Understanding Histone H3 Molecular Weight and Its Implications
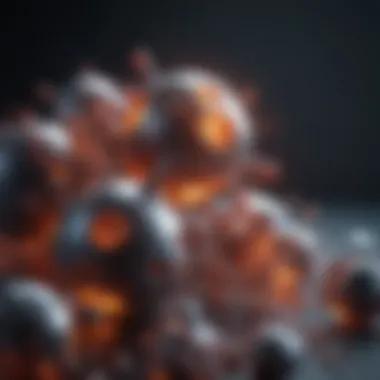
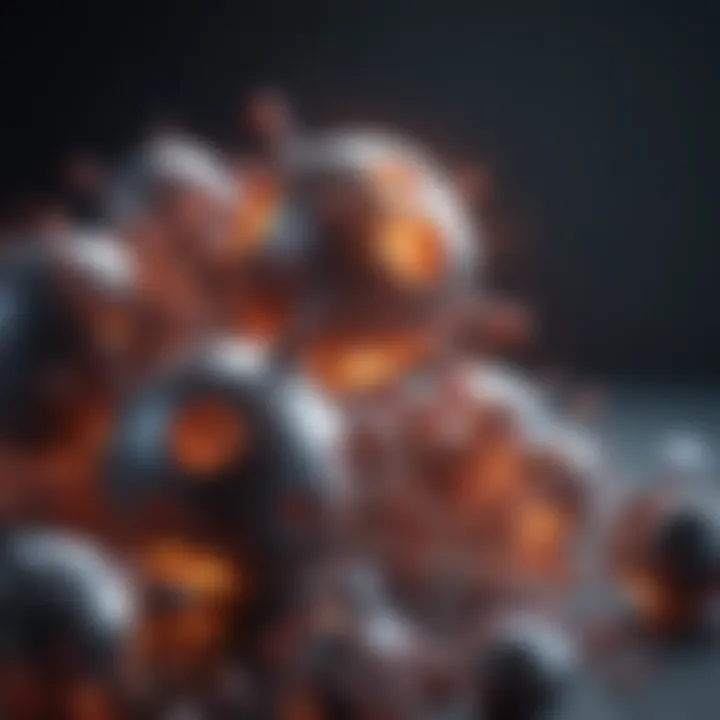
Intro
Histone is a key protein that plays vital roles in the organization and regulation of genetic material. Understanding its molecular weight is essential as it relates to various biological functions, particularly in the realm of epigenetics and gene expression. The molecular weight of histone reflects its structure, genetic variations, and how it interacts with other molecules within the cell.
By exploring the characteristics of histone , researchers can also gain insights into its influence on chromatin structure and dynamics. This article will explore the molecular weight of histone , including its importance in genetic regulation and modifications that can affect cellular processes.
Key Concepts
Definition of the Main Idea
Histone is one of the core proteins found in chromatin, which is the material that makes up chromosomes. Specifically, histone combines with other histones to form nucleosomes, wrapping around DNA. This arrangement plays a crucial role in regulating access to genetic information. The molecular weight refers to the mass of one molecule of histone and provides insight into its potential interactions and functions in the cell. Understanding this concept is fundamental for comprehending how histones contribute to gene regulation and epigenetic modifications.
Overview of Scientific Principles
The molecular weight of histone is approximately 15 kilodaltons (kDa). This value can vary based on post-translational modifications, including methylation or acetylation. These modifications can change both the function and binding affinity of histone , impacting gene expression dynamics.
Key principles related to histone include:
- Nucleosome Structure: Histone , along with A, B, and , forms the nucleosome that acts as the fundamental unit of chromatin.
- Gene Regulation: The modifications to histone can either promote or inhibit the transcription of associated genes.
- Epigenetics: Changes in the molecular weight due to modifications can have heritable effects on gene expression without altering the underlying DNA sequence.
"The role of histone modifications in the regulation of gene expression cannot be overstated; they are central to our understanding of the epigenetic landscape."
"The role of histone modifications in the regulation of gene expression cannot be overstated; they are central to our understanding of the epigenetic landscape."
Current Research Trends
Recent Studies and Findings
Recent literature highlights the significance of histone in various genetic processes. For example, studies have indicated that specific modifications of histone correlate with certain diseases, including cancer. Ongoing research seeks to connect the molecular weight variations of histone with potential therapeutic targets.
Significant Breakthroughs in the Field
Some breakthroughs include the development of advanced sequencing technologies capable of analyzing histone modifications on a genome-wide scale. These tools enhance our understanding of how the molecular weight of histone may correlate with gene expression patterns across different cell types. The exploration of histone has also led to insights into cellular aging and how these modifications can affect the lifespan of a cell.
In summary, a focused study of the molecular weight of histone offers a window into the complex interplay of genetics, epigenetics, and cellular functions. As research continues to expand in this area, the implications of histone modifications will likely lead to significant advancements in both basic science and clinical applications.
Prologue to Histone Proteins
Histone proteins play a foundational role in the structure and function of cellular chromatin. They are crucial for packaging DNA in a manner that allows for both accessibility and protection. This introduction highlights the multifaceted nature of histone proteins and their significance in molecular biology, particularly in the context of gene regulation and epigenetics.
Understanding histones is essential for comprehending how genetic information is organized and expressed in living organisms. Their interactions with DNA not only influence the basic architecture of the genome but also affect gene activity and cellular differentiation. In this article, we will explore various aspects of histone proteins, notably their classification, structural features, and functional roles in genetics.
Histones have essential benefits, including:
- Facilitating the compaction of DNA.
- Acting as structural components of chromatin.
- Serving as platforms for regulatory proteins that influence gene expression.
Given their importance, a nuanced understanding of histone proteins is vital for students, researchers, educators, and professionals in the field of genetics and molecular biology.
Overview of Histones
Histones are a class of highly conserved, basic proteins that are integral to the formation of chromatin. They are categorized into two main classes: core histones and linker histones. Core histones include A, B, , and , while the linker histone helps stabilize the structure of nucleosomes. Nucleosomes are the fundamental units of chromatin, consisting of DNA wrapped around a core of eight histone proteins.
The primary function of histones is to package DNA into a compact, organized structure. This packaging plays a critical role in regulating DNA accessibility and influencing various cellular processes, such as DNA replication, repair, and transcription. Their structure allows for dynamic interactions with the DNA and other proteins adjusting their activities depending on cellular needs.
Furthermore, histones are subject to various post-translational modifications. These modifications, which include acetylation, methylation, and phosphorylation, affect the interaction between histones and DNA, thereby influencing gene expression. The ability to modify histones adds another complex layer to their function, making them central players in gene regulation and epigenetic changes.
Significance of Histones in Genetics
The significance of histones within the realm of genetics cannot be understated. They serve as critical regulators of gene expression by controlling the accessibility of DNA to transcription factors and other proteins. Through various mechanisms, histones influence whether a gene is active or silent, depending on the state of chromatin.
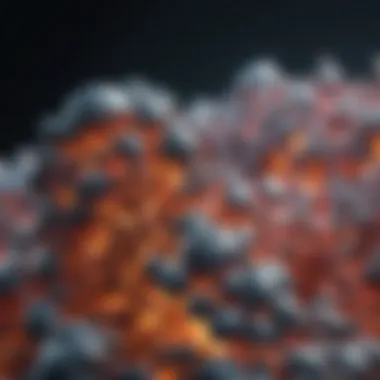
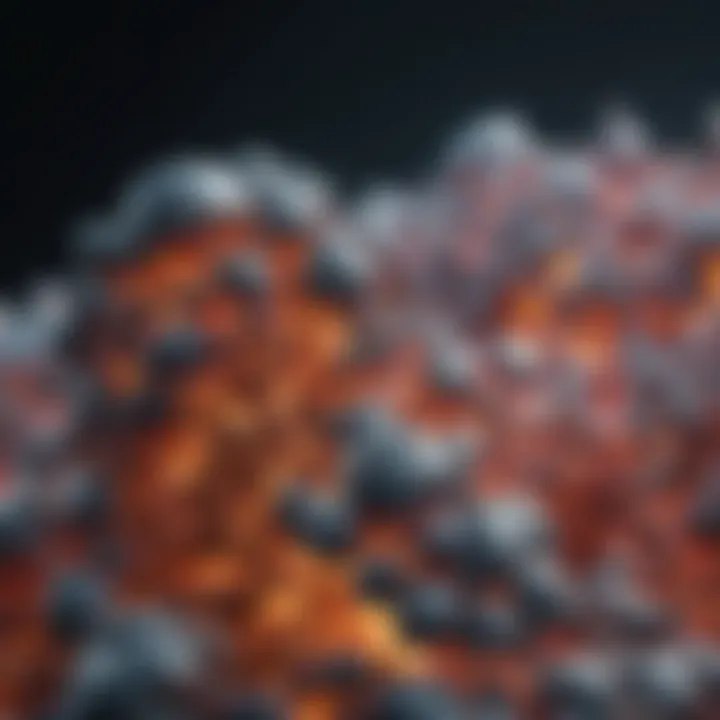
Histones are often associated with the concept of epigenetics, which refers to heritable changes in gene activity that do not involve alterations to the underlying DNA sequence. These changes are triggered by the chemical modifications of histones. As research advances, the connection between histone modifications and specific gene expression outcomes becomes clearer.
Some key points regarding the significance of histones include:
- Regulatory Function: Histones modulate gene expression patterns based on cellular signals.
- Epigenetic Memory: Through modifications, histones can help maintain gene expression patterns across cell divisions.
- Disease Association: Alterations in histone modifications are linked to various diseases, including cancer, suggesting they could be potential therapeutic targets.
Histones represent a bridge between the genetic code and the functional outcomes in the cellular environment, illustrating their vital role in the understanding of genetics.
Molecular Weight of Histone
Definition and Importance
Molecular weight, in the context of proteins, refers to the mass of a given protein molecule expressed in Daltons (Da) or kilodaltons (kDa). Histone , as a core component of nucleosomes, typically exhibits a molecular weight around 15 kDa. This weight is fundamental to our understanding of its biochemical properties and interactions. The significance of molecular weight in histone lies not only in its structural role but also in the potential variations resulting from post-translational modifications such as methylation, acetylation, and phosphorylation. These modifications can alter the molecular weight and subsequently influence the behavior and function of during processes like gene expression and chromatin remodeling.
Standard Molecular Weight Value
The standard molecular weight of histone is approximately 15.3 kDa. This specific value is derived from its amino acid composition and sequence. The molecular weight is considered a critical parameter when conducting biochemical analyses, such as mass spectrometry. Proper identification and quantification of histone in various studies depend on knowing its standard molecular weight. Understanding this value helps researchers to evaluate comparative studies regarding histone variants and their respective roles in cellular pathways.
Variations in Molecular Weight
Variations in molecular weight for histone are a consequence of several factors. Post-translational modifications are the primary contributors to these variations. Common modifications include:
- Acetylation: Addition of an acetyl group that generally reduces the positive charge of histone and affects its interaction with DNA.
- Methylation: Involves adding methyl groups; some methylation patterns are associated with active or repressed gene states.
- Phosphorylation: Addition of phosphate groups plays a key role during cell division and stress response.
These modifications can alter the molecular weight significantly, thereby influencing chromatin structure and function. It is possible for the molecular weight to increase by several kilodaltons, depending on the number and type of modifications.
In summary, the molecular weight of histone is not a static metric but rather a dynamic aspect that reflects its role in cellular processes.
Structure of Histone
The structure of histone is crucial for its functionality and the overall role it plays in cellular processes such as gene regulation and chromatin formation. Understanding this structure helps elucidate how histone proteins interact with DNA. Histone forms part of the core histone octamer that wraps DNA into nucleosomes, thus compaction of the genetic material. This primary function highlights the importance of in not just structural capacity but also in genetic expression regulation.
Primary Structure Analysis
The primary structure of histone consists of a linear sequence of amino acids. This sequence is key to defining the protein's properties and how it interacts with other molecules. Histone has a high content of positive charges due to the presence of lysine and arginine residues. These positively charged amino acids facilitate binding to the negatively charged DNA, securing the nucleosome's structure.
The histone gene undergoes variations, particularly through post-translational modifications. The most notable are methylation, acetylation, and phosphorylation. These modifications can alter how tightly binds to DNA, thus affecting gene accessibility for transcription. In essence, studying the primary structure provides insights into the versatility and adaptability of histone in response to cellular signaling.
Secondary and Tertiary Structures
In terms of secondary structure, histone predominantly contains Ξ±-helices and a few Ξ²-strands. This helical structure allows the protein to fold into a compact form, which is critical for its association with DNA. The ability for histone to form these structures is facilitated by hydrogen bonding patterns among the peptide backbone.
The tertiary structure of histone involves further folding into a globular form. This three-dimensional shape enhances the interaction with other histones and non-histone proteins. The histone fold, a specific structure found in histones, presents a stable platform for forming interactions within the nucleosome core.
In summary, both secondary and tertiary structures are imperative in determining 's stability and functionality. Disruptions in these structures, whether through mutation or post-translational modifications, can have far-reaching impacts on cellular processes, including gene expression.
Understanding the structure of histone provides insights into its role not just as a structural protein, but as a regulator of gene expression and epigenetic modifications.
Understanding the structure of histone provides insights into its role not just as a structural protein, but as a regulator of gene expression and epigenetic modifications.
Role of Histone in Gene Regulation
Histone plays a critical role in the regulation of gene expression. It is an integral part of the histone octamer around which DNA is wrapped to form nucleosomes. This structure is fundamental to the higher-order organization of chromatin. The regulation of gene expression involves complex interactions between various proteins, modifications, and environmental factors. Histone is at the center of these processes. Its modifications can lead to changes in chromatin structure, influencing the accessibility of DNA for transcriptional machinery.
Mechanisms of Transcriptional Regulation
The mechanisms by which histone contributes to transcriptional regulation can be classified into several key aspects:
- Histone Modifications: Post-translational modifications of histone , such as methylation, acetylation, and phosphorylation, directly affect gene activation or repression. For example, trimethylation of lysine 4 on histone is associated with active transcription.
- Chromatin Remodeling: The presence of specific histone variants can influence the dynamics of chromatin remodeling. Histone variants can create distinct chromatin environments that affect how tightly the DNA is wrapped, which is crucial during transcription.
- Recruitment of Regulatory Proteins: Histone modifications serve as signals for the recruitment of transcription factors and co-regulators to the promoter regions of genes. This recruitment is essential for forming the transcriptional complex necessary for implementing gene expression.
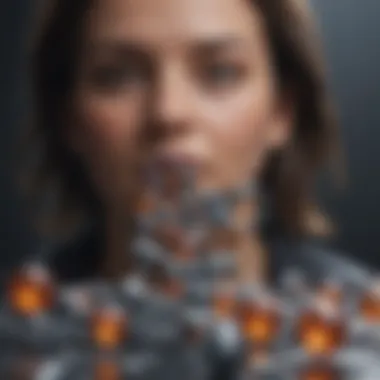
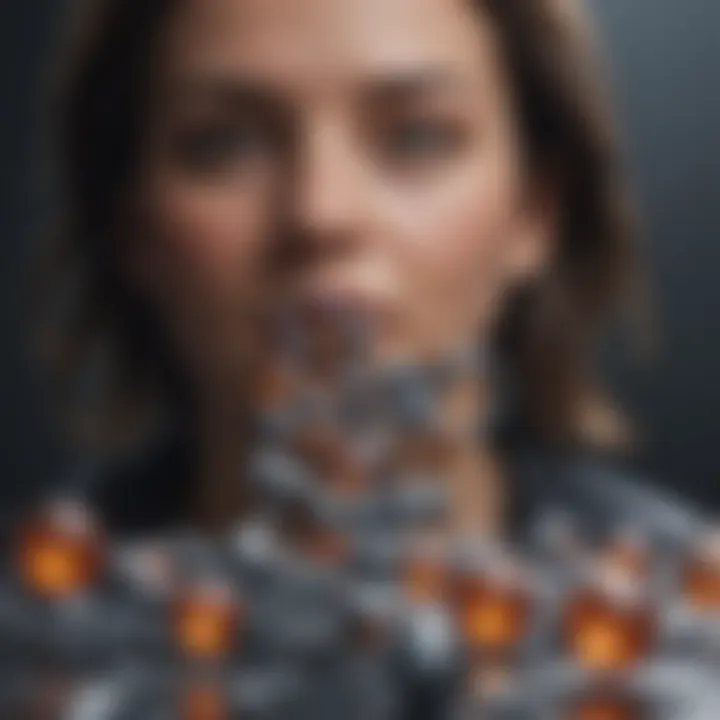
"Histone modifications act as a code that can either promote or inhibit transcription by attracting or repelling regulatory proteins."
"Histone modifications act as a code that can either promote or inhibit transcription by attracting or repelling regulatory proteins."
Influence of Molecular Weight on Function
The molecular weight of histone may have implications for its function and interactions. Variants or modifications can alter its molecular weight slightly, but these changes can affect its physical properties and the behavior of the chromatin.
- Protein Interactions: A slightly different molecular weight can influence how histone interacts with other proteins or DNA. Structural changes, even small ones, may alter binding affinities and impact regulatory networks.
- Compaction of Chromatin: The molecular weight can play a role in the degree of compaction of chromatin. Heavier histones may lead to tighter packing, which affects accessibility for transcription factors and RNA polymerase.
- Potential Biomarkers: Variations in the molecular weight of histone due to mutations can serve as biomarkers for certain diseases. Understanding these variations could aid in the identification of disease states and the development of targeted therapies.
Research on the role of histone in gene regulation highlights the importance of both its structure and its modifications. This understanding informs us about essential biological processes and potential therapeutic applications.
Epigenetics and Histone
Epigenetics is the study of heritable changes in gene expression that do not involve changes to the underlying DNA sequence. Histone proteins, including histone , play a crucial role in this process. The interplay between histones and DNA is fundamental to understanding gene regulation and the mechanisms of heredity. Histone is particularly significant due to its role in modifying gene expression through several mechanisms.
Histone Modifications
Histone modifications are chemical alterations to the histone proteins, which can change the structure of chromatin and influence gene accessibility. Common modifications include methylation, acetylation, phosphorylation, and ubiquitination of histone . Each of these modifications can either promote or inhibit gene expression depending on their nature and location.
- Methylation: Often associated with gene repression. Methyl groups attach to specific lysine residues on histone .
- Acetylation: Generally leads to gene activation. Acetyl groups are added to lysines, rendering the chromatin less condensed and more accessible for transcription.
- Phosphorylation: Typically involved in gene regulation during cell division.
- Ubiquitination: Can signal for either gene activation or repression, depending on the context.
These modifications serve as a regulatory mechanism that can be influenced by environmental factors. They can alter how tightly DNA is wrapped around histones, thus playing a direct role in making genes either more accessible for transcription or more compact and inactive.
Impact on Gene Expression
The impact of histone and its modifications on gene expression is profound. The various states that histone can assume affect not only the chromatin's structure but also its functionality. This creates a complex regulatory system that allows cells to respond rapidly to stimuli or stress by altering gene activity.
"Histone modifications provide a dynamic way for cells to regulate gene expression without altering the genetic code itself."
"Histone modifications provide a dynamic way for cells to regulate gene expression without altering the genetic code itself."
Epigenetic regulation through histones allows for cellular memory, creating distinctions in gene expression patterns among different cell types even though they share identical DNA. For instance, neuronal cells and muscle cells can express different sets of genes while containing the same genetic information. This specificity is key in development and the maintenance of tissue identity.
Additionally, dysregulation of histone modifications is linked to various diseases, including cancer. Understanding how histone modifications impact gene expression patterns could lead to novel therapeutic strategies.
In summary, the relationship between epigenetics and histone is critical. It influences gene expression through a range of modifications, shaping the functional landscape of the genome across different environments and conditions.
Experimental Techniques for Analyzing Histones
Experimental techniques used to analyze histones are crucial for comprehensive understanding of their molecular weight and functional roles. These methodologies contribute significantly to unraveling the complex dynamics of chromatin and gene regulation. They also allow researchers to examine how specific histone modifications may influence biological processes. This section will detail two important techniques: mass spectrometry and chromatin immunoprecipitation (ChIP).
Mass Spectrometry Applications
Mass spectrometry has emerged as a powerful tool for studying histone proteins, including histone . This technique allows for the precise measurement of molecular weights and the identification of various post-translational modifications, which are crucial for histone function. By analyzing histones, researchers can detect changes in protein structure, abundance, and modifications.
- Detection of Post-Translational Modifications
Mass spectrometry can identify modifications such as acetylation, methylation, and phosphorylation. These modifications have significant implications for gene expression and chromatin dynamics. - Tracking Protein Variants
The technique can differentiate between various isoforms of histone . Different isoforms may have distinct functional outcomes in various cellular contexts. - Quantitative Analysis
Mass spectrometry enables quantification of histone proteins in different samples. This is beneficial for comparing conditions, such as normal versus diseased states.
Mass spectrometry not only reveals the molecular weight of histone but also provides insights into its biological roles through detailed analysis of its chemical state. The precision and sensitivity of this technique enhance its application in histone research.
Chromatin Immunoprecipitation (ChIP)
Chromatin immunoprecipitation (ChIP) is another essential method for studying histones. It bridges the gap between protein biology and genomics, allowing for analysis of histone-DNA interactions under different cellular conditions.
- Understanding Histone Binding
ChIP identifies regions of the genome where specific histones bind. Such binding is crucial for regulating gene expression. - Characterizing Histone Modifications
By using antibodies specific to modified histones, ChIP can provide a detailed map of histone modifications across various genomic loci. This helps in understanding how different modifications affect gene transcription. - Integrating with Sequencing Techniques
When combined with sequencing technologies, ChIP can reveal where modification patterns occur across the entire genome. This high-throughput capability allows extensive profiling of histone modifications and helps identify regulatory elements in the genome.
The integration of mass spectrometry with chromatin immunoprecipitation techniques provides a comprehensive perspective on histone biology, enabling deeper insights into gene regulation.
The integration of mass spectrometry with chromatin immunoprecipitation techniques provides a comprehensive perspective on histone biology, enabling deeper insights into gene regulation.
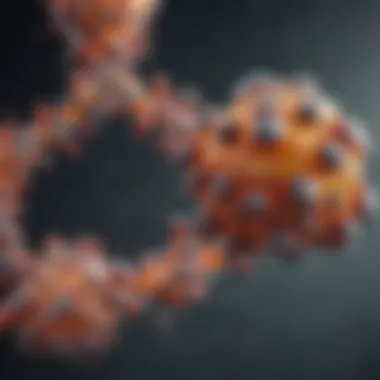
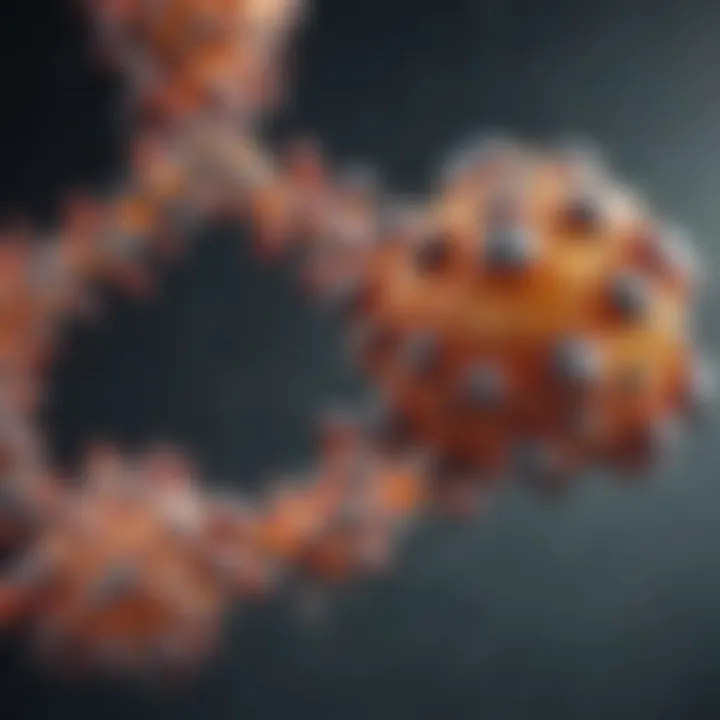
Clinical Relevance of Histone
The clinical relevance of histone is pivotal in understanding the mechanics of various diseases. Histone mutations can lead to altered gene expression profiles, causing significant health implications. This is not merely an academic concern but has real-world effects on disease manifestation and progression. Nearly every function in cells, from repair mechanisms to apoptosis, is influenced by the state of chromatin, and thus the integrity of histone proteins.
Histone Mutations and Disease
Histone mutations have been identified in several cancers. These mutations can lead to changes in chromatin structure, thereby modifying regulation of gene expression. For example, the mutation of histone at specific amino acid positions is observed in gliomas and certain leukemias. These alterations disrupt normal cellular processes and drive tumorigenesis.
- Common mutations:
- K27M in diffuse intrinsic pontine glioma (DIPG)
- G34R, often seen in pediatric glioma cases
Mutated histones can act as drivers of oncogenesis by creating a permissive chromatin environment for oncogenes, while simultaneously silencing tumor suppressor genes. Thus, analyzing these mutations not only improves our understanding of tumor biology but also presents avenues for targeted therapies.
Therapeutic Targets
Histone represents a potential therapeutic target in cancer treatment and other diseases characterized by epigenetic changes. Targeted therapies can involve small molecules aimed at correcting or inhibiting the effects of histone mutations. The identification of altered histone marks offers a foundation for creating treatments that could restore normal gene expression.
- Current approaches in development include:
- Histone deacetylase inhibitors (HDACi):
These compounds aim to reverse abnormal epigenetic signatures by inhibiting deacetylases, thus reactivating silenced genes. - Targeting specific mutations:
Research is uncovering specificity in targeting particular histone mutations. For instance, drugs that specifically target the K27M mutation can potentially change the course of treatment for patients with DIPG. - Chimeric antigen receptor (CAR) T-cell therapy:
This therapy can be tailored to target tumor-associated antigens that result from histone modifications.
"Understanding histone modifications and mutations is integral to advancing personalized medicine approaches, particularly in oncology."
"Understanding histone modifications and mutations is integral to advancing personalized medicine approaches, particularly in oncology."
In summary, the clinical relevance of histone extends beyond basic research. It directly connects to real clinical outcomes in cancer and other diseases, emphasizing the importance of continued exploration in this field.
Future Directions in Histone Research
The field of histone research is constantly evolving, giving rise to new insights and discoveries. This section highlights the importance of future directions in histone research and outlines specific areas warranting further investigation. Understanding molecular weight variations, epigenetic modifications, and the intricate roles histones play in diseases underpins the need for innovative research practices. The advances in this field can potentially propel our understanding of genetics into new realms.
Emerging Technologies
With advancements in technology, new methodologies are being introduced to study histones more effectively. Next-generation sequencing plays a significant role in this context, allowing researchers to analyze histone modifications and their implications on gene expression with unprecedented precision. Additional single-cell sequencing initiatives offer deeper insights into the heterogeneity of histone modifications across different cell types.
Imaging technologies, such as super-resolution microscopy, are also emerging tools. They enable scientists to visualize histone behavior in live cells, providing real-time data on chromatin dynamics. Moreover, mass spectrometry continues to be pivotal in profiling histone modifications at a proteomic scale, revealing complex interactions within chromatin.
"Innovative technologies are not just advancing our understanding of histones, but are also reshaping the entire approach to genomics and epigenetics."
"Innovative technologies are not just advancing our understanding of histones, but are also reshaping the entire approach to genomics and epigenetics."
Potential for Personalized Medicine
The advances in histone research point toward significant implications for personalized medicine. Histone modifications are not merely biochemical markers; they can influence an individualβs susceptibility to diseases, including cancer and neurodegenerative disorders. By understanding the specific modifications on histones, tailored therapeutic approaches can be developed.
For instance, the study of histone variants and their unique properties may lead to the identification of biomarkers for certain diseases. This knowledge could enable the design of targeted treatments that consider a patient's unique epigenetic landscape.
Furthermore, understanding how histone modifications react to environmental factors can pave the way for preventive strategies in personalized medicine. Researching the links between lifestyle choices and epigenetic changes is essential for developing comprehensive health strategies that incorporate both genetic predispositions and environmental influences.
In summary, the future of histone research is multifaceted, with potential benefits reaching far beyond the lab. The integration of emerging technologies and personalized medicine approaches signifies a transformative achievement in molecular biology, ultimately enhancing our understanding of human health and disease.
The End
In the landscape of molecular biology, histone stands out as a significant player. The discussion surrounding its molecular weight is crucial for understanding its function in chromatin structure and gene regulation. This article has emphasized several key aspects related to histone that deserve particular attention.
Summary of Key Points
- Molecular Weight Definition: Histone has a standard molecular weight of approximately 15.2 kilodaltons, which influences its interactions within histone octamers.
- Variability: There are variations in the molecular weight of histone due to post-translational modifications. Such modifications can include methylation, acetylation, and phosphorylation. Each alteration can alter the structural and functional capabilities of the protein, impacting gene expression.
- Functional Implications: The molecular weight of histone affects not only gene regulation but also may play a role in biological processes, such as DNA repair and replication.
- Research Techniques: Various experimental techniques, including mass spectrometry, are pivotal in analyzing histone modifications and understanding the changes in molecular weight, further emphasizing the need for precision in the study of histones.
Implications for Future Study
Further research on histone , focusing on its molecular weight and modifications, can unveil more about its role in genetic regulation and epigenetics. Here are a few promising directions for future inquiry:
- Role in Disease: Investigating how alterations in histone molecular weight contribute to diseases, such as cancer, will be critical in understanding pathological mechanisms.
- Therapeutic Strategies: Developing therapies that target specific histone modifications could revolutionize treatments for various genetic disorders.
- Advanced Techniques: Embracing new technologies in proteomics may yield deeper insights into histone functions, leading to better management of epigenetic conditions.