Understanding RTPCR Techniques in Molecular Biology
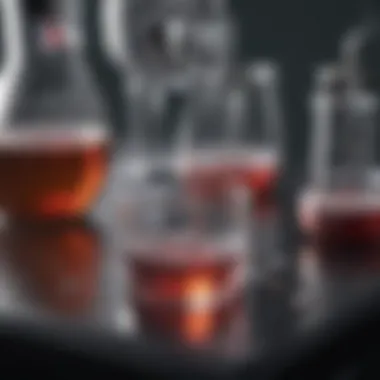
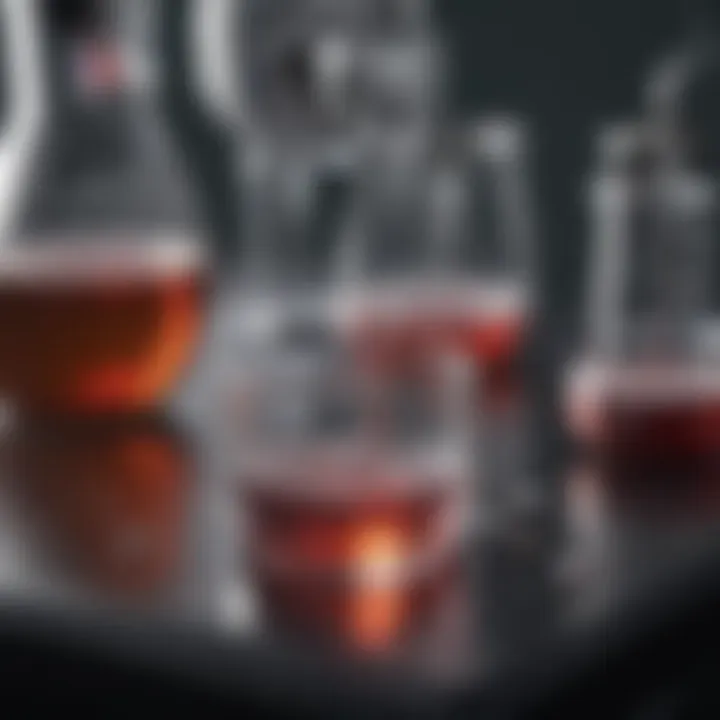
Intro
In molecular biology, the Reverse Transcription Polymerase Chain Reaction (RTPCR) has reshaped the landscape of diagnostics and research. This technique, which allows for the amplification of RNA, plays a critical role in various fields, including virology, genetics, and disease detection. As a cornerstone of lab practices today, understanding RTPCR not only enhances one's grasp of molecular mechanisms but also sheds light on its broad implications in our understanding of health and disease.
RTPCR assays have gained traction particularly with their capability to detect RNA viruses, something that has proven essential in response to global health challenges. This article will guide you through the core concepts surrounding RTPCR, delve into current research trends, and highlight the remarkable strides being made in this area. Prepare to see how RTPCR serves as a bridge between laboratory research and real-world applications in diagnostics.
Prologue to RTPCR
The introduction of Reverse Transcription Polymerase Chain Reaction (RTPCR) is not just a preamble but a vital framework for the entire discussion on its significance in molecular biology. Understanding RTPCR means addressing its intricate role in diagnostics and research, particularly its ability to detect RNA. In an era where rapid testing methods are more essential than ever, unveiling the nuances of RTPCR aids students, researchers, educators, and professionals in grasping how this technique fits within the broader scientific landscape.
Definition and Significance
RTPCR can be broken down into its two main components: reverse transcription and polymerase chain reaction. Simply put, it is a technique that transforms RNA into complementary DNA (cDNA) through the action of the enzyme reverse transcriptase, followed by amplification of that cDNA using the polymerase chain reaction. This transformation and amplification are crucial as they allow for the analysis of gene expression and the detection of specific RNA molecules, notably in viruses.
The importance of RTPCR in our current scientific discourse cannot be overstated. It offers several benefits, including high specificity and sensitivity, which are essential for accurate diagnostics. In the world of viral infections, especially with something like SARS-CoV-2, RTPCR became the gold standard test. This prominence showcases how RTPCR bridges the gap between basic research and real-world applications.
Historical Context
RTPCR is a product of two major advancements in molecular biology that came together in the late 20th century. The inception of PCR by Kary Mullis in 1983 was revolutionary. Mullis provided a method that could amplify DNA exponentially, making it easier to study minute samples. However, it was not until 1990 that the combination of reverse transcription with PCR became a reality, thanks to the growing need to analyze RNA.
Prior to its widespread adoption, the analysis of RNA was a daunting task. Traditional methods were tedious and not particularly reliable, similar to trying to find a needle in a haystack. RTPCR transformed this field by making it possible to detect and quantify RNA efficiently. Historical studies began to highlight its utility in various applications, such as virology and oncology, paving the way for modern diagnostic practices. In essence, RTPCR not only represents technical progress but also a shift in how researchers tackle biological questions.
This understanding of the introduction, definition, significance, and historical context of RTPCR lays a solid foundation for the following sections that will explore the underlying science, components, procedures, and applications of this essential technique.
The Science Behind RTPCR
Understanding the science behind RTPCR is crucial as it lays the foundation for its application in molecular biology. The mechanics of this process reveal not just the how but also the why behind its efficacy in detecting RNA. This subfield is vital for researchers and medical professionals alike, as the underlying technology relies heavily on precision and accuracy.
Mechanism of Reverse Transcription
At the heart of RTPCR lies the mechanism of reverse transcription. This process takes RNA and converts it into complementary DNA (cDNA) using a specific enzyme called reverse transcriptase. This enzyme is what bridges the gap between RNA and DNA, allowing the genetic material to be copied and amplified.
Once the reverse transcription occurs, the cDNA can then be used in subsequent PCR processes. This conversion is significant because RNA, especially in the context of viral infections, is often the primary source of genetic material. By transforming RNA into a form that can be amplified, researchers increase the chances of detecting even the tiniest amounts of genetic material. In practical terms, it means better diagnostics and clearer results in various applications, from healthcare to genetic research.
Polymerase Chain Reaction Fundamentals
The Polymerase Chain Reaction (PCR) is what allows for the amplification of the cDNA produced from the reverse transcription process. Without amplification, the initial amount of genetic material may be too minute for analysis. Thus, PCR is a fundamental part of RTPCR, making it possible to generate millions of copies of specific DNA sequences for either qualitative or quantitative analyses.
Amplification Process
The amplification process within PCR involves several cycles of heating and cooling, enabling the DNA to denature, anneal, and extend. Each cycle doubles the amount of DNA, resulting in exponential growth. This process is beneficial because it turns a sample from potentially undetectable levels to a quantity that is easily analyzed.
A key characteristic of this amplification process is its specificity. By employing carefully designed primers that target particular sequences, PCR ensures that only the wanted sequences are amplified, reducing the chances of background noise from other, unrelated DNA in the sample. The unique advantage here is the ability to achieve high sensitivity and precision, which makes RTPCR extremely useful in AIDS research or detecting viral loads in infected patients.
Thermal Cycling Principles
Central to the PCR amplification process are the thermal cycling principles. This involves carefully controlled temperature fluctuations to create an optimal environment for the reactions to take place. The thermal cycling goes through three main phases: denaturation, annealing, and extension, each held at specific temperatures for precise durations.
The importance of this thermal cycling cannot be overstated; it’s where the magic happens. This cycle enables the enzyme DNA polymerase to replicate the DNA. A significant benefit of these principles is that they allow for rapid amplification, often yielding results in a matter of hours. However, they also demand finely-tuned equipment and ideal conditions, since any discrepancies can lead to failed reactions or suboptimal results, impacting the reliability of the data.
"The integration between reverse transcription and PCR is what truly reflects the sophistication behind molecular diagnostics."
"The integration between reverse transcription and PCR is what truly reflects the sophistication behind molecular diagnostics."
By comprehending the mechanisms of reverse transcription and the fundamental aspects of PCR, one can appreciate the intricacies behind RTPCR. They are not just isolated processes but interconnecting gears in a well-oiled machine that drives the efforts in molecular biology, enabling groundbreaking research and diagnostics.
Components of RTPCR Assay
Understanding the components that make up RTPCR assays is crucial for anyone delving into molecular biology. Each element within an RTPCR setup plays a significant role in ensuring the success of the assay, influencing everything from accuracy to efficiency. When you think about it, it’s not just about having the right mix of ingredients; it’s about how these ingredients come together to create a symphony of processes that lead to meaningful results in research and diagnostics.
Enzymes and Reagents
Enzymes and reagents are the backbone of any RTPCR assay, acting as both catalysts and building blocks for the reaction. Without them, the process couldn't even get off the ground. Let’s break down some of the key players in this arena.
Reverse Transcriptase
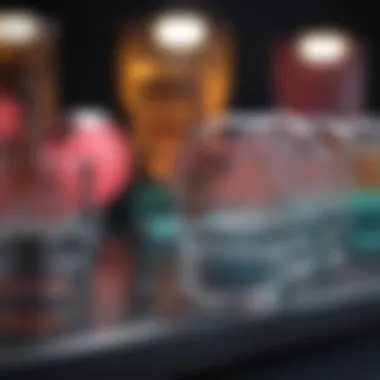
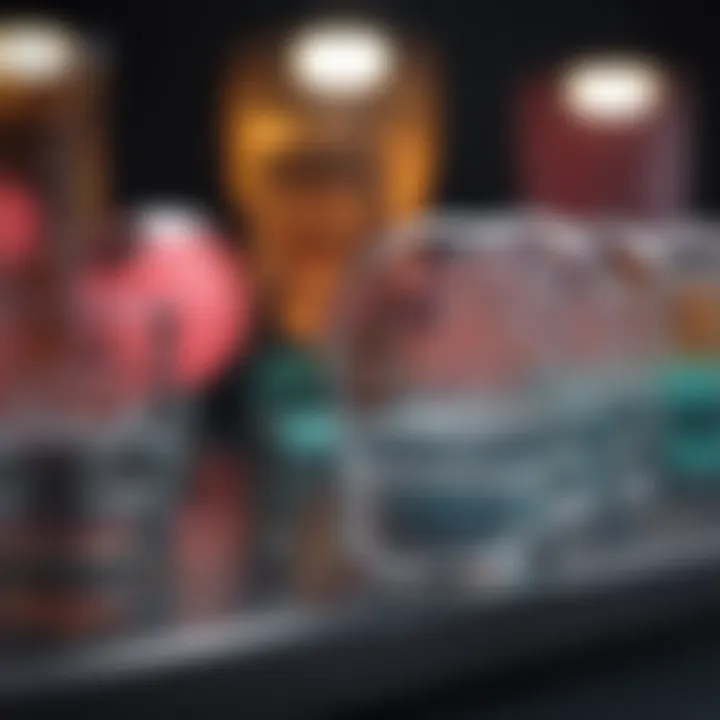
Reverse Transcriptase is often regarded as the unsung hero of RTPCR. This enzyme is responsible for converting RNA into complementary DNA, essentially flipping the script on genetic information. The key characteristic of Reverse Transcriptase is its high fidelity, which minimizes errors during transcription. It ensures the resulting cDNA accurately reflects the original RNA, making it an indispensable tool in both diagnostic and research settings.
A standout feature of Reverse Transcriptase is its ability to work with varying RNA templates, including those that are degraded or present in low quantities. This advantage makes it incredibly useful in detecting RNA viruses or expressing low-abundance genes. However, a potential disadvantage is its slight susceptibility to inhibitors found in biological samples, which can affect efficiency.
Taq Polymerase
Taq Polymerase is another heavyweight in the RTPCR game. This thermostable enzyme is known for its role in amplifying DNA, specifically during the polymerase chain reaction stage. The key characteristic here is its robustness; it can withstand high temperatures used during thermal cycling without losing activity. Taq Polymerase is popular because of its ability to generate large amounts of DNA quickly, crucial when time is of the essence in diagnostic scenarios.
One unique feature of Taq Polymerase is its error rate, which can lead to mutations in amplified products. Although this can be seen as a disadvantage for some applications requiring high precision, its speed and efficiency often outweigh this concern in many routine assays.
Nucleotides
Nucleotides are the building blocks of nucleic acids and play a critical role in RTPCR assays. These small organic molecules are the key characteristic of this component, providing the raw material necessary for synthesizing the new DNA strand. They are critical for catalyzing the reactions and thus directly contribute to the success of the assay.
Nucleotides exhibit a unique feature: they can be added to the reaction mix in varying concentrations to optimize conditions. This flexibility allows researchers and clinicians to fine-tune their experiments based on the requirements. However, one must be cautious, as excessive concentrations can lead to a phenomenon known as self-priming, which can muddle the results.
Primers Design and Selection
Selecting the right primers is akin to choosing the right keys for a lock; it determines whether the RTPCR assay will yield the expected results. The design and selection process involves several considerations, ranging from specificity to efficiency, ensuring that the target sequence is amplified without off-target effects.
Step-by-Step RTPCR Procedure
Understanding the Step-by-Step RTPCR Procedure is crucial for anyone aiming to master this technique. Each phase of the procedure is not just a routine; it's a carefully orchestrated sequence that maximizes the accuracy and reliability of test results. When you talk about RTPCR, you're really looking at a method that’s as much about meticulous planning as it is about scientific principles. Without proper execution, the whole endeavor could go awry, leading to misleading data and ineffective conclusions.
Sample Preparation
The first step in the RTPCR journey is sample preparation. It’s like setting the stage for a grand performance; everything needs to be just right. The quality of the RNA extracted from the sample profoundly impacts the reliability of the results. Proper handling and storage of samples are key to preventing degradation. One must ensure that all enzymes remain intact and functional during the extraction phase.
Moreover, contamination is a lurking threat. Hence, wearing gloves, using sterile equipment, and working in a clean environment are non-negotiable rules. The choice of the extraction kit can also make a world of difference. Brands like Qiagen and Thermo Fisher have specialized kits that simplify this process, allowing for rapid and efficient RNA isolation. The ultimate goal here is to achieve high-purity RNA that is free from inhibitors, ready to undergo reverse transcription and amplification.
Conducting the Reaction
Once you have your sample prepared, the next part of the RTPCR procedure is conducting the reaction itself. At this point, it's essential to follow standard protocols to obtain valid results.
Thermal Cycling Protocols
Thermal Cycling Protocols are like the heart of the RTPCR process. This cycling is what enables scientists to exponentially amplify the target RNA sequence. A typical thermal cycling reaction takes place in three stages: denaturation, annealing, and extension.
- Denaturation involves heat destabilizing the double-stranded RNA, making it single-stranded.
- Annealing allows primers to attach themselves to the single-stranded RNA at specific sites.
- Extension is where Taq polymerase synthesizes new strands of cDNA, effectively duplicating the target sequence.
The key characteristic of these protocols is the precise control over temperature and timing. The ability to modulate these factors is what makes thermal cycling a powerful technique. Scientists can set specific temperatures to optimize each reaction step, resulting in increased yield and specificity. This is why thermal cycling is considered a benchmark method in RTPCR assays, balancing efficiency and precision.
Real-Time Monitoring Techniques
Real-Time Monitoring Techniques build on the solid foundation of thermal cycling. They offer the ability to track the amplification process as it happens, providing a real-time view of reaction kinetics. This insight helps in interpreting results quickly and with higher confidence.
Common methods here include the use of fluorescent dyes, such as SYBR Green or probes like TaqMan. The beauty of real-time monitoring is that it checks for the accumulation of PCR products without needing to pause the whole process for additional analysis steps.
This time-saving characteristic is significant, especially in emergency diagnostic settings, where speed is crucial. However, it’s worth noting that while real-time techniques reduce the need for post-PCR analysis, they also require careful optimization and validation to avoid false positives due to contamination or nonspecific amplification.
"In RTPCR, every detail matters, from the choice of reagents to the fine-tuning of thermal cycles. Missteps can lead to a domino effect, casting doubt on the validity of the results."
"In RTPCR, every detail matters, from the choice of reagents to the fine-tuning of thermal cycles. Missteps can lead to a domino effect, casting doubt on the validity of the results."
Ultimately, grasping the intricacies of the Step-by-Step RTPCR Procedure ensures that researchers and practitioners can confidently navigate this vital molecular biology technique. Each step is a building block towards a solid foundation of reproducible and meaningful scientific inquiry.
Applications of RTPCR
RTPCR has carved out a significant niche within molecular biology, with applications that stretch beyond simple detection methods. This technique plays a crucial role in various fields, particularly in diagnostics and research. The power of RTPCR lies in its ability to amplify and analyze RNA, making it invaluable for studying viral infections and genetic disorders. The benefits of RTPCR extend to its adaptability in different settings, such as clinical laboratories and research facilities. Understanding these applications not only sheds light on the technique itself but also underscores its relevance in the quest for scientific knowledge and health advancements.
Medical Diagnostics
Viral Infections
When it comes to viral infections, RTPCR stands at the forefront of diagnostic testing. Its ability to detect RNA viruses efficiently and accurately makes it a preferred choice in clinical settings. The key characteristic here is the sensitivity of RTPCR, which allows for the detection of even low viral loads, a critical factor during the early stages of infection when viral markers can be sparse.
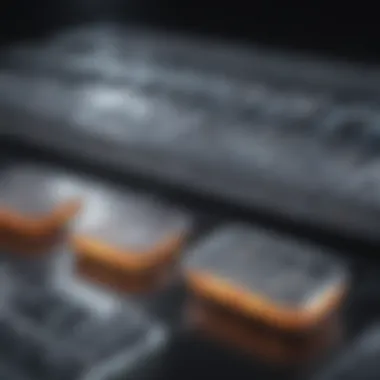
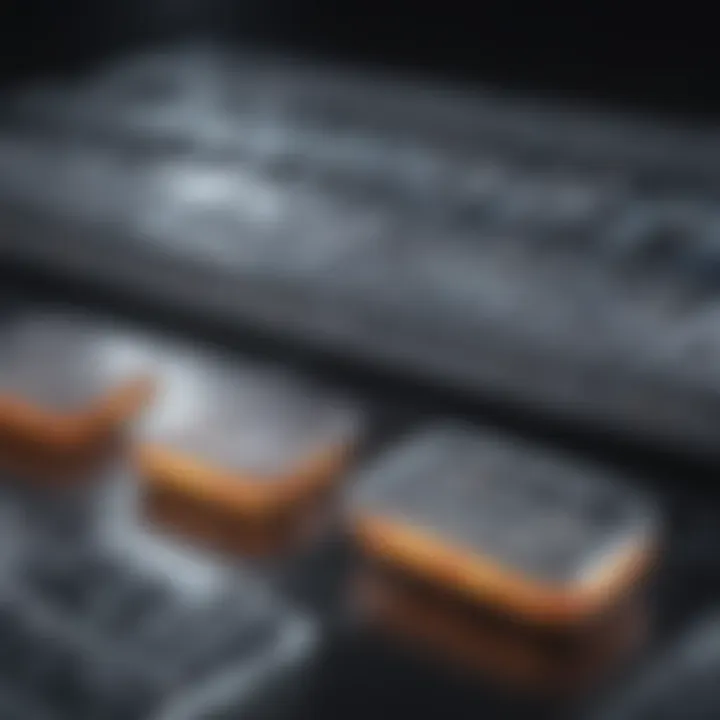
One of the unique features of using RTPCR for viral infections is the speed of results. In outbreak situations, such as when dealing with viruses like SARS-CoV-2, timely diagnostics can inform public health responses effectively. However, there are disadvantages too: As with any scientific technique, RTPCR can be susceptible to contamination, leading to false positives. Thus, meticulous protocols and stringent controls are necessary to ensure the reliability of results.
Genetic Disorders
The application of RTPCR is equally significant in genetic disorders. This technique allows researchers and clinicians to investigate the expression levels of specific genes associated with various inherited conditions. The key advantage lies in its specificity; RTPCR can differentiate between closely related gene variants, offering a clearer picture of genetic mutations.
A noteworthy aspect of using RTPCR for genetic disorders is its ability to facilitate personalized medicine. By analyzing gene expression profiles, healthcare providers can tailor treatments suited to individual patients based on specific genetic expressions. Nevertheless, clinical interpretation of RTPCR results requires skilled professionals, as misinterpretation might lead to improper diagnoses or treatment plans.
Research Applications
Gene Expression Analysis
In the realm of research, gene expression analysis is perhaps the most prominent application of RTPCR. Researchers use this method to quantify mRNA levels across different biological samples. This has vital implications in understanding how gene expression varies under different conditions, such as disease states or treatment responses.
A key characteristic of this area is the enhancement of biological insights through quantitative data. Researchers can determine not just the presence of genes, but also how active they are—an essential factor in developmental biology, cancer research, and pharmacogenomics. Yet, merely focusing on expression levels can oversimplify biological complexity. There are multifactorial elements at play that RTPCR alone cannot decipher, thus, it should be part of a broader toolkit of experimental techniques.
Pathogen Detection
Lastly, RTPCR plays a pivotal role in pathogen detection, especially in identifying bacterial and fungal pathogens. This application highlights the versatility of RTPCR as it can detect RNA from various sources, not limited to viruses alone. One of the main advantages here is the specificity it offers, identifying pathogens that traditional culture methods might miss, particularly in fastidious organisms.
Moreover, its rapid turnaround time relative to conventional microbiological techniques makes RTPCR an attractive option in clinical microbiology labs. However, challenges remain, such as the necessity for precise controls and the potential for cross-contamination, which can complicate interpretation.
Limitations of RTPCR
Though powerful, RTPCR is not without its shortcomings. Recognizing the limitations is crucial for researchers and professionals who rely heavily on this technique. It helps in refinement and ensures results that are reliable and applicable to real-world problems. By grasping the potential pitfalls, users can navigate through challenges effectively while maximizing the benefits of their research.
Technical Challenges
Contamination Risks
Contamination is a major hurdle in the RTPCR process. It can stem from multiple sources, including reagents, equipment, and even environmental agents. When unwanted RNA or DNA is introduced, it can produce erroneous results, leading to false positives or negatives. Addressing contamination is not just a technical necessity but an ethical obligation, especially in clinical diagnostics where patient outcomes might hinge on the accuracy of results.
- Key Characteristics: The signature of contamination is often low-level amplification, which muddles the clarity of genetic signals. Routine checks and stringent laboratory practices can mitigate this risk.
- Benefits: A robust contamination management strategy ensures that RTPCR results remain trustworthy. Clean environments and molecular grade reagents are considered best practices within labs.
- Advantages/Disadvantages: While investing in top-notch cleaning procedures and equipment might incur costs, the risk of misleading findings far outweighs expenses. It’s an investment into reliability.
Efficiency Issues
Efficiency challenges can arise throughout the RTPCR workflow. The technology requires a balance between speed and accuracy, where deterioration in either aspect can lead to disappointing outcomes.
- Specific Aspect: One significant factor is the enzyme efficiency. If reverse transcriptase or polymerase is suboptimal, the resulting yield may not meet the expectations of researchers.
- Key Characteristics: Many labs face issues related to cycle optimization and reagent concentrations, which ultimately affect the overall yield of the reaction.
- Benefits: Overcoming these barriers can lead to faster turnaround times and improved result reliability. Techniques such as optimizing thermal cycling parameters can help.
- Advantages/Disadvantages: While some may look towards rapid assays, faster results can come at the cost of precision. A balanced approach, weighing speed against the fidelity of the data, is fundamental in RTPCR research.
Interpretation of Results
Understanding and interpreting the data generated from RTPCR is a nuanced process. The results must be carefully analyzed in the context of the experiment's setup and controls used in the study. Relying solely on quantitative measures without considering factors like baseline fluctuations can lead scientists astray. This section emphasizes the importance of proper data interpretation:
"The interpretation of RTPCR results requires not just technical skills but also an astute understanding of biological variability."
"The interpretation of RTPCR results requires not just technical skills but also an astute understanding of biological variability."
Here, accuracy in understanding amplification curves and expression levels becomes crucial. Factors, such as signal-to-noise ratio and threshold settings, play pivotal roles in deciding the reliability of results. In short, interpreting RTPCR data isn't merely a mechanical process; it requires thought, context, and often, collaboration with peers to clarify uncertainties.
Recent Advances in RTPCR Technology
Recent advancements in RTPCR technology are reshaping the landscape of molecular biology and diagnostics. Continuous innovation enhances both sensitivity and specificity, making these methods invaluable for researchers and healthcare professionals alike. By integrating cutting-edge technologies and refining existing protocols, scientists have taken significant leaps forward in understanding and utilizing RTPCR for diverse applications. This section explores two prominent areas of recent developments that deserve attention: Digital RTPCR and Nanotechnology Integration.
Digital RTPCR
Digital RTPCR represents a transformative shift in how quantitative measurements are made. Traditional RTPCR techniques often struggle with quantifying low abundant RNA targets due to the background noise that can obscure results. Digital RTPCR, on the other hand, separates the sample into thousands of individual reactions, allowing for precise counting of positive signals. Each droplet or well effectively acts as its own micro-reaction chamber, making this method far more sensitive and reliable in quantifying nucleic acid concentrations in diverse samples.
The advantages of Digital RTPCR include:
- Increased Sensitivity: This method can detect minute amounts of RNA, essential for early diagnosis of diseases like cancer and viral infections.
- Higher Precision: Researchers can quantify targets at single-molecule resolution, providing a clear understanding of expression levels.
- Robust Data Analysis: It reduces variability seen in conventional methods, yielding more reproducible results.
These features make Digital RTPCR an excellent choice if researchers aim to study low-expression genes or analyze heterogeneous populations of RNA.
Nanotechnology Integration
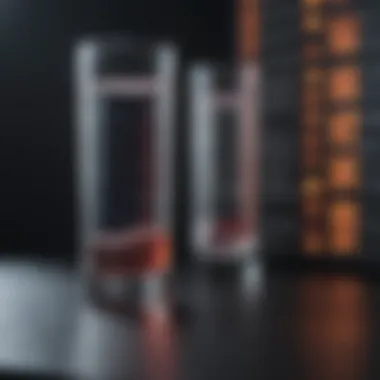
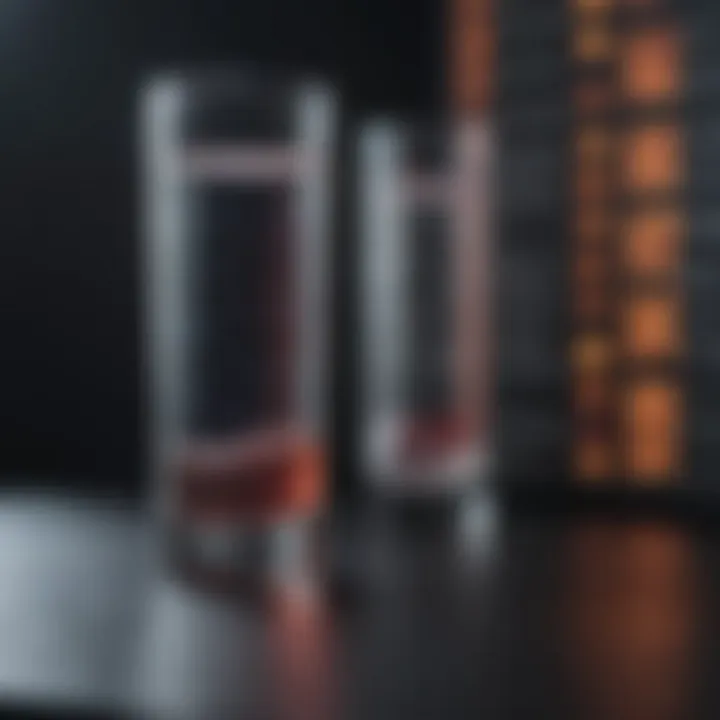
The pairing of RTPCR with nanotechnology opens new avenues for enhancing diagnostic capabilities and performance. By leveraging nanoparticles, researchers can improve assay efficiency, reduce reaction times, and increase throughput. For instance, gold nanoparticles have been utilized to facilitate the visualization of PCR products, making the process quicker and more streamlined.
Key benefits of integrating nanotechnology into RTPCR include:
- Enhanced Signal Detection: Nanoparticles can amplify signals, allowing for improved sensitivity and lower detection limits.
- Miniaturization of Assays: This integration leads to smaller, more efficient devices that minimize sample volumes and costs involved.
- Tailored Surface Chemistry: Customization of nanoparticle surfaces can improve interactions with target nucleic acids, leading to higher binding specificity.
This synergy between nanotechnology and RTPCR exemplifies the direction that modern molecular diagnostics is taking, as scientists increasingly seek to develop more efficient and user-friendly testing methods.
"The future of RTPCR relies on adaptability and the integration of innovative technologies to meet the evolving demands of research and clinical applications."
"The future of RTPCR relies on adaptability and the integration of innovative technologies to meet the evolving demands of research and clinical applications."
For further reading and insights into recent technology developments in molecular biology, you might find these resources helpful: Wikipedia, Britannica.
Ethical Considerations in RTPCR Testing
The ethical implications of RTPCR testing cannot be overstated, considering its crucial role in diagnostics and research. Since RTPCR is frequently employed to detect viral infections or genetic disorders, ensuring ethical guidelines is vital. Missteps in ethics can lead to significant consequences, not just for individuals tested but for the broader community as well. Topics like informed consent, the protection of personal data, and potential biases in testing methods highlight the importance of ethical considerations in this field.
Data Privacy Concerns
The use of RTPCR tests often involves the handling of sensitive personal information. Data privacy is paramount here, as unauthorized access or leaks can have dire repercussions for individuals. For instance, if test results showing a positive diagnosis for a contagious disease are mishandled, it can lead to stigma or discrimination.
To address these concerns, laboratories and researchers need to implement stringent data management protocols. This includes:
- Encrypting personal data to prevent unauthorized access.
- Regular audits to ensure compliance with data protection regulations.
- Ensuring that only essential personnel have access to sensitive information.
Moreover, transparency in how data is collected and used can mitigate fears surrounding privacy. Patients should be educated about their rights regarding personal data usage in RTPCR tests, ensuring informed consent.
Implications of Misdiagnosis
Misdiagnosis is perhaps one of the most concerning possibilities in RTPCR testing. Due to its sensitivity, a false positive or negative result can lead to unwarranted panic or a false sense of security. A false positive could thrust an individual into unnecessary treatment regimens, while a false negative could allow a pernicious disease to propagate without detection.
Consider the scenario during a viral outbreak like COVID-19; an inaccurate RTPCR result could significantly worsen the situation. Therefore, proper training and stringent quality control measures must be in place to minimize misdiagnoses.
A deeper dive into the implications of misdiagnosis reveals the character of responsibility that laboratories and healthcare providers hold. They are charged not just with analyzing data but also with communicating findings effectively and ethically. Making sure that patients understand their results, the potential for error, and the necessity of confirmatory testing when needed can help to alleviate some risks.
In addressing ethical considerations, the RTPCR landscape is one that poses a myriad of responsibilities for professionals in the field.
"Informed consent is not just an obligation; it is a hallmark of respect and integrity in medical practice."
"Informed consent is not just an obligation; it is a hallmark of respect and integrity in medical practice."
By taking these ethical factors into account, the future of RTPCR testing can foster trust and integrity within a field that is indispensable to modern biology and medicine.
Future Directions for RTPCR Research
In recent years, the dynamic landscape of molecular diagnostics has experienced a significant shift, particularly with regards to Reverse Transcription Polymerase Chain Reaction (RTPCR) assays. As the demand for rapid, accurate, and user-friendly testing grows, exploring the future directions of RTPCR research is not just an academic endeavor; it’s a necessity for both medical and scientific communities alike. This section articulates the potential advancements and innovations that could reshape how we understand and utilize RTPCR.
Integration with Other Technologies
As RTPCR evolves, one key trend is its integration with other technologies. The ability to combine RTPCR with next-generation sequencing (NGS), for instance, opens up new avenues for gene discovery and analysis. This synergistic approach allows researchers to not only amplify RNA but also conduct a comprehensive examination of genetic material in a single workflow.
- Benefits of Integration
- Considerations in Integration
- Enhanced Specificity: Combining RTPCR with NGS can improve the specificity of pathogen identification. This application is especially critical in the context of infectious diseases where precise detection can inform treatment strategies.
- Data Richness: Integrating different technologies elevates the amount and quality of genomic data available for analysis. This can lead to better understanding of disease mechanisms, offering insights that RTPCR alone may not provide.
- Cost and Accessibility: Merging these technologies can make testing more expensive and might not be easily accessible in resource-limited settings.
- Technical Expertise: As complexity increases, so does the need for trained personnel who can manage and interpret data from multiple platforms.
Potential for Point-of-Care Testing
Looking ahead, one of the most compelling prospects for RTPCR is its use in point-of-care testing (POCT). The ability to conduct RTPCR assays outside conventional lab settings could transform patient management and treatment outcomes.
- Advantages of POCT
- Challenges of Implementation
- Timeliness: Rapid testing can lead to quicker medical decisions. In viral outbreaks, where early intervention is key, the ability to do tests at the bedside can make all the difference.
- Patient Satisfaction: Performing tests on-site can enhance the patient experience. No one enjoys waiting days for results when treatment could have started days earlier.
- Regulatory Hurdles: Developing FDA-approved POCT kits requires rigorous validation processes that can take time.
- Quality Control: Ensuring that assays maintain accuracy and reliability at the point of care is paramount. Reducing the risk of user error in non-laboratory settings is a critical challenge.
"The future of RTPCR rests not only in refining existing techniques but also in bridging its capabilities with other technologies to create a more robust, reliable, and convenient testing paradigm."
"The future of RTPCR rests not only in refining existing techniques but also in bridging its capabilities with other technologies to create a more robust, reliable, and convenient testing paradigm."