Understanding Water Permeation: Concepts and Uses
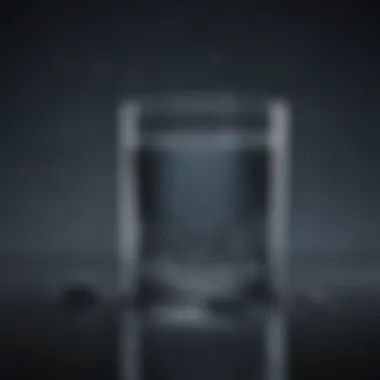
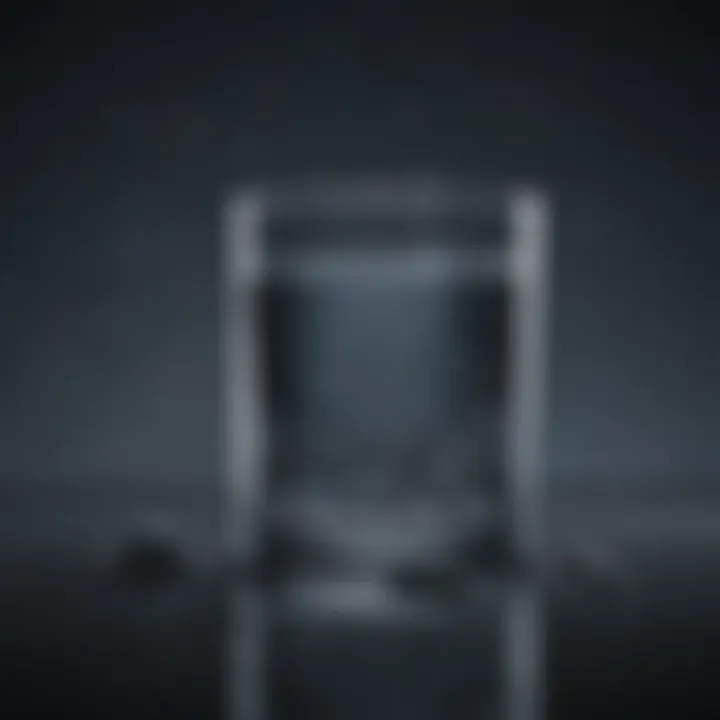
Intro
Water plays a vital role in both our daily lives and the broader ecosystem. Understanding how it permeates various materials goes beyond simple curiosity; it has significant implications in various fields, from biology to environmental science. This article will present a comprehensive overview of water permeation, shedding light on its principles, mechanisms, and applications.
As we journey through this exploration, we'll break down complex ideas into digestible parts, making connections between scientific principles and their practical implications. So, let’s roll up our sleeves and dive into the essential concepts that will shape our understanding of water permeation.
Key Concepts
Definition of the Main Idea
Water permeation refers to the process by which water moves through different materials or mediums. This can occur through physical barriers or biological membranes. The ease with which water permeates a material can vary greatly and depends on several factors, including the material's properties and the environment's conditions.
In simpler terms, think of a sponge soaking up water. The sponge has pores that allow water to get through it, much like how various materials or biological tissues interact with water. The complex dance of molecules at play here is essential for understanding water's behavior in natural and engineered systems.
Overview of Scientific Principles
To truly grasp water permeation, it’s important to touch on a few scientific principles. These principles provide the groundwork for various applications, be it in designing water filtration systems or understanding how plants uptake moisture.
- Diffusion: This is the natural movement of water from an area of higher concentration to a lower concentration. Imagine pouring sugar into a glass of water; over time, the sugar will spread out evenly, just as water does in permeable materials.
- Osmosis: This specialized form of diffusion focuses specifically on water molecules and is generally seen in biological membranes. It explains how cells maintain their proper hydration levels. For instance, when plant roots absorb water from the soil, they are utilizing the principles of osmosis.
- Capillary Action: This refers to the ability of water to flow in narrow spaces without the assistance of external forces. It's a crucial mechanism in soil-water movement and a phenomenon that allows trees to draw water from their roots all the way to their leaves.
- Hydraulic Permeability: This term defines how easily water moves through soil or rocks. Various types of soil or geological materials have different permeabilities, influencing water availability in ecosystems.
Understanding these fundamental principles is essential when discussing the implications of water permeation in biological and ecological contexts.
Understanding these fundamental principles is essential when discussing the implications of water permeation in biological and ecological contexts.
In the sections that follow, we’ll take a closer look at recent research trends and the breakthroughs shaping our understanding of water permeation in various fields. Whether one is deep in the trenches of scientific research or just dipping their toes into the subject, the insights into water's behavior will provide valuable knowledge for all.
Prelims to Water Permeation
Water permeation is more than just a scientific curiosity; it’s one of the essential threads in the vast tapestry of life and environmental interactions. Understanding water's journey through various mediums is crucial in numerous fields, including biology, environmental science, and material engineering. The behavior of water permeation can determine how nutrients reach plants, how drugs are delivered in medical therapies, and even how pollutants move through soil.
The principles underlying water permeation help us appreciate its multifaceted role. When we discuss permeation, we’re really diving into the molecular intricacies of how water molecules interact with their surroundings. It’s about recognizing that water is not just a passive element; it actively participates in various chemical and biological processes. This notion is vital for students, educators, and professionals alike, as it lays a foundation for understanding more complex concepts in diverse scientific domains.
One key benefit of studying water permeation is the insight it provides into optimizing agricultural practices. Such knowledge helps in tailoring irrigation methods suitable for different crops while also considering environmental impact. Additionally, a firm grasp of water dynamics supports the design of innovative biomedical engineering solutions, such as enhancing drug absorption through tissues.
Moreover, considerations about water permeation are embeddings for critical environmental issues today. As we grapple with challenges like climate change and ecosystem degradation, understanding water movement within systems becomes pivotal. Through this article, we aim to illuminate these complex interactions and their implications for both the natural world and human activities.
Definition of Water Permeation
When people speak of water permeation, they refer to the process by which water molecules pass through a barrier, usually a semi-permeable membrane or a porous material. At its core, it’s a dynamic balancing act, driven largely by concentration gradients and the principles of diffusion. The definition encompasses several dimensions, not merely the action of water passing through but the intricate forces and characteristics governing that flow.
This definition is especially relevant in biological contexts, where cells utilize selective permeability to maintain internal stability while allowing essential nutrients to enter and waste products to exit. In simple terms, water permeation is the lifeblood of biological systems, supporting everything from cellular function to growth in higher organisms.
Historical Context of Water Studies
Water studies trace a fascinating lineage back to early explorations in natural sciences. In ancient civilizations, water was often viewed through practical lenses—its availability dictated the success of agriculture and settlements. However, the formal study of water and its permeation really began to pick up steam during the 18th and 19th centuries with the advent of modern chemistry and biological sciences.
Researchers like Robert Boyle and later Louis Pasteur laid some of the groundwork in understanding fluid dynamics and biological interactions. The term ‘osmosis,’ crucial to the conversation on water permeation, was established in the late 1800s, aiding the understanding of how substances interact with water across membranes. Such historical context not only broadens our understanding of water's importance but also gives us a sense of how far scientific inquiry has come.
As history marches on, we find ourselves at the convergence of numerous disciplines—chemistry, biology, and environmental science, among others—all using this foundational knowledge on water permeation to propel research that addresses both age-old questions and modern challenges in an ever-evolving world.
Fundamental Concepts of Permeation
Understanding the fundamental concepts of permeation is crucial for grasping the wider implications of how water interacts with various materials, especially in biological and environmental contexts. At its core, permeation is not just a passive event; it’s a dynamic process influenced by various mechanisms and factors. Grasping these principles can lead to advancements in real-world applications, from improving agricultural practices to enhancing biomedical technologies.
Diffusion and Osmosis: Key Mechanisms
To get into the nitty-gritty, we first need to look at diffusion and osmosis.
Diffusion is the process where molecules move from an area of higher concentration to an area of lower concentration. It’s like when you open a bottle of perfume in a room; slowly, the scent dissipates until it fills the space uniformly. This principle is fundamental to many biological and chemical processes. On the other hand, osmosis is a specialized type of diffusion applicable only to water. It describes how water moves through semi-permeable membranes, usually from areas of low solute concentration to high solute concentration, trying to balance things out.
These mechanisms are painted vividly in biological systems. For instance, think of how plant roots absorb water from the soil. It’s through osmosis that the roots draw water in, effectively supporting the plant's hydration and nutrient uptake.
Factors Affecting Permeation Rates
Permeation rates are not static; they fluctuate based on several factors. Here we'll dive into three significant aspects: concentration gradients, temperature influence, and the properties of solvents and solutes.
Concentration Gradients
Concentration gradients directly influence the rate at which permeation occurs. When there’s a steep concentration gradient, molecules will flow from the area of high concentration to low—similar to how a crowd disperses in a spacious room. A sharp gradient can dramatically enhance permeation rates, making it a vital consideration in both biological and industrial processes. For instance, in drug delivery systems, understanding the concentration gradient helps scientists design more effective medications that target specific areas in the body. However, as beneficial as steep gradients can be, they can also lead to rapid changes that the system might not withstand, sometimes causing imbalances in biological systems.
Temperature Influence
Temperature also plays a pivotal role in water permeation. Higher temperatures generally increase the kinetic energy of molecules, leading to faster movement and, consequently, higher permeation rates. Imagine boiling water—molecules are in constant motion, trying to escape into steam. This characteristic is especially significant in processes like evaporation and transpiration in plants. However, there's a fine balance. At excessively high temperatures, some biological membranes can become compromised, leading to leakage or loss of function. Hence, while temperature can enhance permeation, it requires careful regulation to avoid disruption.
Solvent and Solute Properties
The physical and chemical properties of solvents and solutes also dictate permeation dynamics. For instance, polar solvents like water can dissolve ionic compounds very well, facilitating their permeation through membranes. Conversely, nonpolar substances struggle to navigate polar environments, affecting their transport across different barriers. This key characteristic indicates that the nature of the solvent can either promote or hinder the passage of solutes, which is significant in designing effective drug delivery systems. Unique interactions also highlight potential drawbacks; for instance, solute size and charge can impede permeation, creating chinks in the armor of planned processes.
Understanding these fundamental concepts not only enriches our insight into the mechanisms driving permeation but also sets the stage for exploring applications across various fields, from systemic biology to innovative technologies.
Water Permeation in Biology
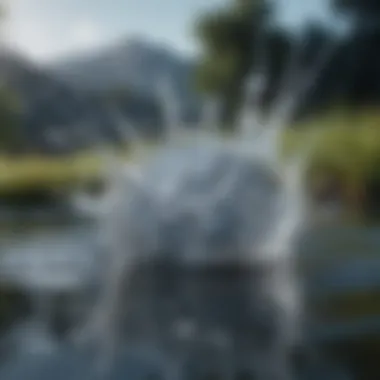
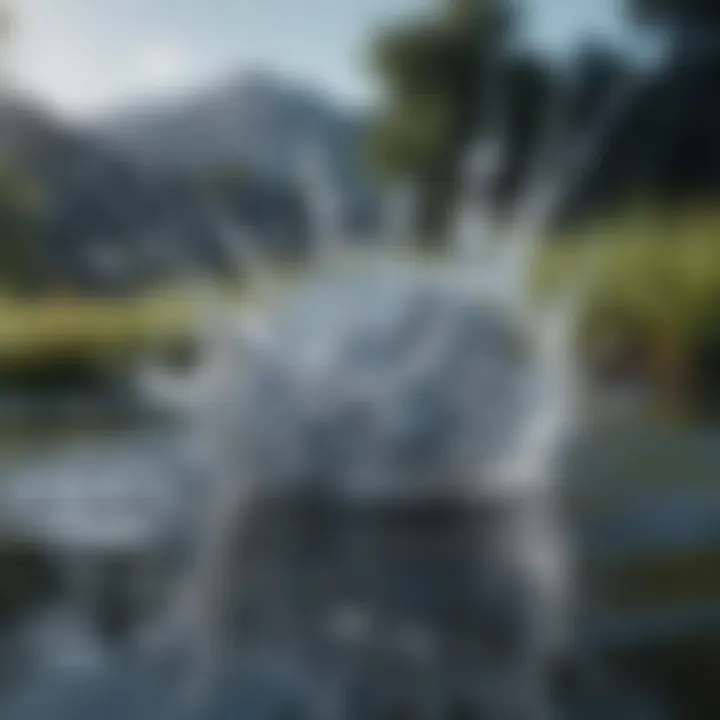
Water permeation is a cornerstone of biological processes. Understanding how water moves through various systems informs us about cellular function, plant health, and even ecosystems. Water acts as a solvent, temperature regulator, and participant in biochemical reactions. This section aims to shed light on two significant aspects of water permeation in biology: cell membrane dynamics and aquaporins, which are specialized channels facilitating rapid water transport.
Cell Membrane Dynamics
Cell membranes are more than just barriers; they are dynamic structures that manage the flux of substances, including water. Composed of a bilayer of phospholipids, these membranes provide a selective environment, crucial for maintaining homeostasis. The way water permeates through these membranes affects everything from nutrient uptake to waste removal.
Key Points about Cell Membrane Dynamics:
- Fluidity: The fluid mosaic model explains that lipids and proteins within the membrane are not static. This fluidity allows for the movement and rearrangement of membrane components, affecting permeability.
- Selective Permeability: Only specific molecules can pass freely through the lipid bilayer, driven by concentration gradients. Water, however, moves through specialized structures due to its polar nature.
- Endocytosis and Exocytosis: Membrane dynamics also include processes like endocytosis, where cells engulf substances, and exocytosis, where materials are expelled. These processes highlight how membranes manage the passage of water along with nutrients.
In biological entities, the dynamic nature of cell membranes is essential for efficient functioning. Membrane disruptions can result in impaired water balance, leading to conditions such as dehydration or overhydration, which shows just how critical water permeation is in living systems.
Aquaporins: Specialized Water Channels
Aquaporins are embedded proteins found in the membranes of many cell types, especially in tissues requiring rapid water transport, such as kidneys and plant roots. These channels play an outsized role in maintaining water balance and regulating cellular water composition.
Features of Aquaporins:
- Selective Water Channels: They facilitate the rapid passage of water molecules while simultaneously preventing the passage of ions and other solutes. This selectivity is crucial in maintaining osmotic balance.
- Signaling Role: Recent studies suggest that aquaporins also have roles beyond transport. They may be involved in signaling pathways that affect cell growth and response to environmental stresses.
- Hydropathy: Their structure exhibits hydrophobic regions that align with the lipid bilayer, which is pivotal for their function. Disruptions in aquaporin activity can lead to significant physiological differences, such as impaired kidney function or drought stress in plants.
"Aquaporins are like nature's water faucet, precisely controlling how much flows in and out."
"Aquaporins are like nature's water faucet, precisely controlling how much flows in and out."
Given the biological importance of water permeation, both cell membrane dynamics and aquaporins are essential in understanding the overall health and functionality of organisms. They illustrate how the intricate design of cells supports not just individual cell life but the larger systems in which these cells operate.
In sum, exploring water permeation in biological contexts provides crucial insights that extend into practical fields like medicine and agriculture, showcasing the relevance of these mechanisms in real-world applications.
Chemical Properties of Water and Permeation
Water is often referred to as the universal solvent, and its chemical properties play a key role in how it interacts with various substances. Understanding these properties is crucial, especially in the context of permeation, as they directly influence processes in biological systems, environmental studies, and even industrial applications. Water’s unique characteristics allow it to engage in various interactions, which in turn affect its ability to permeate different materials.
Polarity and Hydrogen Bonding
Water molecules are polar, meaning they have a partial positive charge on one side and a partial negative charge on the other. This polarity results from the uneven distribution of electrons between the oxygen and hydrogen atoms. Such a structure enables water to form hydrogen bonds—a type of weak interaction—with itself and with other polar substances.
This property significantly affects permeation. For instance, polar solvents often dissolve ionic compounds and other polar substances efficiently due to their ability to stabilize charged ions through hydration shells. This effectiveness facilitates various biological processes, such as nutrient absorption in plant roots and the movement of molecules across cell membranes.
One must not overlook that the strength of hydrogen bonding in water lends itself to high surface tension and a relatively high boiling point compared to other similar-sized molecules. This not only allows for water’s liquid state over a wide range of temperatures but also supports aquatic ecosystems by providing a stable habitat.
Interactions with Other Molecules
Interactions that water exhibits with other molecules are critical in understanding permeation processes. The nature of these interactions can vastly differ based on molecular characteristics.
Ionic Compounds
Ionic compounds consist of positively and negatively charged ions. When these compounds dissolve in water, the polar molecules surround the ions, which breaks ionic bonds and allows the ions to separate and disperse. This process illustrates how water’s ability to interact with ionic compounds can influence the movement of necessary nutrients within biological systems. Moreover, the hydration of ions plays a vital role in cellular processes such as maintaining osmotic balance and facilitating transport mechanisms.
A key characteristic of ionic compounds is their high solubility in polar solvents like water. As a result, these compounds are often used in various agronomic practices, such as fertilizers, which rely on water to transport nutrients to plants quickly. However, the disadvantages may arise in excess, leading to leaching and environmental degradation, making it a double-edged sword in agricultural applications.
Nonpolar Solvents
Nonpolar solvents do not carry any significant charge, which makes them less likely to interact with polar molecules like water effectively. This contrasts sharply with how ionic compounds behave in a similar environment. Nonpolar solvents might disrupt permeation in biological contexts, such as when they replace water in cellular membranes, potentially leading to cellular dysfunction.
However, nonpolar solvents can dissolve other nonpolar compounds effectively, making them valuable in industrial applications, such as extracting oils or fats. One unique feature of these solvents is their ability to mix with other nonpolar substances to form solutions that could be useful for various manufacturing processes.
In summary, the unique properties of both ionic and nonpolar compounds highlight the diverse aspects of water permeation. Water acts as a versatile medium, facilitating essential biological functions while also posing challenges when understanding interactions with nonpolar molecules.
Understanding the chemical properties of water is fundamental to grasp permeation processes across various systems, influencing agriculture, ecology, and industrial applications alike.
Understanding the chemical properties of water is fundamental to grasp permeation processes across various systems, influencing agriculture, ecology, and industrial applications alike.
Permeation in Environmental Sciences
Water permeation plays a critical role in environmental sciences, influencing various natural processes and human activities. Understanding how water moves through different layers of soil, interacts with plant roots, and affects ecosystems provides valuable insights for effective management of natural resources. This section emphasizes the integral relationship between water movement and environmental health, focusing on two pivotal aspects: soil water movement and its impact on plant growth.
Soil Water Movement
Soil water movement is crucial for sustaining ecosystems. It's the process by which water travels through soil layers, driven primarily by gravity and capillary forces. When rain falls, the water doesn’t sit idly on the surface; instead, it infiltrates into the ground, filling up pore spaces in the soil.
Several factors affect this movement:
- Soil Composition: Sandy soils drain quickly, while clay retains water.
- Soil Structure: Well-structured soils improve permeability, allowing better water flow.
- Temperature and Moisture: Warm temperatures can increase evaporation, affecting soil moisture levels.
Understanding soil water movement can help agencies predict water availability, assess groundwater levels, and mitigate flooding. Efficient irrigation practices are also informed by this knowledge, ensuring water reaches plant roots without wastage.
Impacts on Plant Growth
The relationship between water permeation and plant growth cannot be overstated. Plants need water not just to survive but to thrive. When water permeates through the soil, it dissolves nutrients, making them accessible to plant roots. Without proper water movement, the nutrient uptake is severely restricted, leading to stress and reduced growth.
Consider these aspects:
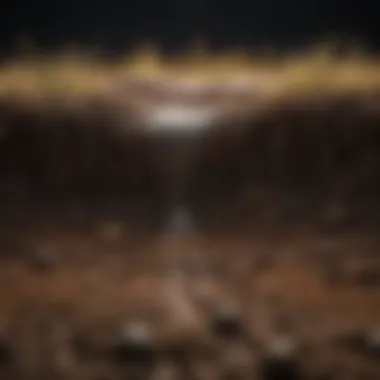
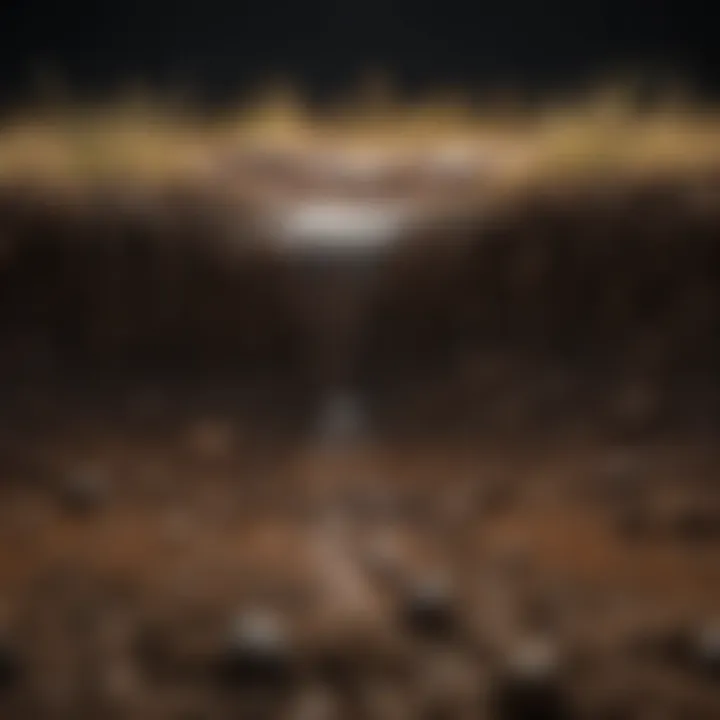
- Optimal Water Levels: Plants need just the right amount of water. Over-saturation can drown roots, while too little can cause wilting.
- Root Development: Adequate water permeation encourages roots to grow deeper and spread wider. This enhances the plant's stability and nutrient absorption capability.
- Ecosystem Balance: Healthy plants contribute to overall ecological balance. They support habitats for various organisms, regulate temperature, and stabilize soils.
A well-balanced water-permeation system not only fosters plant health but sustains entire ecosystems.
A well-balanced water-permeation system not only fosters plant health but sustains entire ecosystems.
Applications of Water Permeation Knowledge
The exploration of water permeation does not just stay in scientific theory; it finds its limbs extended into practical applications across various fields. Knowing how water interacts with different materials has profound implications, especially in agriculture and biomedical engineering. Understanding permeation allows us to improve efficiency in irrigation and drainage, enhancing crop yields and resource management. In healthcare, the principles of water permeation steer innovations in drug delivery systems and tissue engineering.
By tapping into this knowledge, we can drive better practices and technologies that not only optimize processes but also address urgent needs in sustainability and healthcare.
Agricultural Practices
Irrigation Efficiency
Irrigation efficiency is a cornerstone in agricultural practices aimed at maximizing yield while minimizing water wastage. Simply put, high irrigation efficiency means delivering the right amount of water to crops at the right time. This can significantly enhance the overall productivity of agricultural lands while conserving precious water resources.
One key characteristic of irrigation efficiency is its responsiveness. For instance, techniques like drip irrigation and surface irrigation systems have been developed to ensure that water seeps directly to a plant's roots, reducing runoff and evaporation. This particular feature makes it a most favorable option for farmers in regions that often suffer from water scarcity.
However, while drip systems are effective, they can also be expensive to install and maintain. Balancing this cost against the long-term water savings and increased crop efficiency is crucial for farmers deciding on their irrigation approach.
Drainage Management
On the flip side lies drainage management, pivotal in ensuring that excess water does not hinder crop health. This process is particularly important in areas prone to heavy rainfall or poorly draining soils, where excess water can lead to root rot or other diseases. The key characteristic of drainage management is its ability to prevent waterlogging, which can devastate crop yields.
Implementing well-designed drainage systems aids in optimizing soil conditions, thus promoting healthier plant growth. The unique feature of drainage management systems is their ability to be tailored to specific environmental conditions—be it tile drainage, surface drainage, or even subirrigation methods.
Nonetheless, these systems can demand significant initial investments and ongoing maintenance, making it essential for growers to weigh these factors carefully.
Biomedical Engineering
Drug Delivery Systems
Turning eyes toward biomedical engineering, drug delivery systems stand out as a vital application of water permeation principles. These systems are designed to ensure that therapeutic agents reach their targets effectively and efficiently. The hallmark of an efficient drug delivery system is its ability to navigate through various biological membranes, leveraging the principles of permeation.
For example, using hydrogels that mimic biological environments can enhance the release of medication in a controlled manner. These systems can provide sustained delivery, which is beneficial for chronic illnesses where constant medication levels are crucial. A unique feature of these systems is their adaptability; they can be designed to respond to physiological triggers, such as pH changes or specific enzymes, making them notably efficient.
However, challenges remain, such as maintaining the stability of drugs during transit and avoiding side effects. These considerations must be accounted for to achieve optimal therapeutic outcomes.
Tissue Engineering
Another significant aspect of biomedical engineering is tissue engineering, where the aim is to regenerate damaged tissues or organs. In this context, understanding water permeation is essential for creating scaffolds that facilitate nutrient exchange and waste removal. The key characteristic here is the scaffold's porosity and how it allows fluid movement, which is vital for the survival of embedded cells.
By designing scaffolds that closely mimic the natural extracellular matrix, researchers can promote better cell growth and functionality. A notable feature in this field is the creation of bioactive materials that not only support tissue structure but also actively influence cellular behavior.
Nevertheless, the challenge often lies in ensuring long-term durability and biocompatibility of these engineered tissues. Striking a balance between an effective delivery of nutrients and mechanical strength is paramount for the success of such endeavors.
"Understanding water permeation is not merely an academic exercise; it is a gateway to innovative solutions in agriculture and medicine that foster sustainability and enhance life quality."
"Understanding water permeation is not merely an academic exercise; it is a gateway to innovative solutions in agriculture and medicine that foster sustainability and enhance life quality."
Through the applications of water permeation knowledge, we can truly appreciate how intertwined this topic is with real-world challenges, paving the way for informed decision-making and technology development.
Technological Advances in Water Permeation Studies
The study of water permeation has entered a new chapter, fueled by recent technological advancements that open up avenues previously thought unreachable. In an age where accuracy is paramount, understanding how to measure and model the movement of water through various mediums like membranes or soil provides invaluable insights for researchers and professionals across diverse fields.
Recent Innovations in Measurement Techniques
In the realm of water permeation, measurement techniques have come a long way. Traditional methods often struggled with accuracy and could not capture the complexities involved in permeation dynamics. However, innovations such as advanced spectroscopic methods, porosimetry, and high-resolution imaging are changing the game. For instance, using techniques like nuclear magnetic resonance (NMR) and X-ray computed tomography, scientists can visualize how water interacts at the microscopic level with different materials.
These new technologies provide:
- Enhanced accuracy: Measurements are more precise, allowing for better quantification of permeation rates.
- Real-time monitoring: Techniques like acoustic emission monitoring enable continuous observation of water movement, crucial for fields like environmental science.
- Detailed 3D models: High-resolution imaging allows for the creation of 3D maps that reveal how water flows through complex structures.
This leap in measurement capabilities lays a foundation for deeper investigation into both biological and industrial processes, fostering innovations in water management and conservation efforts. The significance of these advancements can't be overstated; they are crucial for modeling processes that affect everything from crop yields to pollution mitigation.
Software Simulations and Modeling
Simulations are the secret sauce for understanding water permeation in a world teeming with complexity. Advanced software models can simulate various environmental conditions, allowing researchers to predict how water will behave under different scenarios without the need for exhaustive field experiments.
These models leverage massive datasets and sophisticated algorithms, creating a virtual landscape where researchers can manipulate variables to study their effects on water movement. For instance, software like COMSOL Multiphysics allows users to solve complex physical problems relating to permeation by modeling interactions in multi-phase systems.
Key benefits of these simulations include:
- Cost-efficiency: Conducting virtual experiments reduces the need for extensive physical testing, saving time and resources.
- Scalability: Researchers can test theories over a large range of scenarios, helping to refine their understanding without the boundaries imposed by fieldwork.
- Predictive powers: Models can forecast future permeation behaviors based on current conditions, which is critical in sectors like agriculture and environmental management.
Ultimately, the blending of novel measurement techniques with robust software simulations represents a potent toolkit for those diving deep into the mechanics of water permeation.
"The integration of advanced technologies not only enhances our understanding of water dynamics but also arms scientists with the tools necessary for addressing pressing global challenges like water scarcity and pollution."
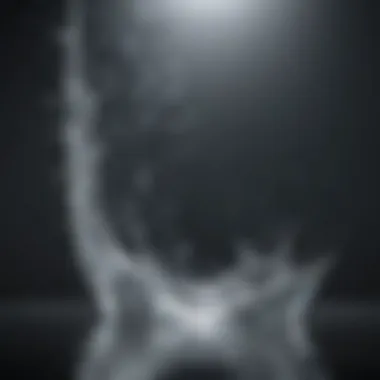
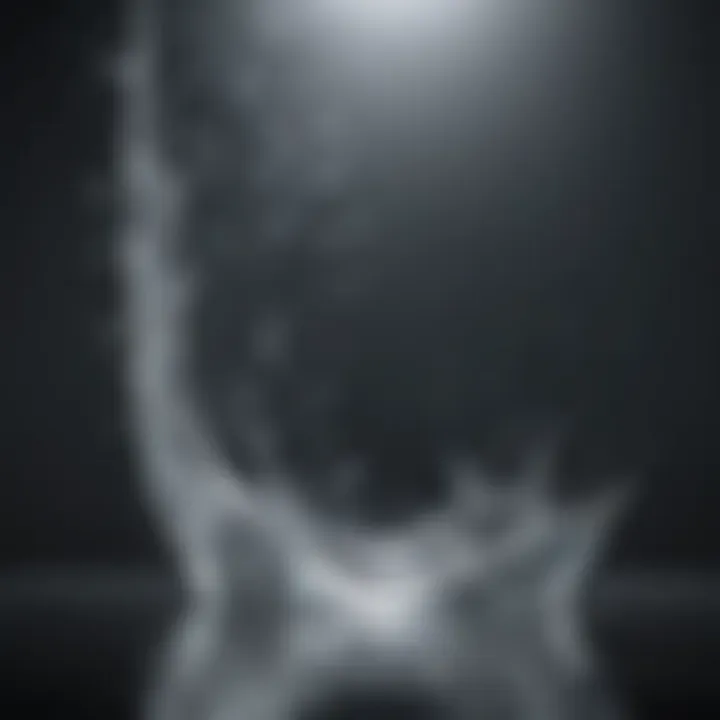
"The integration of advanced technologies not only enhances our understanding of water dynamics but also arms scientists with the tools necessary for addressing pressing global challenges like water scarcity and pollution."
In sum, these technological advances are not merely upgrades; they represent a fundamental shift in how we approach the study of water permeation, making insights more rigorous and applicable than ever before.
Challenges and Limitations in Permeation Research
Understanding the challenges and limitations inherent in permeation research is crucial. The complexity of permeation processes is not just a matter of physics or chemistry; it interlaces across multiple disciplines. Tapping into these challenges allows for a deeper assessment of permeation phenomena and the practical applications of the knowledge gained.
One of the critical challenges researchers face is understanding complex systems. Water permeation does not occur in isolation; it’s influenced by a myriad of factors ranging from molecular characteristics to environmental conditions. This interconnectedness can often lead to unpredictable behaviors, making it difficult to establish clear cause-and-effect relationships. For instance, in biological systems, the permeation of water through cell membranes is not merely a function of osmotic gradients but also deeply affected by the membrane's structural properties. The presence of proteins, such as aquaporins, complicates this relationship further since they selectively facilitate or inhibit water movement, depending on the physiological state of the cell or the surrounding environment.
To effectively study these systems, researchers must employ a combination of tools and methodologies. These might include experimental lab setups, computational simulations, or theoretical models. Each method has its strengths and weaknesses, but they often need to be integrated to paint a comprehensive picture. One significant limitation arises from this necessity; models might oversimplify reality, leading to predictions that don’t hold true in practical scenarios.
"The level of complexity can be daunting, but addressing these challenges is essential for advancing our understanding of water permeation in real-world systems."
"The level of complexity can be daunting, but addressing these challenges is essential for advancing our understanding of water permeation in real-world systems."
Understanding Complex Systems
Complex systems in the context of water permeation involve numerous interacting components that can produce emergent behaviors not predictable from the sum of their parts. To illustrate, when considering soil as a permeation medium, factors such as particle size, soil composition, moisture content, and organic matter all influence how water is absorbed and transported. If one element changes, like an increase in salinity, it can alter the interactions between water molecules and the surrounding soil particles, leading to decreased permeability.
Additionally, the biological aspect adds another layer. The human body’s way of regulating water through the skin or kidneys exemplifies a complex interplay between various biological signs. External factors such as stress, hydration level, and temperature can all radically influence these permeation processes, making it a constant part of experimentation and research challenges. Without appropriate modeling techniques that can respect this complexity, any results derived can soon become questionable.
Future Directions in Research
Looking ahead, the future directions of permeation research are promising but will require innovative approaches to these challenges. Increased integration of multi-disciplinary methods can lead to breakthroughs. For example, combining insights from biology with advanced materials science can develop more effective filtration systems or biological membranes that mimic natural processes.
Moreover, advancing measurement techniques plays a critical role. Emerging technologies like nanotechnology and real-time imaging are paving ways to observe permeation dynamics at previously unattainable resolutions. By capturing molecular motions and interactions in action, researchers can gain insights into permeation mechanisms that traditional methods may miss.
In terms of application, the focus may need to shift towards sustainability and environmental resilience. Understanding how water permeates through different substrates not only helps in agriculture but also in conserving vital ecosystems. Future studies could prioritize these aspects, seeking solutions to pressing global issues like water scarcity and pollution.
In sum, overcoming the challenges and limitations in permeation research isn't merely about addressing gaps in knowledge but is a fundamental aspect of fostering deeper, more responsible applications of this understanding. As researchers face these dual fronts of complexity and limitation, their efforts will likely yield innovations that can significantly reshape how we interact with and utilize water in our world.
Case Studies and Real-World Examples
Case studies offer a unique lens through which to observe the complexities of water permeation in real-world scenarios. They often provide clarity to theoretical concepts, translating abstract principles into concrete instances that demonstrate their impact. By analyzing specific cases in different fields, we gain insights into both the beneficial and adverse effects of water movement through varied materials and ecosystems. Moreover, these examples underline the significance of interdisciplinary approaches; understanding how water interacts with biological systems, environmental settings, and technological advances is crucial for solving ongoing challenges in these areas.
In this section, we look at two vital case studies that illustrate the implications of water permeation: water filtration techniques and the effects of water quality on ecosystems.
Case Study: Water Filtration Techniques
The need for clean drinking water is paramount, and filtration tecnhiques have become crucial in achieving this goal. Various methods are employed to remove impurities like pathogens, sediments, and chemicals, enhancing the safety and palatability of water. For instance, reverse osmosis is a widely adopted technique in both industrial and residential settings. In this process, water is pushed through a semi-permeable membrane. The energy required to force the water through is significant but essential in ensuring contaminants are left behind.
Advantages of Reverse Osmosis:
- High Efficiency: It removes a large percentage of dissolved solids and contaminants.
- Space Saving: The compact design of many systems is ideal for homes.
- Versatility: It can be used in various settings, from municipal water supply to individual households.
However, not all filtration methods align with every situation. For example, in tropical regions, sand filtration is often more practical due to its low cost and simplicity. While it’s not as effective in removing certain contaminants, it is energy-efficient and sustainable, representing a balance between efficacy and practicality.
Ultimately, understanding the principles of water permeation allows engineers and scientists to design filtration systems tailored to the needs of specific environments, leading to improved health outcomes for communities.
Case Study: Impact of Water Quality on Ecosystems
The interrelation between water quality and ecosystem health is profound and sometimes alarmingly apparent. For instance, consider the case of the Chesapeake Bay in the United States. Here, nutrient pollution has had a cascading negative impact; excess nitrogen and phosphorus from agricultural runoff have fueled algal blooms. These blooms obstruct sunlight from penetrating the water, disrupting photosynthesis in aquatic plants.
As the algae die off, their decomposition consumes oxygen in the water, creating dead zones where aquatic life cannot thrive. This situation illustrates how water permeation principles can be applied to assess and mitigate human-induced environmental challenges.
The key elements to consider in analyzing such case studies include:
- Sources of Pollution: Understanding where pollutants originate helps in developing strategies for mitigation.
- Ecological Consequences: Identifying the ripple effects on various species can catalyze targeted conservation efforts.
- Policy Implications: Case studies like these often prompt legislative changes aimed at improving water quality, emphasizing the importance of integrating scientific insights into governing practices.
"Water quality isn't just a measurement; it encapsulates the health of entire ecosystems and the lifeblood of communities."
"Water quality isn't just a measurement; it encapsulates the health of entire ecosystems and the lifeblood of communities."
In summary, case studies, such as these, illuminate the critical role water permeation plays across various contexts. From ensuring safe drinking water supplies to maintaining ecosystem balance, understanding the dynamics at play is essential for fostering sustainable practices and protecting the valuable resource that is water.
Interdisciplinary Perspectives on Water Permeation
Water permeation is a dynamic aspect of various scientific fields, blending concepts from biology, chemistry, geology, and environmental science. Understanding how water interacts with different materials informs not just academic pursuits but also practical applications in everyday life. This section underscores the value of a multidisciplinary approach to water permeation, illustrating how insights gained from one discipline can illuminate challenges in others.
Integration with Other Scientific Disciplines
The integration of water permeation concepts across disciplines showcases the interconnectedness of scientific inquiry. For example, in biology, studying aquaporins allows researchers to unveil how cells manage water intake, crucial for survival and function. In environmental science, permeation processes through soil influence plant hydration and nutrient uptake, impacting agricultural productivity. Key areas of interaction include:
- Physical Chemistry: Examining the molecular structure of water and its behavior in different states and environments.
- Environmental Engineering: Developing water filtration systems that rely on principles of permeation to deliver clean drinking water.
- Medicinal Chemistry: Enhancing drug delivery methods by understanding the way water molecules facilitate transport through biological membranes.
By fostering collaboration between these disciplines, researchers can develop holistic strategies that address complex real-world issues related to water management, ecological balance, and human health. Each field brings unique perspectives and methods, enriching the overall understanding of water's behavior.
Societal Relevance and Implications
The implications of water permeation extend beyond the laboratory forming a fundamental bedrock for societal advancement. Water is life, and its management has profound consequences for public health, agricultural practices, and ecological sustainability. Relevant considerations include:
- Public Health: Understanding how contaminants permeate through water sources is crucial for crafting regulations to safeguard drinking water quality, indicative of both scientific rigor and social responsibility.
- Sustainable Agriculture: Insights into water movement can optimize irrigation practices, thus conserving water while ensuring crops are adequately nourished. This not only supports food security but also minimizes waste in water resources.
- Ecosystem Management: The study of permeation aids in understanding how water quality affects biodiversity. This knowledge is vital for guiding conservation efforts and rehabilitation in degraded ecosystems.
"An interdisciplinary perspective on water permeation is not just advisable; it is essential for sustainable development and the continued well-being of human and ecological systems."
"An interdisciplinary perspective on water permeation is not just advisable; it is essential for sustainable development and the continued well-being of human and ecological systems."
By drawing connections between various domains, society can leverage these insights to address pressing challenges, ensuring a more sustainable future. The ongoing exploration of water permeation encapsulates not merely scientific discovery but also a commitment to societal welfare.