene therapy can correct specific mutations. This approach has therapeutic implications for inherited disorders. Additionally, exploring the role of epigenetics has unveiled a layer of complexity in gene expression. Factors like methylation affect how genes are read, adding further depth to the understanding of gene mutants.
Prolusion to Gene Mutants
Gene mutants play a critical role in understanding genetics, evolution, and various diseases. This section introduces the concept of gene mutants, providing insight into their relevance in modern science. It is essential to grasp the basics of gene mutations, as they are foundational to fields such as molecular biology and genetic engineering.
Gene mutations can serve as a source of variation within populations. This variation is crucial for the process of natural selection, leading to the adaptation of species over time. The study of gene mutants helps researchers comprehend how organisms evolve and adapt to changing environments.
Moreover, gene mutations have profound implications in medicine. They can lead to genetic disorders or predispose individuals to certain diseases, including cancer. Through a deeper understanding of gene mutations, scientists can work toward developing targeted therapies and innovative treatments.
In addition to their academic importance, gene mutants have real-world applications in biotechnology. They are utilized in the creation of genetically modified organisms (GMOs) and biopharmaceuticals. These developments highlight the direct impact of gene mutants in enhancing agricultural practices and advancing healthcare.
"Understanding gene mutants is not just about studying changes in genetic material; it is about comprehending the underlying mechanisms that drive evolution and disease."
"Understanding gene mutants is not just about studying changes in genetic material; it is about comprehending the underlying mechanisms that drive evolution and disease."
The implications of gene mutations extend beyond theoretical knowledge. As society grapples with ethical questions around genetic modification, a firm grasp of gene mutants is necessary. This section sets the stage for a more detailed examination of the definition, mechanisms, and applications of gene mutations in the subsequent sections.
In summary, the introduction of gene mutants is a vital topic, integral to the broader discussions surrounding genetics. It opens a plethora of inquiries ranging from biological processes to ethical considerations. The relevance of this knowledge spans education, research, and practical applications in biotechnology and medicine.
Definition of Gene Mutants
Understanding gene mutants is essential for grasping the complexities of genetics and their impact on evolution, health, and biotechnology. Gene mutants arise from changes in the DNA sequence, leading to variations that can influence how organisms develop and function. These variations play a crucial role in the broader scope of biological research and medical applications. By defining gene mutants, we can facilitate conversations about mutation effects on species, health-related issues, and the direction of future research.
Understanding Mutation
Mutation is a natural process that occurs in all living organisms. It serves as a source of genetic diversity, which is vital for adaptation and survival. Mutations can happen due to various reasons, such as mistakes in DNA replication or influences from environmental factors. Concrete understanding of mutations lays the groundwork for examining their various forms and consequences, highlighting their importance in both genetics and biologic traits.
Types of Gene Mutations
Gene mutations can be categorized in different ways, each category presenting unique characteristics and implications.
Substitutions
Substitutions involve the replacement of one nucleotide with another in the DNA sequence. This type of mutation is particularly significant because it can alter a single amino acid in a protein, potentially impacting that protein's function. A critical aspect of substitutions is that they can lead to silent mutations, which do not change the amino acid sequence, or nonsynonymous mutations that do. Such variations make substitutions a popular topic in genetic studies, as they can explain a variety of phenotypic outcomes. However, while substitutions may be beneficial in certain contexts, they may also lead to harmful genetic diseases if they disrupt essential protein functions.
Insertions
Insertions occur when extra nucleotides are added into a DNA sequence. This type of mutation can result in frameshift changes, altering the reading frame and significantly affecting the resulting protein. The key feature of insertions is that they can introduce premature stop codons, leading to truncated proteins. For this article, inserting information about insertions is beneficial because it helps in illustrating how genetic variability can arise and the potential risks associated with it. Insertions can be beneficial by creating new functions in proteins, but they may also lead to genetic disorders if they disrupt normal gene function.
Deletions
Deletions involve the removal of nucleotides from a DNA sequence. Like insertions, deletions can cause frameshift mutations, which can drastically change the subsequent amino acid sequence. The key consideration with deletions is their potential to disrupt protein production. This characteristic underscores their importance in the study of genetic mutations and can lead to conditions such as cystic fibrosis or muscular dystrophy. Deletions serve as a powerful reminder of how loss of genetic information can have significant implications for health and function, making them an essential part of any discourse on gene mutants.
Mechanisms of Mutation
Understanding the mechanisms of mutation is crucial for grasping how gene mutants function and their broader implications in biology. These mechanisms highlight the underlying processes by which genetic changes occur. Knowing these mechanisms can clarify how mutations contribute to traits and adaptations, impacting everything from evolutionary biology to disease manifestation. Moreover, recognizing that mutations can arise from both intrinsic and extrinsic factors allows scientists to better predict genetic variations. This section delves into two primary pathways leading to mutations: replicative errors in DNA replication and various environmental factors influencing mutation rates.
Replicative Errors
Replicative errors are mistakes made during the DNA duplication process. These errors can occur due to a variety of reasons, such as faulty DNA polymerase action or issues with the replication machinery. If not corrected, these errors become permanent mutations in the genome.
One key characteristic of replicative errors is their relative prevalence in large populations. As cells divide frequently, the probability of errors increases, especially in regions of the genome that are more prone to instability. Importantly, not all replicative errors lead to detrimental effects; some may occur in non-coding regions and have no observable consequences. However, when these errors do affect coding regions, they can lead to significant phenotypic changes.
In this discussion, we see how these errors, although sometimes minor, cumulatively contribute to genetic diversity within a population. They are central to understanding how organisms evolve over generations. Recognizing the inherent nature of these errors provides important insight into both normal biology and the mechanisms behind various genetic disorders.
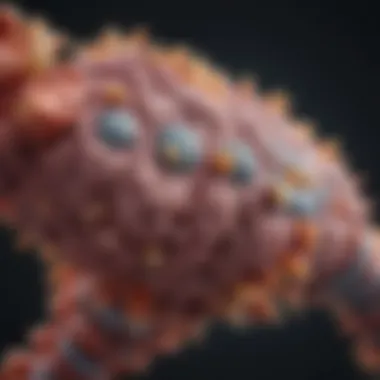
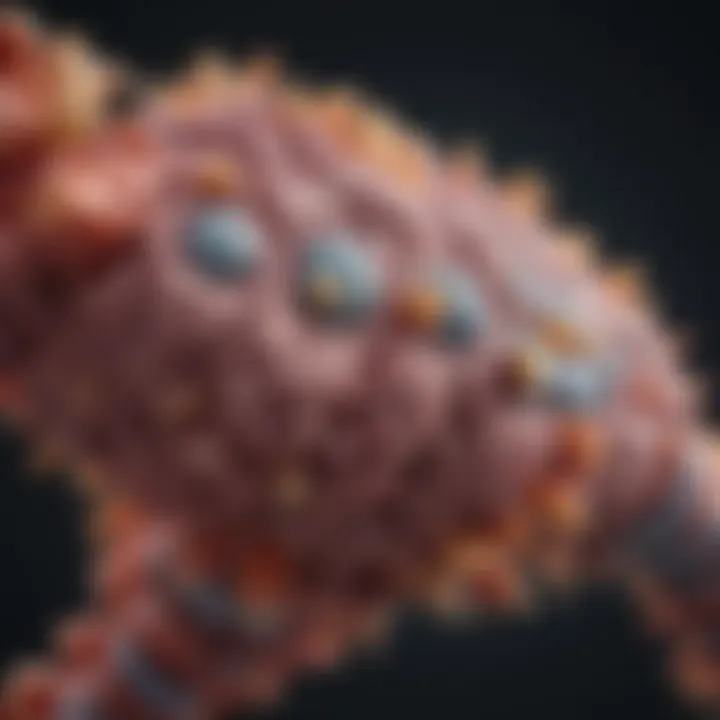
Environmental Factors Inducing Mutations
Environmental factors are external agents that can induce mutations, leading to changes in the genetic material. These include radiation, chemical exposure, and biological agents. Each factor has unique impacts and implications within genetic research.
Radiation
Radiation serves as a substantial agent of mutation. It can cause breaks in DNA strands and lead to various genetic alterations. The key characteristic of radiation is its ability to penetrate cells and disrupt molecular structures. This property makes it a powerful choice for studying gene mutations in laboratories.
Moreover, radiation can be beneficial in certain medical treatments, such as cancer therapy. However, its unique feature also comes with disadvantages, such as unintended mutations that could contribute to diseases like cancer. Understanding radiation’s role is essential in both therapeutic settings and in evaluating environmental safety.
Chemical Exposure
Exposure to chemicals can also provoke mutations. Certain substances can directly interact with DNA, leading to alterations in base pairs or the addition of unusual groups. The key characteristic of chemical exposure lies in its variety—many different types of chemicals can have mutagenic effects, from industrial pollutants to naturally occurring toxins.
One notable aspect of chemical exposure is that the effects can be dose-dependent. Low-level exposures may result in few or no mutations, while higher concentrations can cause significant genetic damage. This nuance highlights both the advantages and risks associated with chemical exposure in biological systems, emphasizing the need for careful assessment in genetic research.
Biological Agents
Biological agents, such as viruses and bacteria, can also lead to mutations. These agents often integrate their own genetic material into the host genome, which can alter gene expression and function. One key characteristic of biological agents is their specificity; different organisms can introduce mutations via various mechanisms, such as transduction or transformation.
This unique feature allows researchers to explore mutation pathways that are not present with chemical agents. Understanding biological agents and their implications is beneficial for studying genetic diseases and viral infections, as they can reveal insights into how mutations contribute to pathology.
In summary, the mechanisms of mutation provide a framework for understanding how genetic variations arise. Replicative errors and environmental factors each play distinct roles in mutation processes. They are critical for both evolutionary biology and medical research, affecting approaches to treatment and prevention of genetic diseases.
Expression and Phenotypic Effects of Mutations
The study of expression and phenotypic effects of mutations is key in understanding how genetic changes manifest in living organisms. These mutations can affect not only the structure and function of proteins but also the overall phenotype of an organism. This section will explore the distinctions between silent and expressive mutations, along with the broader implications on protein functions, particularly the concepts of loss of function and gain of function mutations.
Silent vs. Expressive Mutations
Silent mutations are changes in the DNA sequence that do not result in a change in the amino acid sequence of a protein. These changes occur due to the redundancy in the genetic code, where multiple codons can code for the same amino acid. Although they do not have an immediate observable effect on protein function, silent mutations can still impact gene expression levels or splicing efficiency. For example, certain silent mutations may influence how well a mRNA is translated into a protein, thus subtly affecting an organism's phenotypic traits.
On the other hand, expressive mutations lead to visible changes in the phenotype. These mutations can alter the function of proteins, which can affect an organism's survival and reproductive success. Understanding the differences between silent and expressive mutations is essential for applications in evolutionary biology and medicine, as it highlights the spectrum of potential impacts mutations can have.
Impact on Protein Function
The implications of mutations on protein function are profound. These changes can be categorized primarily into two types: loss of function and gain of function.
Loss of Function
Loss of function mutations result in the reduced activity of a protein. This can happen through various mechanisms such as premature stop codons that truncate the protein or missense mutations that change amino acids to non-functional ones. A key characteristic of loss of function mutations is their ability to disrupt essential cellular processes.
For example, in context of genetic diseases, loss of function mutations in the CFTR gene lead to cystic fibrosis. The mutations prevent normal CFTR protein function, resulting in severe respiratory and digestive issues. This type of mutation is a significant choice for our article because it emphasizes how changes at the genetic level can lead to serious health implications.
While loss of function mutations have clear disadvantages, they also play important roles in research. Studying these mutations can provide insights into disease mechanisms and can guide the development of therapies.
Gain of Function
In contrast, gain of function mutations result in an increased or new activity of a protein. These mutations may cause proteins to adopt new functions or increase their activity levels beyond normal capacity. A prominent example of gain of function mutations is found in oncogenes, where mutations lead to enhanced cell proliferation, contributing to cancer development.
A key characteristic of gain of function mutations is their potential to confer advantageous traits under specific environmental conditions. This makes the study of gain of function mutations particularly beneficial for understanding evolutionary processes.
However, gain of function mutations can also present challenges. They might lead to uncontrolled cellular growth or resistance to therapies. This dual nature makes them a compelling focus in both evolutionary biology and medical research.
Understanding the impact of mutations on protein function not only illuminates the biological processes at play but also underscores the significance of genetic research in medicine and biotechnology.
Understanding the impact of mutations on protein function not only illuminates the biological processes at play but also underscores the significance of genetic research in medicine and biotechnology.
Gene Mutants in Evolutionary Biology
Gene mutants play a critical role in the study of evolutionary biology. They provide insights into how genetic variation contributes to the processes that lead to adaptation and the emergence of new species. Understanding gene mutants helps researchers comprehend how organisms respond to environmental challenges over generations. The mechanisms behind these changes inform critical discussions on biodiversity, species survival, and the intricate dance of life on Earth.
Role in Natural Selection
Natural selection relies heavily on gene mutants. These mutations often provide the raw material for evolutionary change. When a mutation occurs, it may alter an organism's phenotype, potentially providing an advantage or disadvantage in its habitat. The following points illustrate how gene mutants are tied to natural selection:
- Variation: Mutations create genetic diversity within a population. This variation is vital for natural selection as it allows some organisms to thrive while others may not.
- Selection Pressure: Environmental factors impose selection pressures. Organisms with favorable mutations may have better reproductive success, leading to an increased frequency of these traits over generations.
- Adaptability: Gene mutants enable species to adapt to changing environments. For instance, a mutation that enhances drought resistance in plants may be favored in arid conditions, leading to greater survival.
Learning how gene mutants interact within this framework is essential for understanding evolutionary dynamics.
Adaptive Evolution Through Mutations
Mutations are a driving force behind adaptive evolution. These genetic changes facilitate the emergence of traits that enable organisms to occupy new ecological niches.
- Survival of the Fittest: When mutations confer advantages, those traits spread throughout populations. For example, antibiotic resistance in bacteria is a direct result of gene mutations, allowing them to survive in the presence of these drugs.
- Rapid Adaptation: Some populations may experience rapid changes due to immediate environmental threats. For instance, the peppered moth demonstrated swift adaptive responses to increased pollution during the Industrial Revolution, highlighting the power of gene mutants in evolutionary context.
- Speciation: Over extended periods, accumulated mutations can lead to significant differences between populations, ultimately resulting in speciation. This is observed in isolated environments where specific gene mutants may thrive under localized conditions.
"Gene mutations are essential to the process of evolution, unlocking the potential for diversity and the ability to adapt to changing environments."
"Gene mutations are essential to the process of evolution, unlocking the potential for diversity and the ability to adapt to changing environments."
By studying gene mutants, researchers not only unravel the history of life on Earth but also gain insights into possible future adaptations of living organisms.
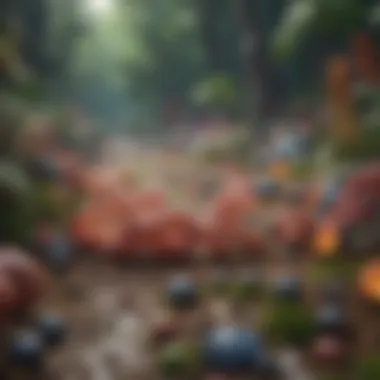
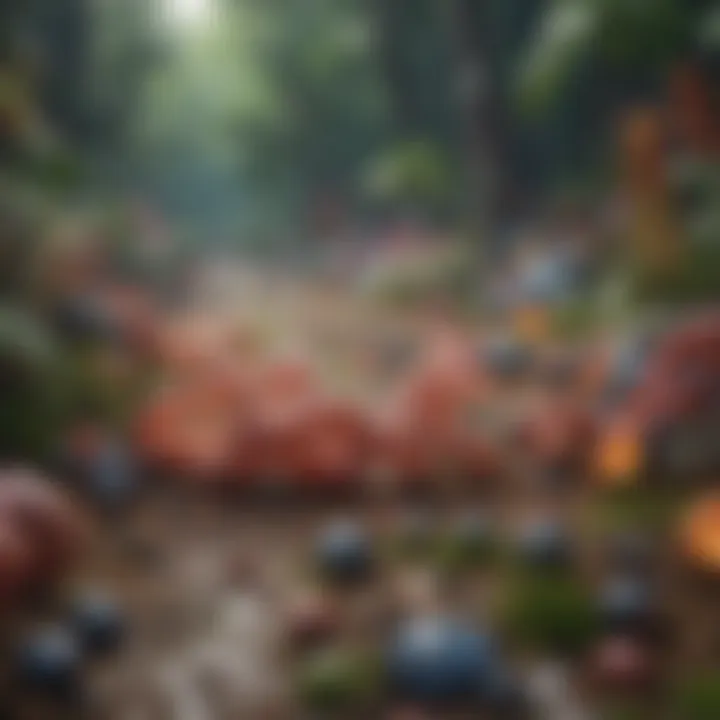
Pathological Implications of Gene Mutations
The significance of gene mutations extends deeply into the realm of pathology. Understanding these mutations is crucial for unraveling the complexities of human diseases and developing effective treatment strategies. Pathological implications of gene mutations encompass a variety of health conditions ranging from genetic disorders to complex diseases and cancer. Addressing these issues sheds light on the genetic basis of diseases, which can lead to preventive measures, personalized medicine, and targeted therapies.
Genetic Diseases
Genetic diseases represent a significant category of disorders attributed directly to mutations within genes. These can be classified into two primary groups: monogenic and complex diseases.
Monogenic Disorders
Monogenic disorders arise from alterations in a single gene. The major characteristic of these disorders is their clear inheritance patterns, making them relatively straightforward to study. Cystic fibrosis and sickle cell anemia are examples of monogenic disorders that highlight the direct connection between specific gene mutations and disease manifestation. This simplicity helps researchers and clinicians pinpoint genetic changes and understand their consequences. The advantage of focusing on monogenic disorders in this article lies in their potential for targeted intervention. Advances in gene therapy offer hope for curing, or at least managing, these diseases effectively. However, on the downside, the rarity of some monogenic disorders complicates research and treatment due to limited case numbers and funding.
Complex Diseases
Complex diseases, such as diabetes and heart disease, involve mutations in multiple genes and are influenced by environmental factors. Unlike monogenic disorders, complex diseases showcase a multifactorial inheritance, which makes them more challenging to understand and treat. The interaction between numerous genetic variations and external influences contributes to the variability in disease presentation among individuals. This complexity emphasizes the need for research that incorporates both genetic and environmental parameters. A notable feature of complex diseases is the ongoing research into genetic predispositions which could facilitate early detection and prevention strategies. However, the intricate nature of these diseases can hinder the development of treatments, as targeting one genetic component may not yield significant effect.
Cancer and Mutations
Cancer serves as a profound example of how gene mutations can have pathological implications. The relationship between mutations and cancer is multifaceted, encompassing both inherited and acquired mutations. Inherited mutations may predispose individuals to certain cancers, while acquired mutations typically develop as a result of exposure to environmental factors, lifestyle choices, or replicative errors during cell division.
The understanding of these mutations can lead to more accurate cancer diagnosis and innovative treatment modalities, including personalized medicine approaches that specifically target the genetic mutations present in a tumor. Furthermore, studies into oncogenes and tumor suppressor genes provide insight into the cellular mechanisms behind tumorigenesis.
"Understanding both genetic and environmental factors in cancer can propel us toward more effective prevention and treatment strategies."
"Understanding both genetic and environmental factors in cancer can propel us toward more effective prevention and treatment strategies."
Techniques for Studying Gene Mutants
Studying gene mutants requires precise and effective techniques. These techniques allow scientists to explore mutations in depth. Understanding these methods is critical to gain insights into gene functions and related biological processes. As we delve into the nature of gene mutants, the methods for studying them can reveal their significance in evolution, medicine, and biotechnology.
Researchers use various advanced technologies to analyze gene alterations. Both genetic sequencing and gene editing tools have become essential in this field. Each method provides unique insights. Moreover, they enable the exploration of gene functions and the examination of mutant effects.
Genetic Sequencing Technologies
Genetic sequencing plays a fundamental role in studying gene mutants. It allows scientists to decipher the DNA sequence of genes and identify mutations. Two main types of sequencing technologies are widely used: Sanger sequencing and Next-Generation sequencing. Both contribute significantly to genetic research.
Sanger Sequencing
Sanger sequencing is one of the oldest and most recognized sequencing methods. This technique involves amplifying a specific DNA segment and then determining its nucleotide sequence. The main characteristic of Sanger sequencing is its high accuracy and reliability. For many years, it was considered the gold standard in sequencing technology.
Sanger sequencing has a few specific advantages. It is particularly beneficial when sequencing smaller genomes or targeted regions. Researchers appreciate its straightforward method of detecting mutations. However, it does come with limitations. The process can be time-consuming and is generally less efficient for analyzing large genomes.
"Sanger sequencing remains a valuable tool, especially for targeted gene studies."
"Sanger sequencing remains a valuable tool, especially for targeted gene studies."
Next-Generation Sequencing
Next-Generation sequencing (NGS) has transformed genetic research. This advanced technology enables the simultaneous sequencing of millions of fragments of DNA. As a result, it provides a comprehensive overview of entire genomes quickly and at a lower cost compared to Sanger sequencing.
A key characteristic of NGS is its high-throughput capability. This allows for extensive genomic data collection in a relatively short timeframe. Its unique feature is the ability to identify not just single nucleotide variations, but also larger structural mutations across genomes.
NGS has numerous advantages: it is scalable, efficient, and cost-effective. However, it also encounters challenges, such as data complexity and storage issues due to massive datasets generated.
Gene Editing Tools
Gene editing tools have also revolutionized the study of gene mutants. They allow precise alterations to the genetic code. Two prominent tools are CRISPR-Cas9 and TALENs. Each of these has its strengths and specific applications in genetic research.
CRISPR-Cas9
CRISPR-Cas9 is a cutting-edge gene editing technology. This method utilizes a guide RNA molecule to lead the Cas9 enzyme to a specific DNA sequence. The primary contribution of CRISPR-Cas9 is its efficiency and precision in making targeted edits to the genome.
A significant characteristic of CRISPR-Cas9 is its simplicity. It is easier to design guide RNAs compared to other gene-editing methods. The unique feature of this system is its ability to induce double-strand breaks in DNA, paving the way for various edits. Its advantages include rapid modification and versatility in different organisms. Yet, ethical concerns and off-target effects are issues that researchers must carefully consider.
TALENs
TALENs are another gene editing tool that presents specific advantages. TALENs rely on customizable DNA-binding domains that target precise sequences in the genome. This method holds significant potential for correcting gene defects caused by mutations.
The advantage of TALENs is their high specificity. This characteristic reduces the likelihood of unintended edits, a common concern in gene editing. However, TALENs are more complex to design and less versatile than CRISPR-Cas9. Therefore, while TALENs have their merits, researchers might prefer CRISPR-Cas9 for its overall efficiency.
In summary, techniques for studying gene mutants are numerous and varied. Genetic sequencing technologies offer crucial insights into the structure of genes. In contrast, gene editing tools enable targeted modifications that can elucidate gene functions. Together, these methods are vital for advancing our understanding of gene mutants and their broad implications in science.
Ethical Considerations in Genetic Research
The ethical considerations in genetic research are paramount for shaping the future of biotechnology and medicine. As we delve deeper into gene editin, mutants, and genetic modifications, the potential for both beneficial and adverse outcomes intensifies. It is essential to balance innovation with social, ethical, and legal considerations. In this context, we must examine the principles that guide genetic research and address the concerns they raise.
Ethics of Gene Editing
Gene editing technologies, like CRISPR-Cas9, present a revolutionary leap in our ability to modify organisms at a genetic level. However, their power also brings significant ethical dilemmas. Key concerns include:
- Informed Consent: Researchers must ensure that studied subjects understand the implications of genetic modifications. This is critical when engagine in potentially life-altering treatments.
- Safety and Unintended Consequences: Engaging in gene editing can lead to unpredictable results. Thus, careful deliberation is required to evaluate the effects of altering genetic material.
- Equity and Access: If advanced gene editing techniques become widely available, disparities in access could escalate, potentially creating a divide between those who can afford genetic enhancements and those who cannot. This raises questions around fairness in society.
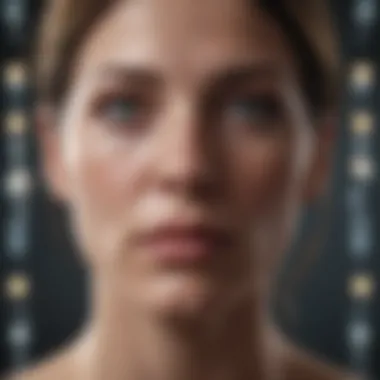
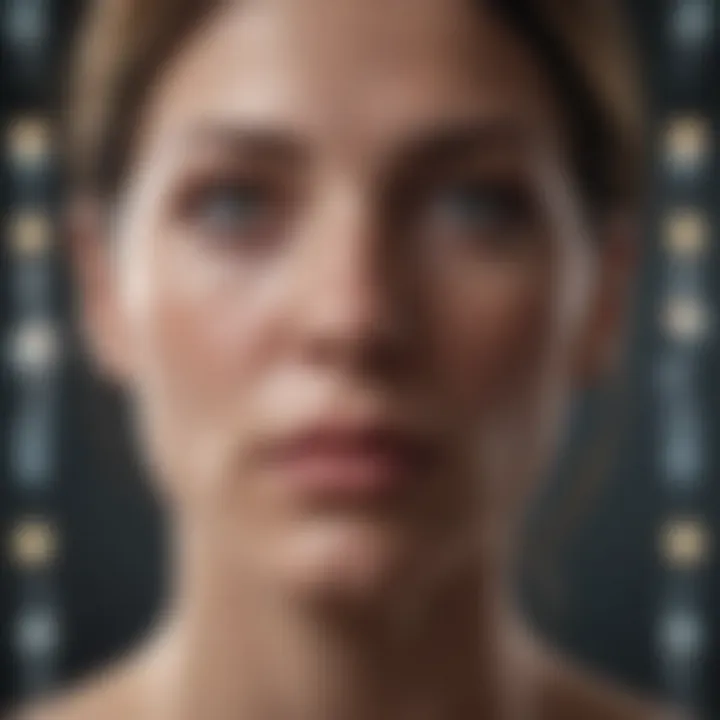
As these aspects unfold, ethical guidelines are needed. They should aim to protect individuals and communities from harm, while also fostering innovation.
Societal Impacts of Genetic Modifications
The adoption of genetic modifications resonates profoundly throughout society. As we consider these impacts, several topics are worthy of discussion.
- Public Perception: How society views genetic enhancements can shape the future of this research. Some may embrace the benefits, while others could resist due to ethical or moral reasons.
- Impact on Biodiversity: Genetically modified organisms (GMOs) can drastically affect ecological balance. Alterations to species may lead to unforeseen consequences for native flora and fauna. Understanding these dynamics is crucial for maintaining ecological integrity.
- Cultural Implications: Regions with strong cultural beliefs regarding nature and life may respond differently to genetic modifications. The dialogue between scientific progress and cultural sensitivity is vital for fostering acceptance and understanding.
"Ethical principles in genetic research must guide actions with respect to all forms of life, ensuring that humanity and biosphere coexist sustainably."
"Ethical principles in genetic research must guide actions with respect to all forms of life, ensuring that humanity and biosphere coexist sustainably."
In summary, as we move forward in the study and application of gene mutants, it is essential to prioritize ethical considerations. Engaging in open discussions about the implications of gene editing and modifications encourages transparency and fosters a sense of responsibility. Therefore, advancing genetic research should not only focus on the breakthroughs but also meticulously contemplate its societal consequences.
Future Directions in Gene Mutant Research
As the field of genetics evolves, exploring future directions in gene mutant research becomes essential. Advances in technology and methodology present new opportunities and challenges. More than ever, understanding gene mutants' role can lead to groundbreaking applications in medicine, agriculture, and environmental science.
Emerging Technologies
Technological innovation drives gene mutant research. Two key areas that stand out in this landscape are advanced gene-editing techniques and next-generation sequencing. These tools allow researchers to modify specific genes with remarkable precision.
- Gene Editing: The CRISPR-Cas9 system plays a crucial role. In essence, this technique enables the targeted alteration of DNA in living organisms. With it, scientists can correct genetic disorders and improve crop resilience.
- Next-Generation Sequencing (NGS): This technology helps quickly sequence large amounts of DNA. NGS allows for a comprehensive understanding of mutations across different genes. By identifying gene mutants at a high resolution, more tailored therapeutic interventions become possible.
Emerging technologies are invaluable for pushing the boundaries of current knowledge and practice in genetic research. Their implications can have profound effects not just on a scientific level but on societal well-being as well.
The Role of Computational Biology
Computational biology increasingly shapes gene mutant research. This area applies algorithms, models, and simulations to biological data. The following aspects highlight its importance:
- Data Analysis: With complex sequencing data, computational biology simplifies understanding. Algorithms can identify patterns in mutations, helping to predict their impact on phenotype and function.
- Modeling Mutations: Computational approaches allow researchers to simulate mutation effects on genes. Such modeling can forecast evolutionary pathways and assist in drug development by simulating how mutants respond to different treatments.
- Integration with Big Data: The rise of big data means vast amounts of genetic information are available for analysis. Computational biology integrates these data sets, providing insights that would be unattainable through traditional methods.
Applications of Gene Mutants in Biotechnology
Understanding the applications of gene mutants in biotechnology is crucial because they offer significant advancements in various fields, particularly agriculture and medicine. Gene mutations allow scientists to modify organisms and develop products that not only enhance human life but also address environmental challenges. This topic delves into the importance of gene mutants by highlighting specific strategies, advantages, and factors concerning their applications.
Genetically Modified Organisms
Genetically modified organisms (GMOs) are one of the most prominent applications of gene mutants. These organisms are created by altering their genetic material, resulting in desired traits that improve growth, yield, or resistance to pests and diseases. The process often involves using specific gene mutants that convey these beneficial properties.
In agriculture, crops such as Bt maize and Roundup Ready soybeans have been genetically engineered to express traits which enhance their viability. For example, Bt maize contains a gene from the bacterium Bacillus thuringiensis, which produces a protein toxic to specific pests. This innovation reduces the need for chemical pesticides, promoting a more sustainable agricultural practice.
Additionally, genetically modified organisms are important in addressing food security. As the global population continues to rise, enhancing crop yields through genetic modification can help feed more people while utilizing fewer resources. The development of drought-resistant varieties also aids farmers in climates where water is scarce, further securing agricultural productivity.
"The ability to create genetically modified organisms is a powerful tool for scientists looking to address pressing problems in food production and sustainability."
"The ability to create genetically modified organisms is a powerful tool for scientists looking to address pressing problems in food production and sustainability."
Biopharmaceuticals Development
The impact of gene mutants on pharmaceutical development is equally noteworthy. Biopharmaceuticals derived from genetically modified organisms dramatically revolutionize the treatment of diseases. They often include therapeutic proteins, antibodies, and vaccines that conventional techniques cannot produce efficiently.
For instance, insulin production for diabetes patients has benefited from genetically modified yeast and bacteria. These microbes can be engineered to synthesize human insulin, allowing for a more reliable and efficient production method compared to traditional extraction from animal sources.
Moreover, the use of gene mutants in vaccine development has accelerated responses to emerging infectious diseases. Genetic engineering methods streamline the creation of vaccines that are safer and more effective. mRNA vaccines, such as those developed for COVID-19, showcase how advances in biotechnology are possible through the manipulation of gene mutants. These vaccines prompt the body's immune response without using live virus, significantly reducing risk.
The development of gene therapy also holds tremendous promise. By employing viral vectors carrying corrected gene sequences, scientists can target and treat genetic disorders directly at the source. This technique demonstrates how gene mutants can lead to breakthroughs in personalized medicine, tailoring treatments to individual genetic makeups.
In summary, the applications of gene mutants in biotechnology are vital for enhancing food production and transforming healthcare. Both genetically modified organisms and biopharmaceuticals reveal the profound potential of manipulating genetic information. As research progresses, these applications will continue to develop, yielding solutions to many of the challenges faced in modern society.
The End
The conclusion serves as a critical component in understanding the broader implications of gene mutants. It synthesizes the knowledge acquired in previous sections, reinforcing the interconnectedness of gene mutations in various domains of study.
A fundamental understanding of gene mutations is essential for recognizing their role in evolutionary biology. These mutations are not merely incidental changes; they create diversity within populations, enabling natural selection to occur. Awareness of how these changes shape biological systems can lead to more effective conservation strategies as well as advancements in understanding species evolution.
In medicine, grasping the implications of gene mutations allows researchers and practitioners to better comprehend genetic diseases. For instance, recognizing how specific mutations result in monogenic disorders enhances diagnostic capabilities. Likewise, the relationship between cancer and mutations emphasizes the need for continued exploration in this area. This understanding can lead to more precise therapeutic approaches, illustrating the importance of genetic research in health care.
Biotechnology is another field strongly influenced by the study of gene mutants. Applications that range from genetically modified organisms to the development of biopharmaceuticals highlight the practical benefits of this knowledge. Understanding how gene mutants can be utilized promotes innovation and can address global challenges such as food security and disease management.
Importance of Including References
- Credibility: References strengthen the article's reliability. Relying on credible studies helps to build trust with the audience, especially those who are well-versed in this area.
- Further Exploration: Including references allows readers to pursue deeper understanding beyond the content of the article. It opens pathways for further research, which is intrinsic to scientific learning.
- Acknowledgment of Work: References recognize the contributions of previous researchers. This fosters an environment of respect and collaboration in the scientific community.
- Facilitates Understanding: For complex topics like gene mutations, referenced materials can break down concepts into more digestible forms. They provide a context, enhancing comprehension, especially for students and new researchers.
Benefits of Citing Diverse Sources
A diverse selection of references adds depth to the discussion. When references come from various types of sources, such as journals, books, and reputable websites, they provide a more comprehensive view of the current state of research. Readers benefit from different perspectives that challenge or support existing knowledge.
"A reference is more than a citation; it is a bridge between researchers, their ideas, and their discoveries."
"A reference is more than a citation; it is a bridge between researchers, their ideas, and their discoveries."
Considerations for References
When integrating references into an article, it is essential to be selective. Ensure that cited works are recent and relevant. Additionally, differentiating between primary and secondary sources can enhance the quality of the references. Primary sources present original research findings, while secondary sources offer analyses or interpretations of these findings.
To conclude, the importance of references in discussing gene mutants cannot be overstated. They provide validity, encourage further inquiry, and acknowledge the foundations upon which current understanding stands. Attention to the quality and variety of references can greatly enrich the discourse surrounding gene mutations.