Understanding the GFP cDNA Sequence: Applications
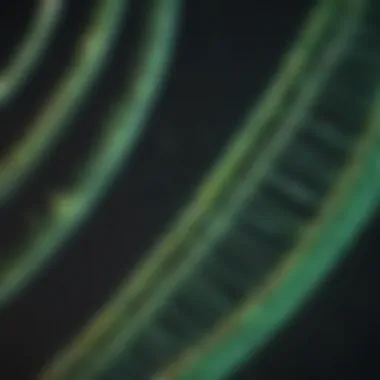
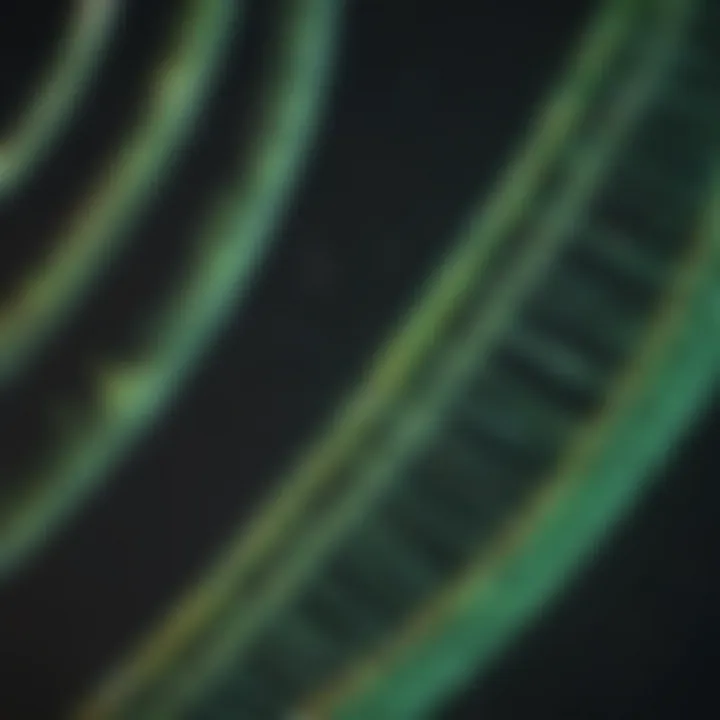
Intro
The green fluorescent protein (GFP) cDNA sequence stands at the forefront of many biological research pursuits. Understanding its nuances offers profound insights into cell biology and genetic engineering. The manipulation and analysis of the GFP cDNA sequence have opened up new pathways in research methodology and biotechnological advancements. This article will guide you through the significance of the GFP cDNA sequence, focusing on its structure, function, and varied applications.
Key Concepts
Definition of the Main Idea
The GFP cDNA sequence is derived from the jellyfish Aequorea victoria, where it was first discovered. This sequence encodes the green fluorescent protein, which exhibits bright green fluorescence when exposed to ultraviolet light. It is crucial in many experimental techniques, serving as a marker for gene expression studies. The unique properties of GFP make it a valuable tool in visualizing cellular processes in real-time.
Overview of Scientific Principles
The GFP's ability to fluoresce is a result of its specific amino acid composition, which allows it to absorb light energy and emit it at a different wavelength. This capability is grounded in the principles of fluorescence and biochemistry. Understanding these scientific principles not only allows researchers to utilize GFP effectively but also encourages innovations in how we observe biological processes.
Current Research Trends
Recent Studies and Findings
Recent studies have expanded our understanding of GFP applications. For example, advancements in fluorescence microscopy have enabled scientists to observe live cellular dynamics with unparalleled clarity. Researchers are constantly exploring novel ways to enhance GFP’s efficiency and brightness, as well as creating new variants that extend its color palette, addressing some limitations of the original protein.
Significant Breakthroughs in the Field
The development of optimized GFP derivatives, such as mCherry and mKate, represents a noteworthy breakthrough. These derivatives allow for simultaneous multicolor labeling, which significantly enhances the analysis of complex biological systems. Notably, innovations have also been made in the areas of gene delivery systems, where GFP is employed to evaluate the success of transfection methods.
"The GFP cDNA sequence has transformed how we study and understand genetic expression. Its impact spans multiple disciplines within biological sciences."
"The GFP cDNA sequence has transformed how we study and understand genetic expression. Its impact spans multiple disciplines within biological sciences."
Preamble to GFP cDNA
The study of green fluorescent protein (GFP) cDNA is of paramount importance in modern biological research. Understanding GFP cDNA provides critical insights into gene expression, cell biology, and even applications in biotechnological innovations. This introduction sets the stage by elucidating significant aspects concerning GFP cDNA, its benefits, and considerations that researchers must take into account.
Definition and Overview
Green fluorescent protein (GFP) is originally derived from the jellyfish Aequorea victoria. The cDNA, which refers to complementary DNA, is synthesized from messenger RNA (mRNA) of the gene encoding GFP. This process is vital as it enables researchers to explore and manipulate the genes encoding GFP for various experimental applications.
The primary feature of GFP is its ability to fluoresce when exposed to ultraviolet or blue light, which makes it a powerful tool in biological assays. The cDNA sequence encodes not only the amino acid sequence of GFP but also contains regulatory elements crucial for transcription and translation. Understanding the cDNA sequence thus facilitates studies on gene expression and tracking of cellular processes.
Historical Context
The discovery of GFP was a significant advancement in molecular biology. Initial studies of this protein date back to the 1960s, but it wasn't until the 1990s that it gained widespread attention in the scientific community. Researchers such as Osamu Shimomura, who isolated GFP in 1962, laid the groundwork for its application in genetic and cellular studies. The later work by Martin Chalfie and Roger Tsien, who utilized GFP in cells of model organisms, brought the protein into mainstream scientific research, resulting in a Nobel Prize in 2008.
Since its inception, GFP has revolutionized imaging techniques in live cells, aiding in the visualization of cellular structures and functions in real time. Consequently, the historical advancements in the isolation and characterization of GFP cDNA reflect an ongoing evolution in scientific exploration, rendering GFP a cornerstone in molecular biology.
The journey of GFP from a jellyfish protein to a fundamental tool in modern science illustrates the interconnectedness of discovery and application.
The journey of GFP from a jellyfish protein to a fundamental tool in modern science illustrates the interconnectedness of discovery and application.
Understanding these foundational elements offers insight into how GFP cDNA can be utilized effectively in current and future research endeavors.
Biological Function of GFP
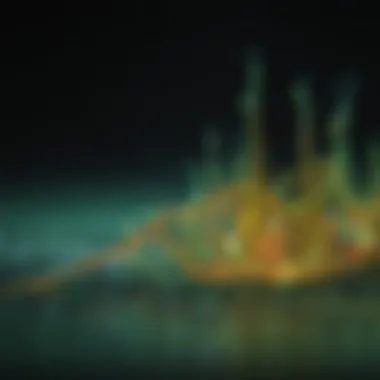
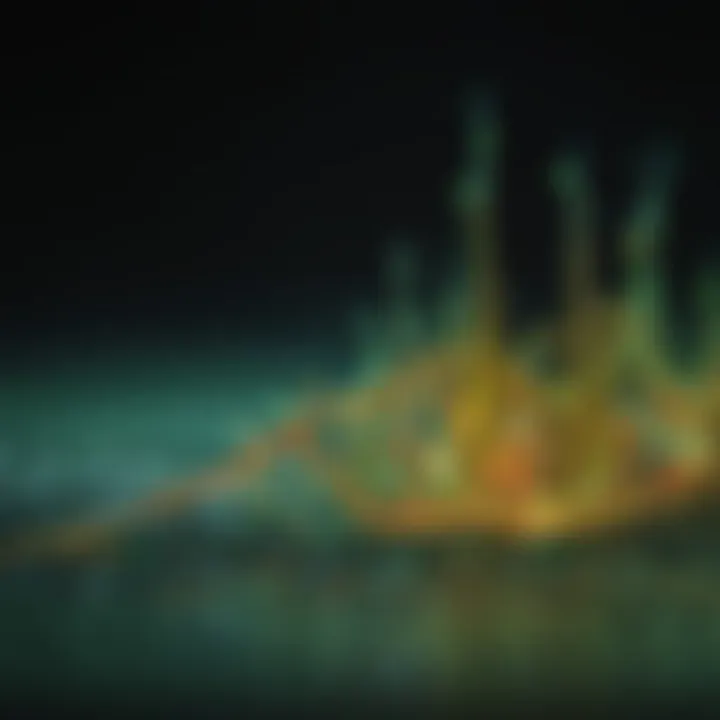
The biological function of the green fluorescent protein (GFP) is fundamental to understanding its implications in various scientific fields. GFP serves as a powerful tool in cell biology, providing insightful information about cellular processes. Its intrinsic fluorescence allows researchers to visualize and track proteins and cellular components in living organisms. The significance of GFP extends beyond mere aesthetics; its utility in experimental design enhances researchers’ ability to study complex biological phenomena.
Mechanism of Fluorescence
GFP's remarkable fluorescence is a product of its unique molecular structure. This protein contains a chromophore, which is crucial for its ability to absorb and emit light. The chromophore’s formation occurs through the post-translational modification of specific amino acids within the protein. When exposed to ultraviolet or blue light, the chromophore absorbs energy, which it later releases as visible green light. This property has made GFP an indispensable marker in molecular biology.
The efficiency of this fluorescence can be influenced by several factors, including pH levels, temperature, and the presence of specific ions. A deeper understanding of the mechanisms that control GFP's optical properties is essential for developing improved variants of the protein. Variants enhance its brightness, alter its emission spectra, or increase its stability under various experimental conditions.
Role in Cellular Studies
GFP's impact in cellular studies is profound. Scientists utilize GFP-tagged proteins to observe live cellular activities in real-time. This tracking capability is crucial for studying dynamic processes such as protein localization, interaction, and turnover rates. For example, researchers can use GFP to visualize the movement of cellular organelles or the trafficking of proteins within the cell. This allows for more precise mapping of cellular functions and behaviors in response to different stimuli.
Moreover, GFP has been integral in genetic studies where researchers fuse GFP to target genes, enabling the direct observation of gene expression patterns in various tissues. This approach offers insights into developmental biology and gene regulation. In addition, GFP can aid in identifying cellular responses to environmental changes or drug treatments, thus enhancing the understanding of cellular physiology.
GFP has revolutionized the way scientists study cellular mechanisms by offering a non-invasive method to monitor biological processes in real time.
GFP has revolutionized the way scientists study cellular mechanisms by offering a non-invasive method to monitor biological processes in real time.
In summary, the biological function of GFP represents more than just a fluorescent marker; it epitomizes a breakthrough in cellular biology. Its mechanism of fluorescence allows for innovative experimental designs, and its role in cellular studies provides unparalleled insights into the dynamic world of cells.
Isolation of GFP cDNA Sequence
The isolation of the green fluorescent protein (GFP) cDNA sequence presents significant implications in both basic research and applied sciences. This process enables researchers to harness the fluorescent properties of GFP for a myriad of applications, including gene expression studies, cellular tracking, and various biotechnological innovations. Understanding how to effectively isolate the GFP cDNA sequence is fundamental for any scientist working in molecular biology or genetic engineering.
When isolating cDNA sequences, it is crucial to start with high-quality RNA extracted from cells expressing GFP. The choice of method for isolation can greatly affect the outcome. There are several considerations that come into play during this process:
- Quality of RNA: The integrity of the RNA is paramount. Degraded RNA can lead to incomplete or erroneous cDNA synthesis, affecting downstream applications.
- Specificity: Isolating specifically for GFP cDNA requires precise techniques to ensure that other unrelated cDNA sequences do not interfere.
- Yield: A successful isolation must produce a sufficient amount of cDNA for subsequent experiments.
By paying attention to these factors, researchers can maximize the benefits derived from isolating the GFP cDNA sequence.
Methods of cDNA Synthesis
The methods of cDNA synthesis are pivotal for obtaining high-quality GFP cDNA. There are multiple approaches, each with its advantages. Commonly used techniques include:
- Reverse Transcription-PCR (RT-PCR): This method converts RNA to cDNA by using reverse transcriptase. It is widely used due to its sensitivity and efficiency in synthesizing cDNA.
- Poly(A) Tailing: By adding a poly(A) tail to the RNA template before reverse transcription, researchers can enhance the specificity of the cDNA synthesis. This technique favors the mRNA molecules that typically have a poly(A) tail, resulting in a more enriched product.
- Single-Stranded cDNA: Isolation of single-stranded cDNA can be advantageous for certain applications, particularly those requiring unique amplification methods.
Each of these methods has unique benefits in yielding high-quality cDNA that retains the essential properties needed for advanced applications such as transient expression studies or for use in localization assays.
PCR Amplification Techniques
PCR amplification is a crucial step that follows cDNA synthesis. It allows for the selective amplification of the GFP cDNA, making it easier to analyze. Different PCR techniques can be utilized during this stage:
- Standard PCR: This method uses primers specific to the GFP sequence. It is straightforward and effective, allowing researchers to amplify specific regions of the cDNA.
- Quantitative PCR (qPCR): This advanced technique offers the ability to quantify the amount of cDNA synthesized, providing insights into gene expression levels. This can be particularly informative in understanding the role of GFP in various biological processes.
- Nested PCR: By using two sets of primers, this method increases specificity and sensitivity, which is critical when working with cDNA that may be present in low amounts.
Overall, mastering these PCR amplification techniques enhances the research capabilities regarding the GFP cDNA sequence, allowing for greater exploration into its implications and applications.
Analysis of GFP cDNA Sequence
The analysis of the GFP cDNA sequence is vital for various reasons in the understanding of green fluorescent protein and its applications in research and biotechnology. By analyzing this sequence, researchers can delve into the genetic, structural, and functional aspects of GFP, paving the way for a deeper understanding of its role in biological systems. The implications of this analysis extend beyond theoretical knowledge, influencing practical applications in genetic engineering, microscopy, and cellular biology.
Sequencing Approaches
Sequencing approaches for GFP cDNA are critical for producing a complete understanding of its genetic makeup. Techniques such as Sanger sequencing and next-generation sequencing (NGS) are commonly employed. Each method has its own merits and limitations.
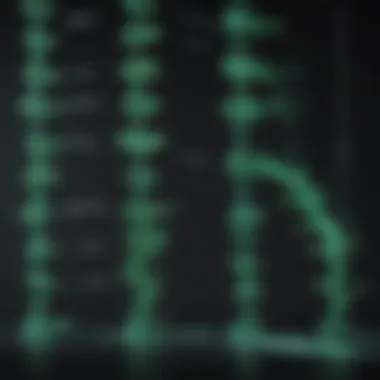
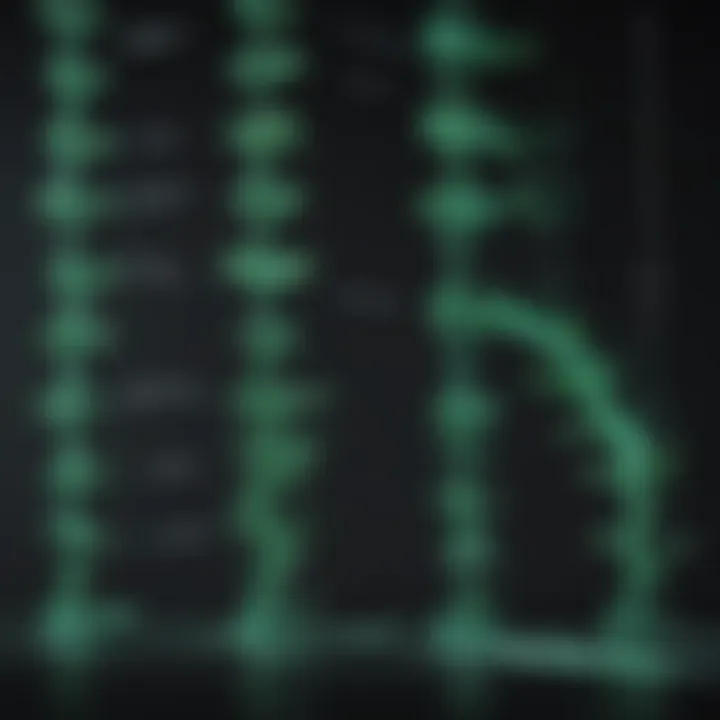
- Sanger Sequencing: This method is often the go-to for smaller sequences and provides high accuracy. It allows researchers to verify specific segments of the GFP cDNA, making it useful for confirming the sequences from cDNA synthesis.
- Next-Generation Sequencing: This technique enables massive parallel sequencing, making it suitable for analyzing large fragments of GFP cDNA. It provides greater throughput and the ability to analyze multiple samples simultaneously.
Each of these sequencing approaches contributes to a comprehensive understanding of the GFP cDNA sequence by enabling researchers to identify mutations, analyze expression levels, and assess sequence variations.
Bioinformatics Tools
Bioinformatics tools play a vital role in the analysis of GFP cDNA sequences. These tools assist not only in storing and processing large datasets but also in interpreting the biological significance of the data obtained.
Specific tools include:
- BLAST: This tool allows researchers to compare their GFP sequences against a database of known sequences. It helps in identifying homologous sequences and understanding evolutionary relations.
- Geneious: This application integrates multiple bioinformatics features for sequence alignment, assembly, and annotation, providing a user-friendly approach to sequence analysis.
- MEGA Software: Used for molecular phylogenetics, MEGA enables researchers to conduct comparative analyses of GFP sequences across different species.
- UC Davis Campus Genomics Portal: This portal facilitates access to genomic data specific to various organisms, enhancing the research capabilities of scientists working with GFP.
Through the utilization of these bioinformatics tools, researchers can effectively analyze data, thus aiding in the discovery of new applications and improving existing methodologies associated with GFP cDNA.
Applications of GFP cDNA in Research
The applications of GFP cDNA are vast and crucial to modern biological research. Understanding its implications allows scientists to utilize this tool effectively. The GFP cDNA sequence is a versatile molecular tool that has transformed cellular imaging, genetic studies, and biotechnology. By enabling researchers to visualize and track cellular processes in real time, GFP cDNA offers significant advantages over traditional methods. This section will explore its various applications, including gene expression studies, tracking cellular processes, and driving biotechnological innovations.
Gene Expression Studies
The use of GFP cDNA is prevalent in gene expression studies. Researchers employ it to assess the activity of specific genes under various conditions. Utilization of GFP provides a direct method to visualize gene expression in live cells without destroying them. This capacity supports real-time observation, allowing researchers to gather more nuanced data.
Methods such as fusion with promoter sequences enable specific gene tracking. For instance, if a gene of interest is tagged with GFP, its expression can be monitored in terms of timing and location. This insight is invaluable for understanding development, cellular responses to stimuli, and differentiation processes.
Furthermore, combining GFP with advanced imaging techniques, such as confocal microscopy, enhances visual clarity. The quality of data achieved through GFP-based methods is superior, thus bolstering scientific conclusions.
Tracking Cellular Processes
Another prominent application of GFP cDNA is in tracking dynamic cellular processes. GFP allows researchers to observe intricate intracellular events in real time. This capability is essential for studying processes like protein localization, cellular signaling pathways, and interactions within cells.
"GFP acts as a beacon, illuminating the otherwise hidden intricacies of cellular interactions."
"GFP acts as a beacon, illuminating the otherwise hidden intricacies of cellular interactions."
For example, researchers may attach GFP to proteins involved in signaling paths. This tagging aids in understanding how different proteins interact and perform their biological roles. Studies that rely on GFP can reveal how cells react to external stimuli and how they undergo changes such as division, migration, or apoptosis.
Moreover, using GFP in live-cell imaging can elucidate disease mechanisms. By monitoring cells affected by diseases, such as cancer, researchers can track metastatic behavior or changes in enzyme activity, all critical for developing therapeutic interventions.
Biotechnological Innovations
The versatility of GFP cDNA extends into biotechnological innovations. It holds significant potential in various fields, including medicine, agriculture, and environmental science. One major area of interest is gene therapy. Here, GFP serves as a marker for successfully delivered therapeutic genes into target cells, assessing the effectiveness of treatment methods.
In agriculture, GFP has been applied to create genetically modified organisms that express desired traits. For instance, plants engineered to express GFP can be monitored for growth responses and the effects of environmental stresses. This work aids researchers in producing crops with improved resistance traits.
Additionally, innovations in synthetic biology frequently utilize GFP as a reporter gene. This application helps in developing engineered systems that can respond dynamically to environmental changes. Overall, the integration of GFP cDNA influences numerous fields, underscoring its importance in pushing scientific boundaries.
Current Challenges in GFP Research
The exploration of green fluorescent protein (GFP) cDNA has advanced significantly, yet it faces several challenges that impact its utility in research. Understanding these challenges is crucial for anyone involved in biological studies involving GFP. This section will analyze specific issues that researchers often encounter, focusing on stability, photobleaching, and limitations in specific applications. Recognizing these obstacles can inform better practices and innovations moving forward.
Stability and Photobleaching Concerns
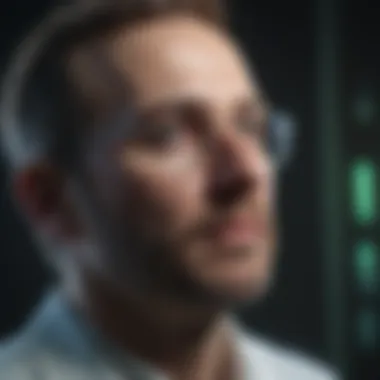
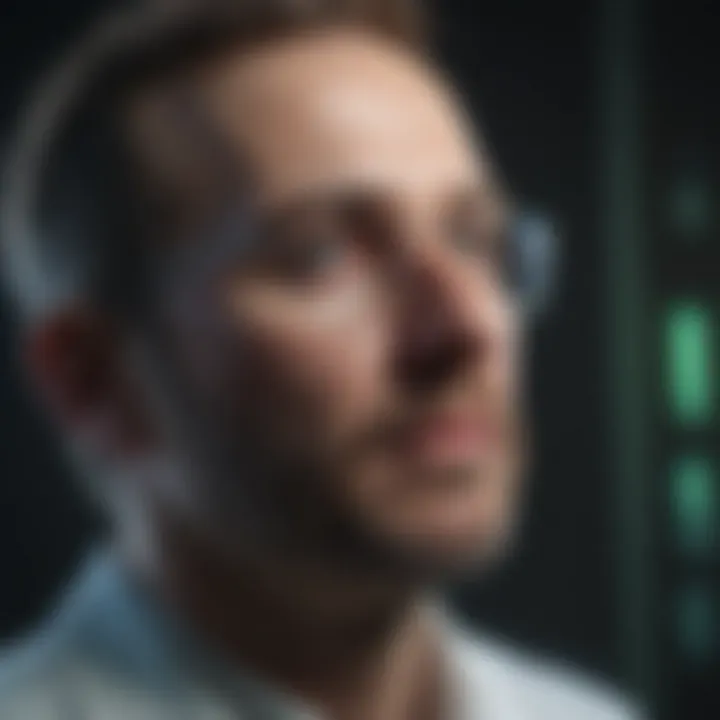
One significant challenge is the stability of GFP under various experimental conditions. GFP is sensitive to environmental factors, including
- temperature fluctuations,
- pH changes, and
- ionic strength.
These variables can lead to a loss of fluorescence intensity, undermining the reliability of results. Photobleaching, the process where fluorescent molecules lose their ability to fluoresce due to prolonged exposure to light, poses another serious concern. The intensity of the fluorescence diminishes over time, which can compromise any long-term imaging experiments.
Small modifications in the structure of GFP can potentially enhance its stability and resistance to photobleaching. Researchers continue to investigate new variants of GFP with improved fluorescent properties. This can help mitigate issues related to unstable results and fading signals, ensuring more accurate data collection over extended periods.
"Improving the stability of GFP is essential to maintain its value as a marker in cellular imaging."
"Improving the stability of GFP is essential to maintain its value as a marker in cellular imaging."
With careful consideration of the environment in which GFP is used, as well as employing advanced imaging techniques, researchers can address some of these limitations more effectively.
Limitations in Specific Applications
While GFP serves as a powerful tool in many research contexts, it does have limitations in specific applications. Certain issues include:
- Tissue Penetration: Fluorescent signals weaken significantly when passing through dense tissues, reducing the utility of GFP in whole organism imaging.
- Signal Overlap: In multicolor imaging, GFP’s emission spectrum can overlap with that of other fluorophores, complicating data interpretation.
- Cellular Context: The expression levels of GFP can vary widely depending on cell type and experimental conditions, leading to inconsistent results across studies.
Research teams must consider these issues when designing experiments. Alternative fluorescent proteins, such as mCherry or mCitrine, may be used in conjunction with GFP to overcome some limitations related to signal overlap. Additionally, using advanced imaging techniques, like two-photon microscopy, may enhance tissue penetration, allowing for better visualization in vivo.
Future Perspectives on GFP cDNA Usage
The exploration of the GFP cDNA sequence continues to unveil promising avenues for future research and applications in diverse biological fields. Understanding these future perspectives is crucial for researchers, educators, and students, as they highlight the innovations that can stem from GFP cDNA technologies. The ongoing evolution in this area emphasizes the need for adaptive methodologies and intricate analysis.
Emerging Technologies
Recent advancements in genetic engineering, synthetic biology, and imaging technologies open new doors for GFP cDNA applications. Underlying these advancements is the growing capability to modify genetic constructs with precision. Techniques such as CRISPR-Cas9 enable researchers to insert GFP sequences into specific genes, facilitating targeted gene expression studies.
Moreover, the integration of machine learning and artificial intelligence in bioinformatics can improve the analysis of fluorescent signal data, leading to more reliable quantification in various studies. Promising developments in super-resolution microscopy also enhance the observation of GFP-labeled proteins at molecular levels. Thus, combining GFP cDNA with cutting-edge technologies significantly enhances the potential for innovative applications in research.
Potential in Therapeutics
The therapeutic potential of GFP cDNA usage is extensive. GFP is not only a powerful marker for cellular components but also a tool for tracking therapeutic vectors in gene therapy. By tagging genes of interest with GFP, researchers can monitor the efficacy and distribution of these therapies in real-time. This is particularly vital in understanding the dynamics of drug delivery systems and the response of cellular environments to therapeutic interventions.
Furthermore, the use of GFP in stem cell research offers significant insights into differentiation processes and cellular reprogramming, which are critical in regenerative medicine. Utilizing GFP cDNA could provide substantial evidence of successful therapeutic outcomes at a cellular level. The multifaceted nature of GFP empowers it to become a staple in future therapeutic applications.
"The future of GFP cDNA holds the promise of transforming our approach toward cellular therapies and integrating advanced technologies to deepen our understanding of biological processes."
"The future of GFP cDNA holds the promise of transforming our approach toward cellular therapies and integrating advanced technologies to deepen our understanding of biological processes."
Closure
The conclusion serves a pivotal role in this article by succinctly encapsulating the significance of the GFP cDNA sequence in various biological applications. Understanding the implications of this sequence is not merely an academic exercise; it represents a crossing point of innovation across multiple fields such as molecular biology, biotechnology, and genetics. This encapsulation aids in redefining how researchers and practitioners view fluorescent proteins as tools in advancing scientific knowledge and practical applications in medicine and technology.
Summary of Key Points
The GFP cDNA sequence is a cornerstone in several biological processes and advancements. Here are the primary points summarized:
- Definition and Importance: The GFP cDNA represents the genetic blueprint for producing fluorescent proteins that are widely used in imaging.
- Biological Functions: This sequence elucidates the mechanistic understanding of fluorescence, allowing for comprehensive cellular studies.
- Methods of Isolation: Advanced techniques, such as PCR amplification, facilitate the extraction and analysis of the GFP cDNA.
- Applications in Research: The GFP cDNA sequence is instrumental in gene expression studies, as well as in tracking dynamic cellular processes.
- Current Challenges: Issues like photobleaching and application limitations present hurdles, prompting ongoing research and developments.
- Future Directions: Emerging technologies and potential therapeutic applications highlight the need for continuous exploration in this domain.
Call to Action for Further Research
The narrative surrounding GFP cDNA is far from complete. Future research should emphasize:
- Addressing Limitations: Investigate means to enhance the stability and usability of fluorescent proteins in varied environments.
- Innovating Applications: Explore novel biotechnological uses, particularly in gene therapy and cellular engineering.
- Cross-disciplinary Approaches: Encourage collaboration between biologists, chemists, and engineers to leverage the full potential of GFP cDNA applications in innovative ways.
Continued investigation into GFP and its applications ensures that this essential genetic sequence retains its place at the forefront of scientific discovery. By fostering a culture of inquiry and innovation, the full potential of GFP cDNA can be realized, expanding its usefulness in research and therapeutic contexts.