A Comprehensive Guide to Protein Synthesis
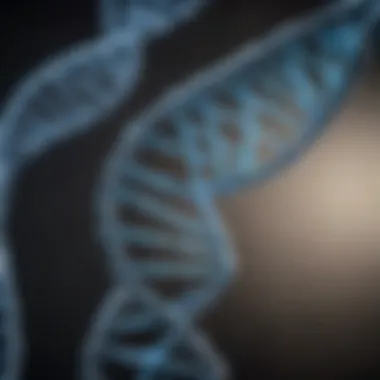
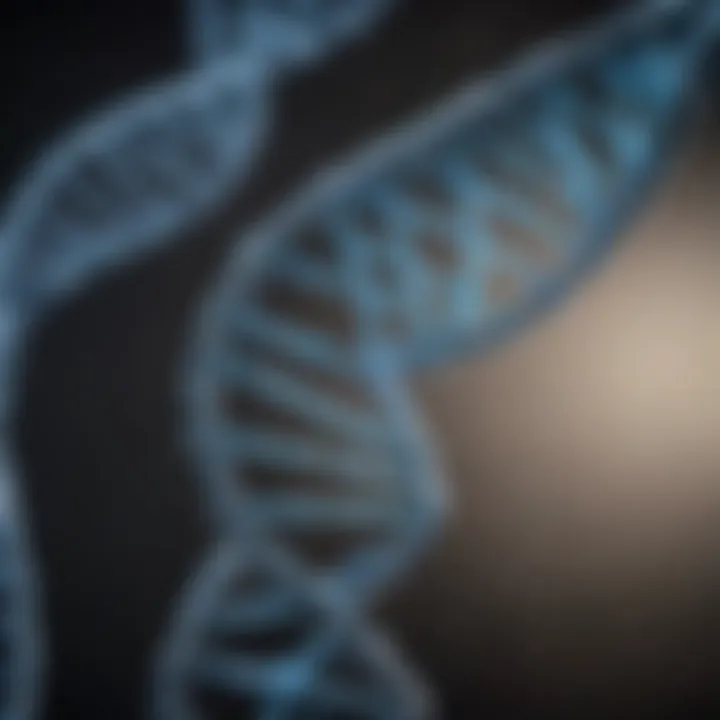
Intro
Protein synthesis is a crucial biological process fundamental to life. It involves a series of steps that translate genetic information into functional proteins. Understanding these steps is essential for students, researchers, educators, and professionals alike. This guide aims to clarify the intricacies of protein synthesis, from the initial transcription of DNA to the final modifications that lead to fully functional proteins.
Key Concepts
Definition of the Main Idea
Protein synthesis refers to the process in which cells generate proteins. Proteins serve a multitude of functions, including acting as enzymes, structural components, and signaling molecules. Each proteinβs structure and function are determined by the sequence of amino acids it contains, which is dictated by the genetic blueprint found in DNA.
Overview of Scientific Principles
The process of protein synthesis consists of three main stages: transcription, translation, and post-translational modification.
- Transcription: This is the initial step where a specific segment of DNA is copied into RNA. The enzyme RNA polymerase plays a critical role in synthesizing messenger RNA (mRNA) from a DNA template.
- Translation: In this stage, read the information on the mRNA to construct a protein. Ribosomes, along with transfer RNA (tRNA), are crucial for this process as they decode the mRNA's sequence into a corresponding sequence of amino acids.
- Post-Translational Modification: After translation, newly formed proteins may undergo modifications that are essential for their final functional forms. These modifications can include phosphorylation, glycosylation, and cleavage.
Each of these phases is tightly regulated and essential for ensuring that proteins are synthesized correctly. Protein synthesis is not just a cellular function; it is a foundational aspect of life.
"Understanding protein synthesis is essential for advances in biology and medicine, affecting areas such as gene therapy and biotechnology."
"Understanding protein synthesis is essential for advances in biology and medicine, affecting areas such as gene therapy and biotechnology."
Current Research Trends
Recent Studies and Findings
Research in protein synthesis is rapidly evolving, with many studies focusing on the regulation of these processes. For instance, studies have identified various factors that influence translation efficiency and accuracy. Another important area of research is understanding how ribosomes recognize different mRNA sequences, which can affect protein production rates.
Significant Breakthroughs in the Field
Recent breakthroughs include advancements in techniques like CRISPR and RNA sequencing, enabling scientists to manipulate and observe protein synthesis at unprecedented levels of detail. These technologies have opened new avenues for research in genetic expression and regulation, which may pave the way for novel therapeutic strategies.
Prolusion to Protein Synthesis
Protein synthesis is a fundamental biological process, essential for the growth and maintenance of cellular structures and functions. Understanding this process provides insights into how cells respond to their environment, adapt to changes, and ultimately survive. In this article, we will dissect the intricate steps that comprise protein synthesis, covering a wide range of topics from transcription to post-translational modification.
The creation of proteins in cells is not just a genetic exercise; it integrates various molecular players, including DNA, RNA, and ribosomes, each fulfilling a distinct role in the synthesis chain. Moreover, protein function is vital for all cellular actions, whether they involve metabolic reactions, structural support, or signaling pathways.
This exploration into protein synthesis will benefit students, researchers, and professionals, highlighting its significance in biological mechanisms and potential applications in health and disease.
Defining Protein Synthesis
Protein synthesis refers to the multi-step process by which cells construct proteins based on the information encoded in their genes. This process primarily involves two key phases: transcription and translation. During transcription, the DNA sequence of a gene is copied into messenger RNA (mRNA). This mRNA then serves as a template for building a protein during translation, where ribosomes read the mRNA to assemble amino acids into a functional protein chain.
The enzymatic machinery in the cell facilitates these phases, ensuring that proteins are synthesized accurately and efficiently. Proteins, the end products of this synthesis, play crucial roles in virtually every cellular process. Thus, understanding protein synthesis is fundamental in various fields, including molecular biology, genetics, and biotechnology.
Importance in Cellular Function
The process of protein synthesis is paramount for maintaining cellular function and homeostasis. Proteins perform a vast array of functions, including enzyme catalysis, cellular signaling, and structural support. For instance, enzymes are proteins that accelerate biochemical reactions, making them vital for metabolic processes.
Additionally, proteins are integral to signal transduction pathways, helping cells respond to environmental changes. Without proteins, cells would be unable to perform their fundamental functions. Furthermore, abnormalities in protein synthesis can lead to a variety of diseases, including cancer and genetic disorders.
Understanding how proteins are synthesized sheds light on potential therapeutic targets and strategies to address issues stemming from protein misfolding or dysfunction. Therefore, a comprehensive grasp of protein synthesis is essential for advances in medical research and treatment options.
Overview of Genetic Information
In understanding protein synthesis, the overview of genetic information provides foundational knowledge about how genetic material guides the cellular machinery that produces proteins. This section sheds light on two key components: the role of DNA and the structure of genes. Each contributes significantly to our grasp of the broader biological processes involved in protein synthesis.
Role of DNA
DNA, or deoxyribonucleic acid, serves as the master template for all genetic information in living organisms. It not only encodes the instructions necessary for the production of proteins but also governs hereditary traits and cellular functions. The double-helix structure of DNA allows it to store vast amounts of information in a compact form.
The significance of DNA in protein synthesis stems from its function during transcription. In this phase, specific sequences of DNA are transcribed into messenger RNA (mRNA). This conversion is crucial because mRNA, rather than DNA, plays a direct role in ribosomes during translation. Furthermore, the integrity of DNA is vital for maintaining the accuracy of protein production. Any alterations, or mutations, within the DNA sequence can lead to the synthesis of dysfunctional proteins, potentially resulting in various diseases. Understanding these dynamics is essential for recognizing how genetic information directly influences protein synthesis.
Structure of Genes
Genes are defined as specific sequences of nucleotides that code for proteins. Each gene contains a particular sequence that defines the order of amino acids in a protein. The basic unit of a gene includes promoter regions, exons, and introns. Promoters are vital for initiating transcription, as they signal the start point for RNA polymerase to bind and read the DNA sequence.
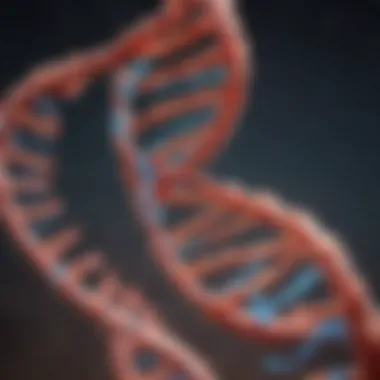
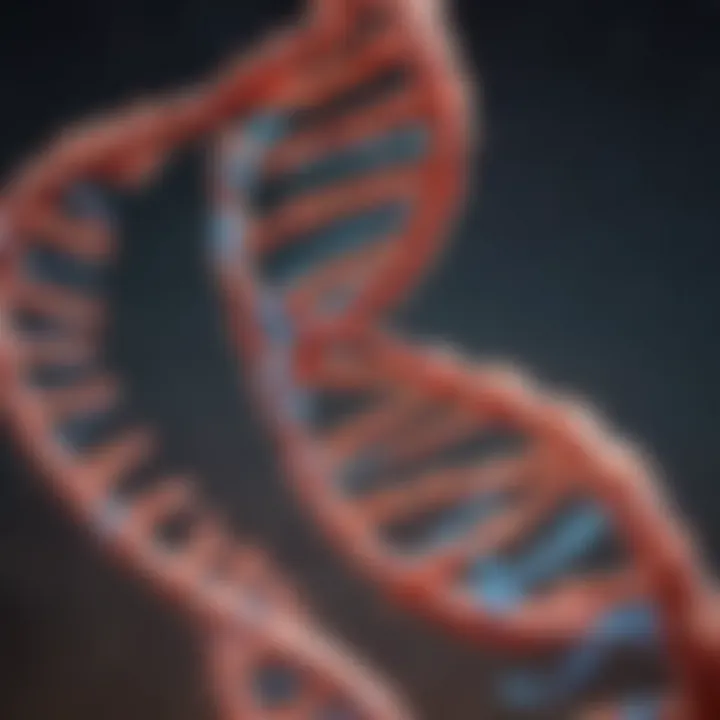
Exons are the coding regions that are present in the final mRNA transcript, while introns are non-coding sequences that are spliced out during RNA processing. The structure of genes is crucial for determining how and when a protein is synthesized. By facilitating various regulatory mechanisms, the arrangement of genetic information allows cells to respond to internal and external signals appropriately. This responsiveness is essential for maintaining cellular homeostasis and adapting to changing environments.
In summarizing, the overview of genetic information not only frames the necessity of DNA and the structural aspects of genes but also highlights their interconnectedness with the fundamental processes of protein synthesis. A profound comprehension of these elements enables a better understanding of cellular biology and genetic engineering, with implications that reach far beyond basic science.
Transcription: The Initial Phase
Transcription is a critical process in protein synthesis, acting as the bridge between DNA and RNA. It is during this phase that the genetic information stored in DNA is copied to messenger RNA (mRNA), which carries the code required for protein production. Understanding transcription is essential not only for grasping how proteins are synthesized but also for comprehending various cellular functions and mechanisms.
Initiation of Transcription
The initiation phase of transcription begins when RNA polymerase, the enzyme responsible for synthesizing RNA, binds to a specific region of DNA called the promoter. This step is crucial as it marks the starting point for transcription. The promoter contains specific sequences that signal the RNA polymerase to attach to the DNA. Once bound, the DNA strands unwind, creating a transcription bubble.
It is important to note that various transcription factors assist RNA polymerase in recognizing promoters. These proteins facilitate the assembly of the transcription machinery. Without these factors, the process may not initiate properly, leading to ineffective gene expression. The initiation stage is, therefore, a finely tuned mechanism that ensures transcription occurs at the right time and under accurate conditions.
Elongation of RNA Strand
After initiation, elongation commences. During this phase, RNA polymerase moves along the DNA template strand, adding RNA nucleotides complementary to the DNA sequence. This action builds the RNA strand in a 5' to 3' direction. Unlike DNA replication, where both strands are synthesized, only one strand of DNA serves as the template during transcription.
The RNA polymerase acts relatively quickly, synthesizing the RNA strand in real-time. This phase continues until the RNA polymerase reaches a termination signal. Elongation is vital for producing a complete RNA strand, which will ultimately be processed into mRNA.
Termination of Transcription
Termination marks the end of transcription. When RNA polymerase encounters specific sequences in the DNA known as terminators, the synthesis of RNA halts. At this point, the RNA polymerase releases the newly synthesized RNA molecule, which is initially in the form of pre-mRNA.
Following termination, the RNA strand undergoes additional processing before it can be translated into a protein. The termination process is crucial as it ensures the accuracy of the mRNA produced, which affects subsequent steps in protein synthesis.
"Transcription is the first step in the journey from gene to protein, pivotal for understanding cellular functions."
"Transcription is the first step in the journey from gene to protein, pivotal for understanding cellular functions."
In summary, transcription involves initiation, elongation, and termination phases. Each phase holds significance in determining the accuracy and effectiveness of gene expression and protein synthesis. Gaining a deeper understanding of this initial phase of protein synthesis equips one with the foundational knowledge necessary for exploring subsequent processes.
RNA Processing: Modifications After Transcription
RNA processing is a critical phase in the lifecycle of messenger RNA (mRNA). After transcription, the precursor mRNA, or pre-mRNA, undergoes a series of modifications. These modifications transform the immature RNA transcript into a functional mRNA that can be translated into protein. Understanding this step is crucial as it prepares the RNA for its ultimate role in protein synthesis.
The main types of RNA processing include capping, polyadenylation, and splicing. Each modification has specific functions and plays an essential role in how genes are expressed and ultimately how proteins are synthesized.
Capping of RNA
Capping refers to the addition of a special structure at the 5' end of the pre-mRNA. This cap, often known as the 5' cap, is a modified guanine nucleotide. The cap serves several important functions. It protects the mRNA from degradation, aids in the transportation of mRNA out of the nucleus, and is critical for the initiation of translation. Without a proper cap, the stability and efficiency of mRNA are significantly reduced.
The following are key points regarding capping:
- Protection: The cap shields the mRNA from enzymes that could otherwise degrade it.
- Translation Initiation: The ribosomes recognize the cap to begin translation.
- Splicing Enhancement: The presence of the cap can facilitate splicing splicing of the pre-mRNA.
"The 5' cap is vital for mRNA stability and translation efficiency."
"The 5' cap is vital for mRNA stability and translation efficiency."
Polyadenylation
Polyadenylation is the addition of a poly(A) tail at the 3' end of the pre-mRNA. This tail consists of a series of adenine nucleotides and plays multiple crucial roles. Firstly, it aids in stabilizing mRNA and protects it from degradation by nucleases. Secondly, the poly(A) tail is essential for the export of the mRNA from the nucleus to the cytoplasm. Lastly, it influences the efficiency of translation. Various lengths of poly(A) tails can regulate mRNA stability and translation levels.
Important aspects of polyadenylation include:
- Stability: The poly(A) tail offers protection from enzymatic degradation.
- Nuclear Export: It helps in the transport of the mRNA into the cytoplasm.
- Regulation: The length of the tail can affect how long the mRNA lasts and how much protein is produced.
Splicing of Introns and Exons
Splicing is an essential process that removes introns, the non-coding regions of the pre-mRNA, and joins exons, the coding sequences. This process is carried out by a complex of proteins and RNA called the spliceosome. Correct splicing is vital; any errors can lead to the production of nonfunctional proteins or diseases.
Splicing serves the following purposes:
- Gene Regulation: It can produce multiple mRNA variants from a single gene, allowing for different protein types.
- Efficiency: Removing introns increases the efficiency of translation by streamlining the mRNA.
- Mature mRNA Formation: Ensures that only coding regions are present in the final mRNA, ready for translation.
Translation: The Synthesis of Proteins
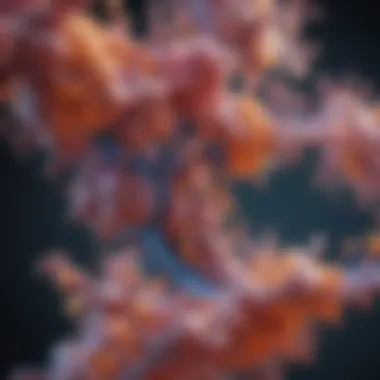
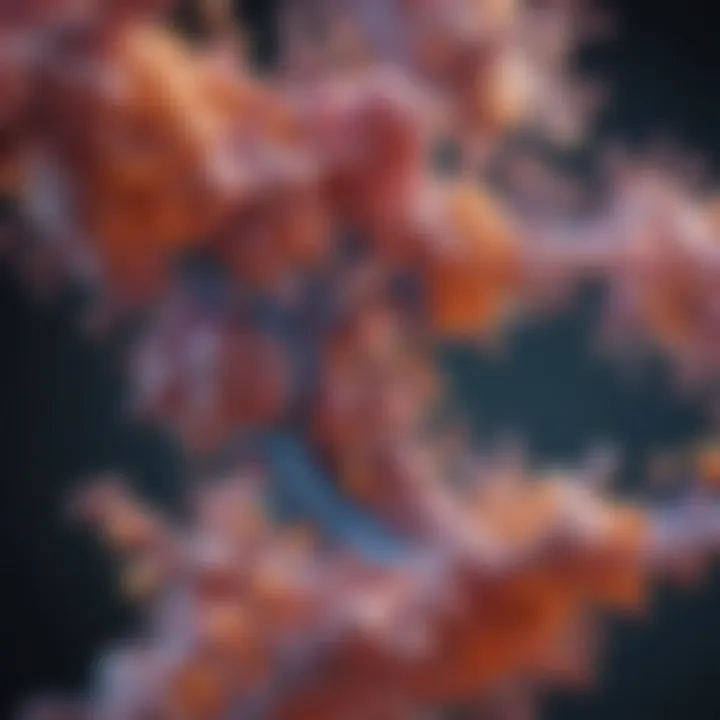
Translation is a critical process in the overall mechanism of protein synthesis. It transposes the genetic code from mRNA into a sequence of amino acids, forming proteins that are essential for various cellular functions. Understanding translation helps clarify how cells produce the diverse array of proteins needed for structure and function. It also emphasizes the importance of accuracy and regulation during protein synthesis, as errors can lead to dysfunctional proteins, contributing to diseases.
Initiation of Translation
The initiation of translation marks the beginning of protein synthesis. It starts with the assembly of the ribosome, a molecular machine composed of ribosomal RNA and proteins. This assembly involves the small ribosomal subunit binding to the mRNA molecule at the start codon, typically AUG. The presence of initiation factors is critical here; they assist in the recruitment of transfer RNA (tRNA) carrying the first amino acid, methionine.
Once the ribosomal subunits and the tRNA have correctly assembled around the start codon, this sets the stage for the elongation phase. The role of initiation in translation is paramount as it determines the fidelity and efficiency of protein synthesis.
Elongation in Translation
During elongation, the ribosome moves along the mRNA strand, adding amino acids one by one to the growing polypeptide chain. Each amino acid is brought to the ribosome by a specific tRNA, which recognizes the corresponding codon on the mRNA. The ribosome facilitates the formation of peptide bonds between adjacent amino acids, effectively linking them together into a polypeptide.
Key Steps In Elongation:
- Codon Recognition: The tRNA's anticodon pairs with the mRNA's codon in the A site of the ribosome.
- Peptide Bond Formation: The ribosome catalyzes the peptide bond formation between the existing amino acid chain and the new amino acid.
- Translocation: The ribosome shifts to the next codon, moving the tRNA from the A site to the P site.
This process continues until a stop codon is reached, clearly demonstrating the importance of elongation in determining protein sequence and structure.
Termination of Translation
Termination occurs when a stop codon is encountered in the mRNA. Stop codons do not correspond to any amino acids; instead, they signal the end of the translation process. Release factors play an essential role here, binding to the ribosome and facilitating the disassembly of the ribosomal complex. This interaction enables the release of the newly synthesized polypeptide chain, completing the synthesis process.
Aftermath of Termination:
- The ribosomal subunits separate, allowing the mRNA and tRNA to be released and recycled for future rounds of translation.
- The polypeptide may undergo several modifications post-translation, setting the stage for its eventual function within the cell.
In summary, the translation process comprehensively underscores the sophistication of cellular machinery and the need for precise mechanisms in protein production.
Role of Ribosomes in Translation
Ribosomes play a crucial role in the translation phase of protein synthesis. Acting as the site where messenger RNA (mRNA) is translated into a polypeptide chain, these complex molecular machines are vital to understanding how proteins are made in cells. Their importance lies not only in the synthesis process but also in their ability to ensure the accuracy and efficiency of protein production. The structure and functionality of ribosomes greatly influence the successful assembly of proteins, which are essential for cellular function.
Structure of Ribosomes
Ribosomes are made up of ribosomal RNA (rRNA) and proteins. They exist in two subunits: the large subunit and the small subunit. The sizes of these subunits can vary between different organisms, but the basic structure remains conserved. This bipartite system enables ribosomes to interact with mRNA and transfer RNA (tRNA) during the translation process.
- Large Subunit: The large subunit catalyzes peptide bond formation between amino acids, helping to synthesize the growing polypeptide chain. It contains the active site for peptidyl transferase, an enzymatic function critical for protein synthesis.
- Small Subunit: The small subunit's primary role is to read the mRNA sequence. It ensures the correct alignment of tRNA anticodons with the mRNA codons, maintaining fidelity in translation.
This structural specificity allows ribosomes to bind mRNA and tRNA effectively, coordinating the assembly of amino acids in the correct sequence dictated by the genetic code.
Functionality of Ribosomal Sites
Ribosomes have three key sites that facilitate protein synthesis:
- A Site (Aminoacyl Site): This site holds the incoming tRNA, which carries an amino acid. The tRNA anticodon pairs with the corresponding mRNA codon here.
- P Site (Peptidyl Site): The P site holds the tRNA that is bound to the growing polypeptide chain. Peptide bonds form between the amino acids in the P and A sites during this stage.
- E Site (Exit Site): After the amino acid is added to the peptide chain, the tRNA moves to the E site before exiting the ribosome.
This coordinated action within the ribosome ensures that peptide synthesis occurs smoothly and efficiently. Proper functioning of ribosomal sites not only determines the speed of translation but also the overall accuracy of protein synthesis. Ribosomes essentially act as the machinery that translates genetic information into functional proteins, highlighting their indisputable significance in cellular processes.
"Ribosomes are not just passive players. They are dynamic machines intricately designed to read and translate genetic code into proteins needed for life."
"Ribosomes are not just passive players. They are dynamic machines intricately designed to read and translate genetic code into proteins needed for life."
Ribosomes represent a critical link in the protein synthesis chain, showcasing the elegance of cellular machinery in transforming genetic information into actionable proteins. Their structure and functionality exemplify the complexity of life at the molecular level.
Post-Translational Modifications
Post-translational modifications (PTMs) are crucial events that take place after protein synthesis has occurred. These modifications enhance the functionality, stability, and overall profile of a protein. While translation forms the primary structure of a protein, PTMs can significantly alter its physical and chemical properties. This alteration is essential for few reasons. First, PTMs enable proteins to gain complex structures that are necessary for their biological activity. Also, they provide means for regulating protein function in response to environmental changes.
Phosphorylation, glycosylation, ubiquitination, and methylation are examples of common types of PTMs. Understanding these modifications is vital for revealing how proteins interact within cells and how their functions are modulated. For students, researchers and professionals, the study of post-translational modifications offers insights into cellular mechanisms and potential targets for therapeutic intervention.
"Post-translational modifications are not just modifications; they are critical regulators of cellular function and homeostasis."
"Post-translational modifications are not just modifications; they are critical regulators of cellular function and homeostasis."
Types of Modifications
a. Phosphorylation: This process adds a phosphate group to a protein, commonly altering its activity and signaling pathways. It is mediated by enzymes such as kinases and phosphatases.
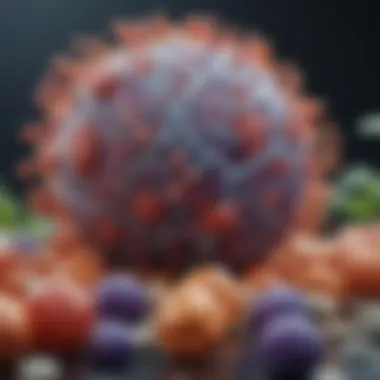
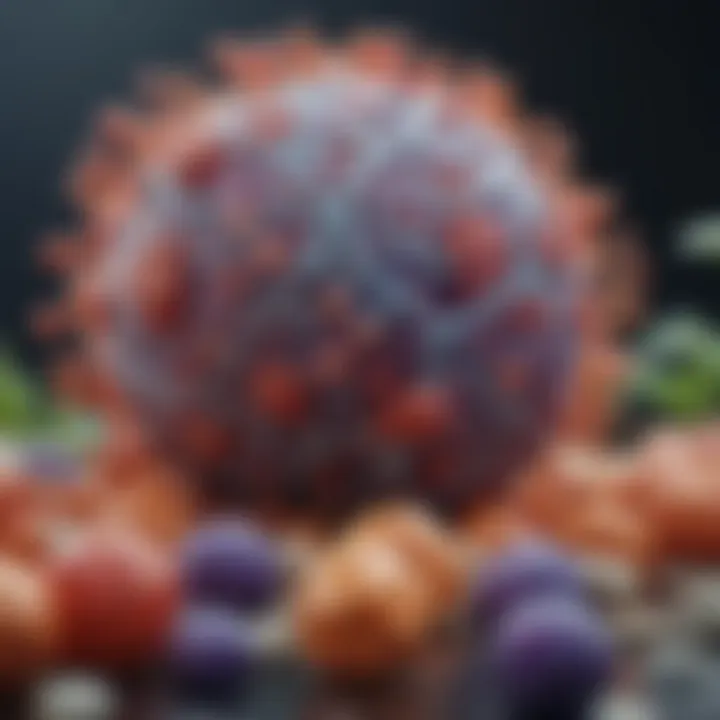
b. Glycosylation: Glycosylation involves the attachment of sugar moieties to proteins. This modification is important for protein folding, stability, and cell signaling. It happens mainly in the endoplasmic reticulum and Golgi apparatus.
c. Ubiquitination: This modification marks proteins for degradation, thus regulating protein levels in cells. Ubiquitin, a small protein, is attached to a target protein and directs it to proteasomes.
d. Methylation: Methylation adds a methyl group to proteins, influencing their function and interactions. It often plays a role in gene expression regulation.
Purpose of Modifications
The purpose of post-translational modifications centers around several critical functions:
- Regulation of Activity: Many proteins require PTMs to change conformation and become active or inactive. For example, phosphorylation can either enhance or inhibit the activity of enzymes, allowing for precise control of biochemical pathways.
- Protein Stability: Modifications help extend the lifespan of proteins in the cellular environment. Glycosylation, for instance, can protect proteins from proteolytic degradation.
- Cellular Localization: Certain PTMs serve as signals that guide a protein to its appropriate location in the cell. This targeting is vital for proper function, especially in multi-compartmentalized cells like eukaryotes.
- Protein-Protein Interactions: PTMs also facilitate interactions between proteins. For example, phosphorylated proteins can have specific affinity for other proteins, impacting signaling cascades.
In summary, post-translational modifications play an indispensable role in ensuring that proteins are not only synthesized accurately but also functionally proficient and responsive to cellular needs.
The Role of Chaperones in Protein Folding
The process of protein folding is crucial in determining the functionality of the protein. Molecular chaperones play an essential role in ensuring that proteins fold into their correct three-dimensional structures. Without these chaperones, proteins can misfold and lead to various diseases, including neurodegenerative conditions such as Alzheimer's and Parkinson's.
Chaperones assist in protein folding by providing a favorable environment for nascent polypeptides to reach their functional conformations. They bind to unfolded or misfolded proteins, preventing aggregation and providing pathways for proper assembly. Their involvement is a vital step that cannot be overlooked in the protein synthesis pathway.
"Chaperones are critical for the maintenance of cellular proteostasis, ensuring that proteins perform their designated roles efficiently."
"Chaperones are critical for the maintenance of cellular proteostasis, ensuring that proteins perform their designated roles efficiently."
Function of Molecular Chaperones
Molecular chaperones, such as heat shock proteins, assist in the folding process by preventing incorrect interactions that can lead to aggregation. These chaperones work mainly in two stages: the initial binding stage and the subsequent folding stage. In the initial stage, they recognize partially folded or unfolded proteins using specific binding sites. Once they bind, chaperones facilitate the folding process by undergoing conformational changes themselves, thus stabilizing the substrate protein.
Some commonly known molecular chaperones include Hsp70 and Hsp60. Hsp70 binds to nascent polypeptides and stabilizes them during translation, while Hsp60 forms large complexes that allow proteins to fold properly in an isolated environment. Their functions highlight the dynamic nature of protein folding and the importance of assistance in achieving correct structures.
Importance in Protein Functionality
The correctness of protein folding directly influences its functionality. Misfolded proteins can lose their biological activity, leading to malfunctioning pathways, signaling issues, or even cell death. This is particularly important in enzymes, where proper folding is essential for the enzyme's active site configuration.
Chaperones also play a protective role when cells undergo stress; they help to refold denatured proteins. In stressful conditions like heat shock or oxidative stress, the load on the cellular machinery increases. Chaperones mitigate this impact by refolding damaged proteins or directing them towards degradation pathways if they cannot regain their functional forms.
Understanding the role and mechanism of chaperones is critical for the development of therapeutic strategies aimed at misfolding diseases. For instance, enhancing chaperone activity can be a potential approach to counteract protein misfolding in various diseases.
Protein Targeting and Trafficking
Protein targeting and trafficking are crucial aspects of cellular biology. They ensure that proteins reach their correct destinations within the cell where they can perform their specific functions. This section emphasizes the mechanisms, importance, and implications of proper protein sorting, highlighting the rigorous processes involved.
Mechanisms of Targeting
The targeting of proteins to their appropriate cellular locations involves several well-coordinated mechanisms. These can include signal peptides, which are short sequences of amino acids that direct the protein to a particular site. For instance, proteins destined for the nucleus typically have nuclear localization signals. Other mechanisms involve the use of vesicles, that transport proteins towards their intended organelles.
In the endoplasmic reticulum (ER), transmembrane proteins enter through protein translocators. In contrast, proteins meant for secretion are often packaged into transport vesicles that bud from the ER and fuse with the Golgi apparatus. The Golgi plays a key role in modifying and sorting proteins before they are dispatched to their final destinations.
Additionally, post-translational modifications can also aid in tagging proteins for particular cellular locales. They help in recognizing where the protein is needed, which is essential in multicellular organisms where the protein's role varies significantly depending on cell type and function.
Significance in Cellular Function
The significance of protein targeting and trafficking cannot be overstated. It fundamentally contributes to maintaining cellular homeostasis and function. When proteins reach their designated sites, they can interact with other cellular components effectively, which is essential for various biological processes such as signaling, metabolism, and structural integrity.
Disruptions in targeting mechanisms can lead to severe cellular dysfunctions and contribute to the onset of various diseases, including cancer and neurodegenerative disorders. > "Accurate protein sorting is essential for cellular identity and function. Any misdirection can trigger pathological conditions."
Furthermore, the process impacts overall cellular health by ensuring that each organelle receives the appropriate enzymes or structural proteins necessary for its activities. Understanding these mechanisms allows researchers to devise therapeutic strategies that can correct mislocalized proteins, an area of increasing relevance in the field of medical research.
The End: Implications of Protein Synthesis
The process of protein synthesis is central to understanding cellular health and function. It is not simply a mechanism of producing proteins, but rather a critical pathway that influences various biological processes. Employees, students, and researchers can benefit by studying this because proteins are fundamental to life. They are involved in catalyzing reactions, providing structural support, and regulating cell signaling, among other roles.
Protein Synthesis and Cellular Health
The relationship between protein synthesis and cellular health cannot be overstated. Proteins are made up of amino acids, which are the building blocks of life. Disorders in protein synthesis can lead to various diseases. For example, genetic mutations can affect the transcription or translation of proteins, resulting in malfunctioning proteins. This can contribute to conditions like cystic fibrosis or muscular dystrophy.
Furthermore, robust protein synthesis is essential for tissue repair and growth after injury. A deficiency in adequate protein synthesis can hinder recovery and impact overall health. This highlights the need for a well-regulated synthesis process to maintain cellular integrity.
Future Perspectives in Protein Research
Looking forward, the exploration of protein synthesis remains a rich field for research and innovation. Advances in molecular biology techniques provide new methods to investigate how proteins are synthesized, modified, and function within the cell. One area of interest is the role of ribosomes and the efficiency of different ribosomal sites in translation. By understanding these mechanisms better, we can develop targeted therapies for diseases caused by protein malfunctions.
In addition, biotechnological applications have emerged, such as synthetic biology, where researchers manipulate genetic information to design proteins that do not exist in nature. These developments may lead to the creation of novel therapeutic proteins, enhancing treatment options for various health conditions.