Whole Genome Shotgun Sequencing Explained

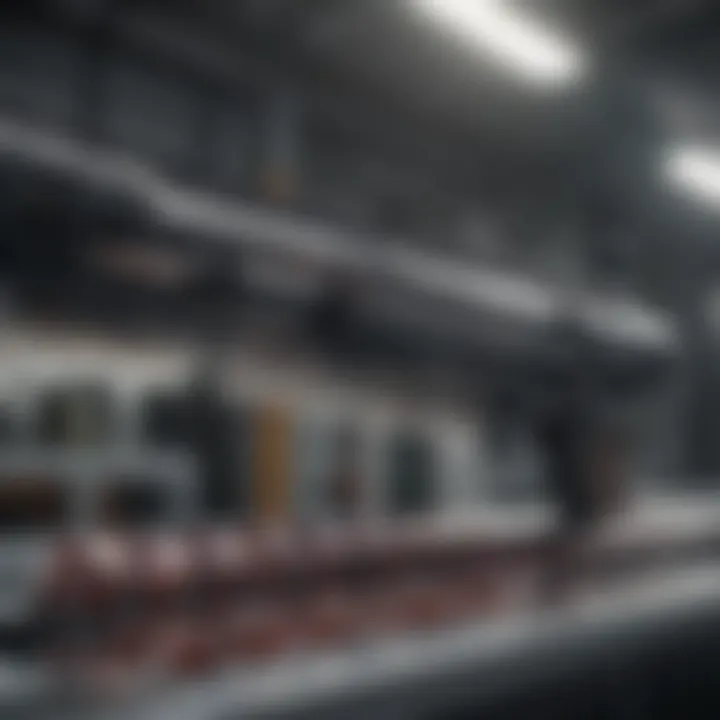
Intro
Whole genome shotgun sequencing (WGS) is a transformative method in the field of genomics. It allows researchers and scientists to sequence entire genomes quickly and efficiently. This technique has significantly advanced our ability to analyze genetic material. The methods of WGS have evolved over the years, driven by technological advancements and increasing need for comprehensive genomic data.
The concept of WGS revolves around the idea of randomly breaking up the genome into smaller fragments, sequencing those fragments, and then assembling them back together. This approach contrasts with older genomic sequencing methods, which often followed a more linear path. As researchers and organizations continue to harness the power of WGS, its applications span nearly all life forms, from microorganisms to complex eukaryotes.
In this article, we will delve into the fundamental aspects of WGS, its current advancements in research, and the challenges faced in data analysis. Understanding WGS is vital for professionals and researchers in genetics, bioinformatics, and other related fields. By exploring these components, we aim to provide a clear view of how WGS shapes modern genomics.
Key Concepts
Definition of the Main Idea
Whole genome shotgun sequencing is a powerful method that simplifies the process of sequencing DNA. The primary goal of WGS is to generate a complete representation of an organism's genetic material. This sequencing technique can be applied to various organisms and is often used in medical research, evolutionary biology, and biodiversity studies.
Overview of Scientific Principles
The foundational principle of WGS involves fragmenting the genome into smaller pieces, usually several hundred base pairs long. These fragments are then sequenced using high-throughput sequencing technologies. Modern sequencing machines, such as the Illumina sequencing platform, can produce millions of sequences in a single run. After sequencing, sophisticated software is utilized to align and assemble the fragments back into their original order, forming a complete genome.
Some key steps in this process include:
- Fragmentation: The genome is randomly sheared into smaller segments.
- Library Preparation: Specific adapters are added to the ends of the fragments, allowing them to be amplified and sequenced.
- Sequencing: The fragments are fed into the sequencer, where they are read and converted into data.
- Assembly: Computational tools reconstruct the original genome by aligning overlapping sequences from the fragments.
"Whole genome shotgun sequencing revolutionizes genomic research, making it faster and more efficient than traditional methods."
"Whole genome shotgun sequencing revolutionizes genomic research, making it faster and more efficient than traditional methods."
Current Research Trends
Recent Studies and Findings
WGS is continuously evolving, with numerous studies showcasing its versatility and efficacy. Recent research highlights the importance of WGS in personalized medicine, where individual genomic information can guide treatment plans tailored to the patient's genetic profile. Studies also explore the use of WGS in tracking outbreaks of infectious diseases, identifying resistance mutations in pathogens, and understanding genetic diversity in populations.
Significant Breakthroughs in the Field
Noteworthy breakthroughs have emerged in WGS technology. Improvements in sequencing accuracy, reduced costs, and faster data processing have opened new avenues for research. One significant development is the introduction of long-read sequencing technologies, such as those from Pacific Biosciences and Oxford Nanopore. These technologies allow sequences to be read over longer stretches of DNA, improving assembly and characterization of complex regions of the genome.
As these advancements continue, WGS will likely play a crucial role in future genomic studies. The insights gained from comprehensive genomic data can lead to new discoveries in medicine, evolutionary biology, and conservation efforts.
Prologue to Whole Genome Shotgun Sequencing
Whole Genome Shotgun Sequencing (WGS) has emerged as a fundamental method in genomics, enabling researchers to decode entire genomes with remarkable speed and efficiency. Its significance cannot be overstated. In an era where understanding genetic information is crucial for advancements in medicine, agriculture, and evolutionary biology, WGS serves as a cornerstone technology. This approach facilitates an extensive exploration of genetic variations, offering insights that have transformative implications for various fields.
The importance of WGS lies in its ability to provide a comprehensive view of an organism's genetic makeup. Traditional sequencing methods often fall short in terms of throughput and detail. In contrast, WGS allows for the capture of vast amounts of data in a relatively short time, significantly reducing the costs associated with genomic studies. Such efficiency is vital for projects aiming to sequence multiple genomes, paving the way for large-scale studies in diversity, mutation rates, and evolutionary patterns.
However, the introduction of WGS is not without its own set of challenges. Data interpretation remains a complex task, requiring advanced bioinformatics tools and expertise. This means that while WGS offers incredible potential, it also demands a robust infrastructure for data processing and analysis.
In summary, WGS is an important topic to understand in contemporary genomics. It encompasses various techniques that contribute to its efficacy and broad application, and it raises points worth considering regarding its implications and challenges.
Defining Whole Genome Shotgun Sequencing
Whole Genome Shotgun Sequencing is a method for sequencing an entire genome. It fragments the DNA into smaller pieces, selects a representative sample of these fragments, and sequences them. Each fragment's sequence is then assembled into a complete genome using computational algorithms.
This approach differs from traditional sequencing methods, which typically sequence regions of the genome sequentially. By obtaining multiple overlapping sequences at once, WGS efficiently utilizes next-generation sequencing technologies. This method is particularly useful for complex genomes, such as those of eukaryotes, where the genome shows significant size and structural diversity.
One of the primary strengths of WGS is its capacity for high-throughput sequencing. This enables it to produce millions of reads in a single experiment, providing a comprehensive picture of an organism’s genetic content in one go. It is this feature that has made it a preferred choice in many modern genomic studies.
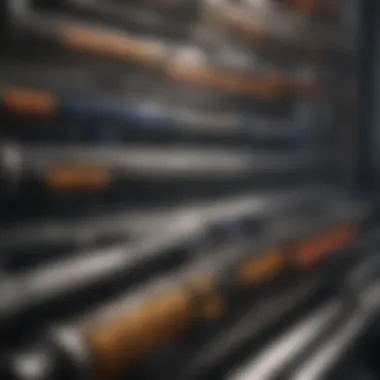
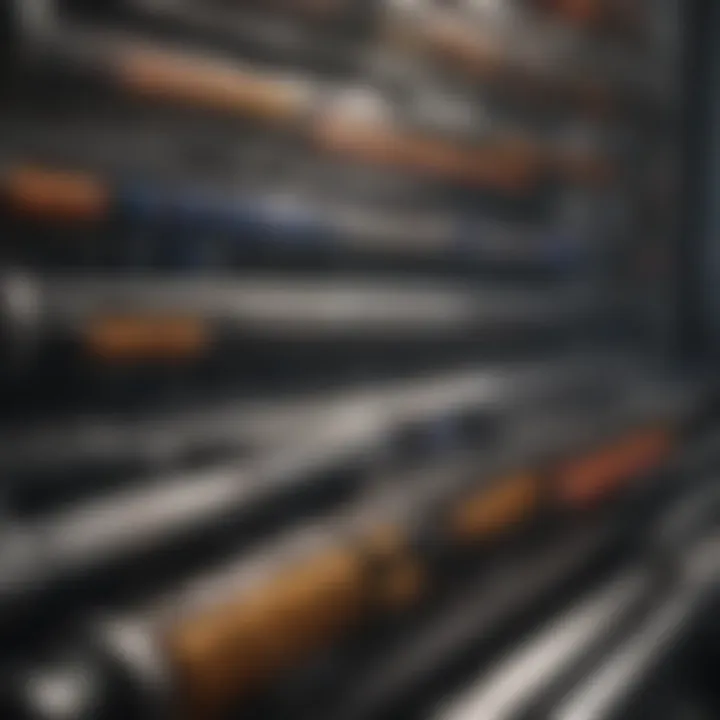
Historical Context and Development
To appreciate the importance of Whole Genome Shotgun Sequencing, it is vital to understand its historical context. The evolution of sequencing technologies has been marked by various milestones.
The early days of DNA sequencing were dominated by the Sanger method, which, although revolutionary, had limitations in speed and scalability. As the Human Genome Project progressed in the late 20th century, the need for faster methods became increasingly apparent. The advent of next-generation sequencing technologies in the 2000s provided the solution.
WGS entered the spotlight when researchers made substantial advancements in cloning strategies and sequencing technologies. The technique matured through iterative developments, such as improvements in fragment library construction and computational assembly algorithms, paving the way for its widespread adoption in genomic studies today.
In recent years, WGS has also seen integration with other technologies, allowing for greater insights into genetic variants and their functional implications. This historical evolution underscores the importance of WGS in the field of genomics and its role as a critical technology in the future.
Fundamental Principles of WGS
Understanding the Fundamental Principles of Whole Genome Shotgun Sequencing (WGS) is crucial for grasping how this technology advances genomic sciences. WGS allows researchers to decode entire genomes rapidly and efficiently. It brings several benefits, including decreased sequencing times and lower costs compared to traditional methods. The principles of WGS are pivotal in shaping the future of genomics, influencing areas such as personalized medicine, evolutionary studies, and microbiome research. A solid grasp of these principles ensures that scientists and practitioners can effectively utilize the technology in various applications.
Fragmentation of Genomic DNA
The process begins with the fragmentation of genomic DNA. This step involves breaking down the long strands of DNA into smaller, more manageable pieces. The fragmentation can be achieved using several methods, including physical shearing, enzymatic digestion, or sonication. Each approach has its benefits and challenges. When DNA is fragmented, it creates a library of small DNA pieces that can be cloned and sequenced separately. This process is essential as it allows the entire genomic sequence to be captured without the need for precise starting templates. The quality of fragmentation is critical; over-fragmentation can lead to gaps in the data, while under-fragmentation can complicate the assembly process.
Cloning and Sequencing of DNA Fragments
Once fragmentation is complete, the next step is cloning and sequencing of DNA fragments. The fragments are inserted into cloning vectors, which transport them into host cells, typically bacteria. This cloning step allows for the amplification of each fragment, ensuring that there is sufficient material for sequencing. After cloning, the fragments are sequenced using high-throughput sequencing technologies. This stage is vital because it generates data that represents the composition of the original genomic DNA. Different sequencing technologies, such as Illumina or Pacific Biosciences, can be used, each with unique benefits. For instance, Illumina is known for high throughput and accuracy, while Pacific Biosciences offers longer reads, which can facilitate assembly.
Assembly of the Sequenced Fragments
The final principle in WGS is the assembly of the sequenced fragments. This involves compiling the sequenced data back into a complete genome. Advanced bioinformatics algorithms are employed to align the overlapping regions of the individual sequences, allowing researchers to reconstruct the original genome. Assembly can be straightforward in organisms with less complex genomes, but can be more challenging in complex genomes due to repetitive sequences and structural variations. Achieving a high-quality assembly is crucial for subsequent data analysis and interpretation. The accuracy of this assembly can significantly impact results, particularly in studies related to genetic disorders or evolutionary biology.
"Whole Genome Shotgun Sequencing revolutionized how we approach genomic studies, merging efficiency with comprehensive coverage of genetic information."
"Whole Genome Shotgun Sequencing revolutionized how we approach genomic studies, merging efficiency with comprehensive coverage of genetic information."
In summary, comprehending the fundamental principles of WGS lays a foundation for understanding its applications and implications in modern science. From fragmentation to assembly, each step plays a vital role in the efficacy of genome analysis.
Technological Advancements in WGS
Technological advancements in Whole Genome Shotgun Sequencing (WGS) have dramatically transformed the landscape of genomics. These innovations have not only accelerated the process of sequencing but have also enhanced the accuracy and breadth of genomic data obtained. Understanding these advancements is crucial for comprehending their implications across various fields, from clinical genomics to microbial studies.
Next-Generation Sequencing Technologies
Next-Generation Sequencing (NGS) technologies represent a significant leap from earlier methods. This shift allows for the parallel sequencing of millions of fragments simultaneously, drastically reducing the time and cost associated with genome sequencing. NGS techniques utilize massively parallel sequencing platforms, such as Illumina, Ion Torrent, and Pacific Biosciences, among others. Each of these platforms has its strengths, targeting specific needs within genomic research.
The key benefits of NGS include:
- High-throughput capabilities: This allows rapid sequencing of entire genomes within days, making it feasible for large-scale projects.
- Cost-effectiveness: The reduction in costs facilitates broader access to genomic data.
- Improved accuracy: Enhanced error correction methods ensure more reliable results.
In comparison to the former Sanger sequencing, NGS offers a dramatic increase in efficiency and scale. However, it also introduces complexities in data management and requires sophisticated bioinformatics tools to process the data generated.
"Next-Generation Sequencing has revolutionized the way researchers approach genomic studies, making it feasible to sequence complex genomes quickly and affordably."
"Next-Generation Sequencing has revolutionized the way researchers approach genomic studies, making it feasible to sequence complex genomes quickly and affordably."
Comparison with Traditional Sequencing Methods
Traditional sequencing methods, like Sanger sequencing, used to dominate the field. Sanger sequencing is known for its long-read capabilities and high accuracy for small-scale sequencing projects. However, it is limited in throughput and is considerably slower and more expensive on a large scale.
In contrast to Sanger sequencing, WGS through NGS provides:
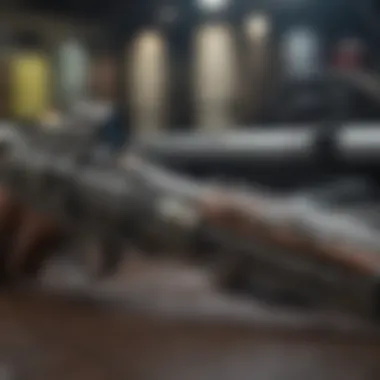
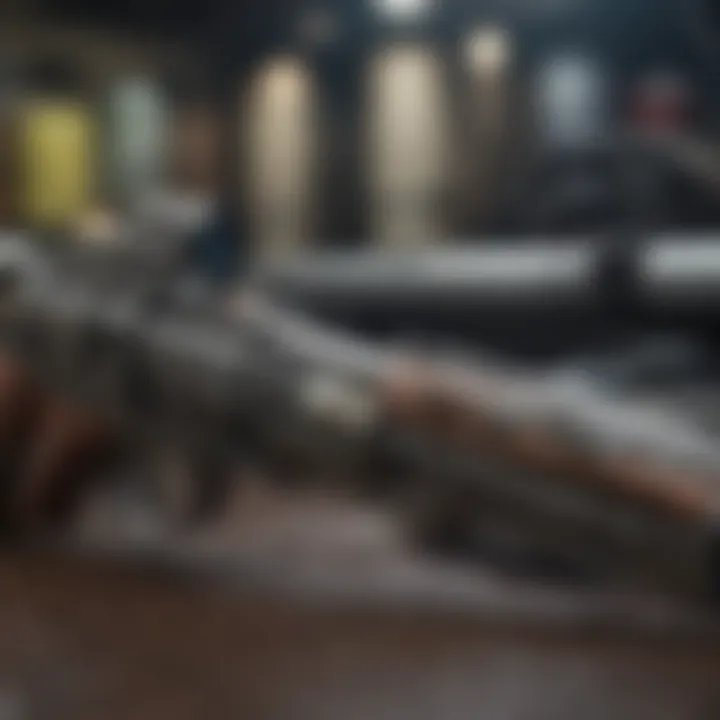
- Higher throughput: NGS can sequence entire genomes at once, while Sanger requires sequencing fragment by fragment.
- Lower cost per base: The cost-effectiveness of NGS makes large-scale projects feasible.
- Scalability: WGS can be adjusted for varying scales of projects, from small microbial genomes to entire human genomes.
Thus, while traditional Sanger sequencing remains beneficial for some applications, it’s clear that technological advancements in WGS represent a paradigm shift in genomics, enabling a more comprehensive exploration of genetic information and its vast implications for research and medicine.
Applications of Whole Genome Shotgun Sequencing
Whole genome shotgun sequencing (WGS) seeks to bridge gaps in genomic understanding across various fields. Its versatility allows for extensive applications, making it a fundamental tool in modern genomics. This section delves into three prominent areas where WGS plays a pivotal role: clinical genomics and personalized medicine, evolutionary biology studies, and microbial genome sequencing.
Clinical Genomics and Personalized Medicine
In clinical settings, whole genome shotgun sequencing enables the identification of genetic variants that contribute to diseases. Personalized medicine tailors medical treatment to an individual’s genetic profile. By sequencing patient genomes, healthcare providers can determine predispositions to various conditions. For example, identifying mutations in the BRCA1 and BRCA2 genes helps assess breast and ovarian cancer risk.
Furthermore, WGS contributes to pharmacogenomics, the study of how genes affect a person's response to drugs. This knowledge can guide drug selection, dosing, and strategies to avoid adverse effects. Consequently, the alignment of treatment with genetic information enhances the effectiveness of therapies.
Evolutionary Biology Studies
Whole genome shotgun sequencing plays a significant role in evolutionary biology. It allows researchers to examine the genetic makeup of species, providing insights into their evolutionary relationships. By comparing genomes, scientists can trace lineages and identify common ancestors, contributing to our understanding of evolution.
In addition, WGS facilitates studies of adaptation and speciation. It aids in uncovering genetic changes associated with environmental adaptations, thus revealing how species evolve in response to ecological pressures.
Microbial Genome Sequencing
WGS is also pivotal in microbial genome sequencing. This application supports efforts in microbial ecology, public health, and personalized treatments for infections. Sequencing microbial genomes helps identify pathogens quickly, which is crucial for effective treatment. For example, during outbreaks, rapid sequencing can track transmission routes and inform containment strategies.
Moreover, insights gained from microbial genomes contribute to our understanding of microbial diversity. By comparing genomes across different environments, researchers can explore the roles of microbes in ecosystems. The knowledge from these studies informs biotechnological applications, such as drug development and environmental management.
Overall, the applications of whole genome shotgun sequencing highlight its transformative impact across disciplines. As technology evolves, so will its applications, further enriching our understanding of biology.
Data Analysis in WGS
Data analysis is a cornerstone of whole genome shotgun sequencing (WGS). The intricate process of generating vast amounts of genetic data necessitates robust analytical approaches. As sequencing technology advances, the scale and complexity of data increase substantially. Thus, efficient analysis is critical for deriving meaningful insights from genomic data. Researchers and practitioners must employ bioinformatics tools to navigate this landscape, addressing challenges while maximizing the utility of the information generated.
Bioinformatics Tools and Software
Bioinformatics encompasses a variety of software and algorithms designed to facilitate the analysis of genomic data. Key tools include:
- Bowtie: A fast and efficient alignment tool for short reads.
- GATK (Genome Analysis Toolkit): Essential for variant discovery and genotyping.
- SPAdes: Used for assembling genomes from short reads.
- Quantitative Insights Into Microbial Ecology (QIIME): Focused on analyzing microbial community data.
Using these tools, researchers can perform tasks such as read alignment, variant calling, and genome assembly. Each software has its strengths and limitations, thus understanding their capabilities is crucial for accurate data interpretation.
Challenges in Data Interpretation
Data interpretation in WGS is marked by several challenges. One primary issue is the high level of noise present in sequencing data. Artifacts can arise from sequencing errors, sample contamination, or biases in the sequencing process. Furthermore, distinguishing between true genetic variants and incidental findings complicates analysis. Researchers often grapple with:
- Data Quality: Ensuring that the data is clean and reliable is crucial.
- Variant Interpretation: Analyzing the functional significance of identified variants requires expertise and careful consideration.
- Integration of Multi-Omics Data: Merging genomic data with transcriptomic, proteomic, or metabolomic data adds layers of complexity.
Addressing these challenges mandates a rigorous understanding of both the biological context and the analytical frameworks employed.
Handling Large Datasets
With the advent of high-throughput sequencing technologies, handling large datasets has become a fundamental task in WGS. As the volume of data continues to grow exponentially, researchers face specific issues:
- Storage Solutions: Efficient data storage is necessary to accommodate the massive files generated. Solutions include cloud storage and high-capacity data servers.
- Data Processing: The need for powerful computational resources to analyze large datasets cannot be overstated. Utilizing cluster computing or high-performance computing resources is often required.
- Scalability of Analysis Pipelines: Analytical workflows must be adaptable to scale with rising data volumes and complexities.
Ethical Considerations in WGS
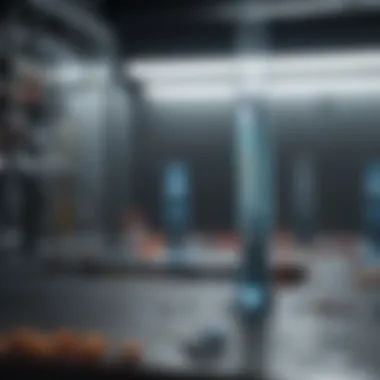
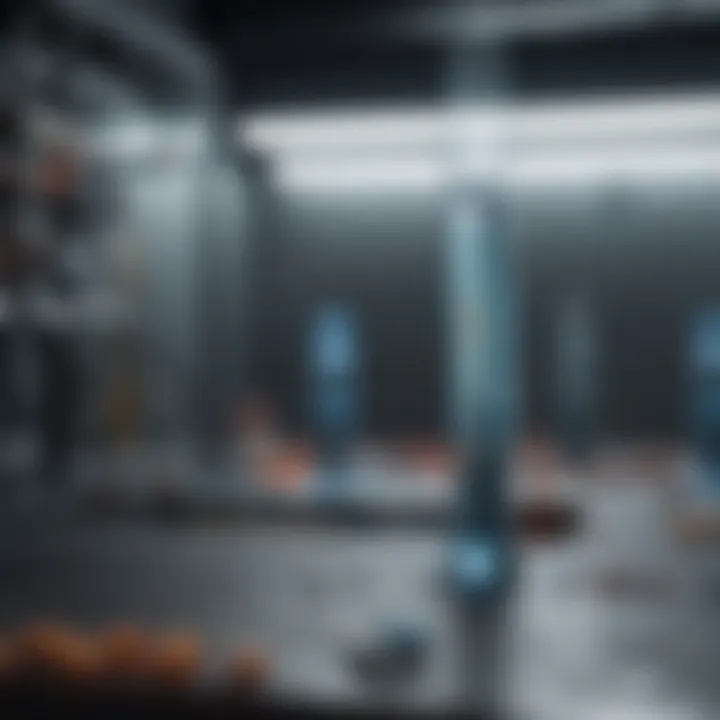
Ethical considerations in Whole Genome Shotgun Sequencing (WGS) are crucial for ensuring responsible use of genomic data. With rapid advances in sequencing technologies, the implications affect not just individual privacy but also societal norms and behaviors. This section delves into key aspects that warrant careful attention as they shape the future landscape of genomic research and applications.
Privacy and Data Security
Privacy in WGS revolves around how genetic information is collected, stored, and shared. The genomic data derived from WGS holds sensitive information about an individual’s health, ancestry, and potential predispositions to diseases. Therefore, maintaining data security is paramount.
Some of the primary concerns include:
- Unauthorized Access: Genetic data can be a target for hackers, making secure storage systems essential.
- Informed Consent: Participants must be fully educated on how their genetic data will be used and who may have access to it.
- Data Anonymization: While researchers may attempt to anonymize genetic information, complete anonymity is hard to achieve. There remains the risk of re-identification through advances in computational technologies.
- Long-term Implications: Genetic data could be stored indefinitely. Future uses of this data must adhere to ethical standards at the time of its collection.
The protection of genomic data is not only a technical challenge but also an ethical obligation to ensure trust and participation in research.
The protection of genomic data is not only a technical challenge but also an ethical obligation to ensure trust and participation in research.
Implications for Genetic Discrimination
Genetic discrimination refers to the unfair treatment of individuals based on their genetic information. As WGS becomes more prominent, the potential for misuse of this data is a growing concern. Various aspects of genetic discrimination include:
- Employment Opportunities: Employers may access genetic information to make decisions about hiring or promotions, potentially leading to biased outcomes.
- Insurance Coverage: Insurers might use WGS data to set premiums or deny coverage based on genetic risks.
- Social Stigma: Individuals with certain genetic traits may face stigma or ostracism from their communities if such information becomes public.
Addressing these issues necessitates robust policies and legislation to guard against discrimination based on genetic information. Awareness campaigns should also educate the public on the ethical application of genomic data, emphasizing that genetic information should not dictate a person's worth or potential.
Future Directions in Whole Genome Shotgun Sequencing
The future of Whole Genome Shotgun Sequencing (WGS) presents exciting opportunities and challenges that can profoundly impact genomics. As technology progresses, understanding these trends is vital for researchers and professionals. This section explores innovations on the horizon and the potential for integration with other omics technologies. Addressing these elements is crucial as the scientific community seeks to enhance the speed, accuracy, and applications of genomic sequencing.
Innovations on the Horizon
A critical aspect of future developments in WGS is the continual evolution of sequencing technologies. These innovations promise to increase throughput and reduce costs, making genomic analyses more accessible. Technologies such as single-molecule sequencing and advances in nanopore sequencing are at the forefront of this evolution. They allow for longer read lengths, which reduce the complexity of genome assembly.
Moreover, improvements in bioinformatics tools will play a substantial role in the future of WGS. Enhanced algorithms and software are needed to manage the growing amounts of data generated. Machine learning and artificial intelligence are increasingly becoming pivotal in interpreting complex genomic data. They can aid in identifying patterns that humans might overlook, leading to more accurate results in genomic studies.
"The integration of AI technologies in genomic analysis is not just beneficial; it is essential for managing the future demands of data interpretation."
"The integration of AI technologies in genomic analysis is not just beneficial; it is essential for managing the future demands of data interpretation."
Integration with Other Omics Technologies
The future of WGS also involves a greater integration with other omics technologies, such as proteomics, metabolomics, and transcriptomics. This multi-omics approach enables a comprehensive understanding of biological systems. By combining data from various omics, researchers can achieve a more holistic view of how genes influence phenotypes and metabolic processes.
The merging of omics technologies can facilitate breakthroughs in personalized medicine. For instance, by integrating genomic data with proteomic information, practitioners can identify how genetic variations influence protein expression. This information can lead to more tailored treatments for patients based on their specific biological profiles.
In addition, this interdisciplinary approach fosters collaboration among researchers from different fields. Sharing insights and methodologies from diverse areas enhances the overall capacity to address complex biological questions.
In summary, the future of Whole Genome Shotgun Sequencing appears bright, with numerous advancements anticipated in both technology and integration strategies. Staying abreast of these developments will be crucial for all stakeholders in the field of genomics.
Ending
The conclusion serves as a crucial component of this article, summarizing the key insights into Whole Genome Shotgun Sequencing (WGS) while emphasizing its significance in genomics. A comprehensive understanding of WGS is essential due to its implications in both research and clinical settings.
In the Summary of Key Points, we revisit the fundamental principles of WGS, which include its methodology of fragmenting genomic DNA, the advanced cloning processes, and the sophisticated data assembly techniques. These elements must be appreciated, as they showcase the evolution of sequencing technologies and their practical applications across various scientific disciplines.
Final Thoughts on WGS highlight the ongoing advancements in this field. As technologies progress, WGS is likely to become more accessible, enhancing its applications in personalized medicine and evolutionary biology. The potential for integrating WGS with other omics technologies presents exciting avenues for future research.
The impact of WGS on our understanding of genetic information is profound; it not only aids in disease diagnosis but also facilitates breakthroughs in precision medicine.
The impact of WGS on our understanding of genetic information is profound; it not only aids in disease diagnosis but also facilitates breakthroughs in precision medicine.
As we continue to explore the frontiers of sequencing, ongoing challenges in data analysis remain relevant. Efforts to enhance data interpretation, security, and ethical considerations will be essential for the future of genomics. Better tools and methodologies will ensure that we maximize the benefits of WGS while addressing the potential concerns.
Understanding these dynamics will prepare researchers and professionals in the field to adapt to the rapidly changing landscape of genomic technologies.